Agent
Alcohol
Phenol
Concentration
100%
3–12%
Diluent
None
Glycerin
Patient position
Lateral (bedside upwards)
Lateral (bedside down)
Added tilt
Semi-prone
Semi-supine
Painful side
Uppermost
Lowermost
Injection causes
Immediate burn
Painless warmth
Neurolysis occurs at:
Immediate
Delayed (15 min)
CSF uptake ends at:
30 min
15 min
Full effect occurs at:
3–5 days
1 day
Wallerian Degeneration
Named for Dr. Augustus Volney Waller (1816–1870), this is the major pathophysiologic mechanism underlying chemical neurolysis. It is a process that results when part of the axon, which has separated from a neuron’s cell body, degenerates distal to the injury. This is also known as anterograde or orthograde degeneration. Between the central nervous system (CNS) and the peripheral nervous system (PNS) , there are vast differences that characterize Wallerian Degeneration. The process will typically occur within 24 h from initial injury, whereby the axon will degrade stepwise; however, as noted above, the effects of alcohol and phenol subarachnoid neurolysis will typically demonstrate marked physiological changes at a much earlier time than 24 h.
In the PNS, after application of a chemical neurolytic drug (alcohol or phenol), it is the macrophages that will initiate degradation by removing myelin and axonal debris. After 96 h of initial injury , the basal lamina houses those remaining Schwann cells , which provide nerve growth factors for axonal sprouts to form. From the sprouts, new neuronal tissue will grow through the tube and will continue to advance to its target tissue. A regenerative process occurs over a period of multiple weeks to several months in certain situations under favorable physiological conditions (absence of acidosis) [1].
By comparison, the CNS does not contain Schwann cells, but oligodendrocytes that produce a myelin sheath. The macrophage removal of debris occurs over weeks, instead of days. This is due to inhibitor factors that hinder the progression of debris removal. For the regenerative process, it is not the myelin producing oligodendrocyte that provides the network lattice for the axon; rather, it is the astrocytes. Glial filaments are produced and form a glial scar, which fills the void created by the degraded axon and myelin from the initial injury. As a result, the normal axonal pathway is permanently disrupted and new neuronal tissue does not replace that scar [1].
Alcohol
One of the two primary agents used for neurolytic procedures, alcohol, exerts its mechanism of action through dehydration. As a result, phospholipids, cerebrosides, and cholesterol are extracted from neuronal cells. Additionally, mucoproteins are precipitated, and sclerosis of the nerve fiber and myelin sheath itself occurs. The primary mode of injury is through Wallerian degeneration . Alcohol will spare the Schwann cell tubes, leaving the possibility for neuron regeneration, the exception being the dorsal root ganglion. Indirectly, it acts on modulating pain perception via production of arterial vasospasm in a concentration-dependent manner [1].
One will observe inflammatory changes in the nerve roots, Lissauer’s tract, posterior columns, and meninges with subarachnoid alcohol chemical neurolysis. Individuals treated with ethanol will often complain of intense burning dysesthesias secondary to chemical neuritis when receiving the injection, or just afterwards. Another commonly encountered, potentially concerning complication is the potential for toxicity. One significant issue with alcohol is that toxicity is not dose dependent; individuals can develop toxicity even with standard dosages are injected intravascularly [1].
Phenol
The other widely used compound for neurolysis is phenol (carbolic acid). A unique added benefit to neurolysis with phenol is its local anesthetic properties. This affords additional analgesia for individuals receiving the injection because it is more tolerable. The effects include neurolysis via a different mechanism of action, in addition to Wallerian degeneration. Local anesthesia is achieved at concentrations of <1%, with no neurolysis. To attain maximal neurolysis, one would have to inject 12% phenol, which is the highest concentration that can be placed into solution. As a result, phenol has a biphasic action, wherein warmth and numbness occur initially from the local anesthetic properties, and nonselective degeneration with neurolysis follows shortly thereafter [1].
There is a direct correlation between concentration and neurolysis, unlike what is observed with alcohol chemical neurolysis, which is essentially complete at concentrations above 33% [7]. At concentrations <5%, primarily sensory block of A-delta and C-fibers occurs, and concentrations >5% beget both motor and sensory blockade. Protein degeneration is another outcome of using concentrations >5%, but coagulation of proteins occurs specifically at concentrations between 5 and 6%. More importantly, orthograde Wallerian degeneration ensues at the same concentration as protein coagulation. A-delta and C-fibers (cold, pressure, and nociceptive receptors) and A-beta fibers (stretch receptors) are destroyed, but the dorsal root ganglia are spared with phenol at these higher concentrations [1].
Unlike its counterpart alcohol , phenol damages the neural tube because it is directly neurotoxic , so nonspecific regeneration of the axon takes place. The greatest afforded benefit of phenol is that there is predictable toxicity and adverse outcomes, which are concentration dependent. With concentrations >6%, there are predictable neural adverse events, including the potential for spinal cord infarcts, development of adhesive arachnoiditis, and meningitis when the chemical is used intrathecally. The most concerning toxicities occurring after intravascular injection include central nervous system depression and cardiovascular collapse. Other organ systems may be affected, which include development of hepatic toxicity, and chronic poisoning, which can cause skin eruptions, gastrointestinal, and renal toxicity. In general, clinical applications are the same as for alcohol, but also include use of phenol for the splanchnic nerve, peripheral nerve roots, as well as for sympathetic block of the celiac ganglion and lumbar sympathetic chain.
Selected Techniques
Applications are vast, which include performing neurolytic blocks at the head and neck, subarachnoid, epidural, transforaminal, celiac plexus, lumbar sympathetic, SI joint, ganglion impar, and superior hypogastric plexus nerves as targets of nociception. With subarachnoid, and to a lesser extent epidural block, there is predictable segmental sensory loss for precise targeting of dorsal roots, while avoiding ventral root motor deficits. This chapter will focus on the technical aspects of nerve blocks with chronic pain treatment for the rehabilitation patient. When performing a neurolytic block at any site, imaging (ultrasound, fluoroscopy, CT scan , MRI ) is mandatory for both medical–legal reasons, to assure appropriate technique and procedure-related approach , as well as to assure competent assessment and negotiation of the relevant anatomy.
Head and Neck Blocks
The trigeminal nerve and Gasserian ganglion blocks are two different techniques used in targeted neurolysis for chronic facial pain conditions. Interventionists have at their disposal fluoroscopic, ultrasound, and CT-guided access to the foramen ovale to execute the block. CT has advantages in offering views in rostral, sagittal, and axial sections. This is not to say fluoroscopy cannot be effectively used, as high success rates are observed when utilized; however, it is at the discretion of the individual performing the block as to how to best carry it out. Pain secondary to cancer, which can occur in the V1, V2, and V3 trigeminal nerve distributions, can be treated in this manner (see Figs. 39.1, 39.2, 39.3, 39.4, and 39.5 below).
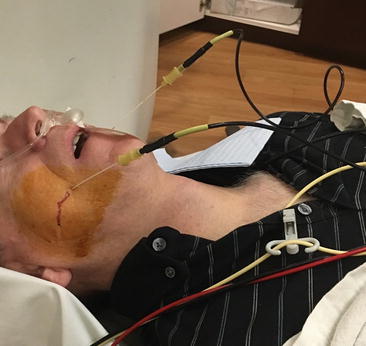
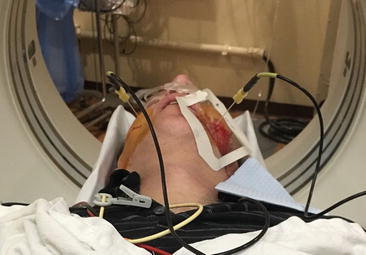
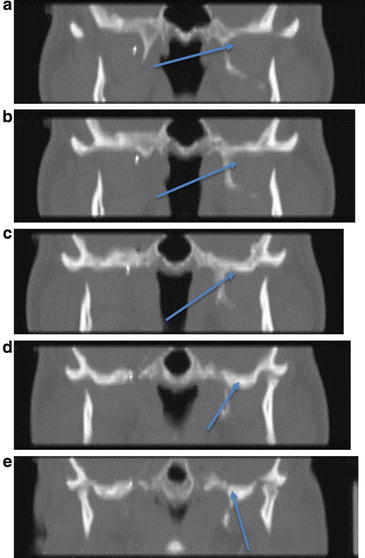
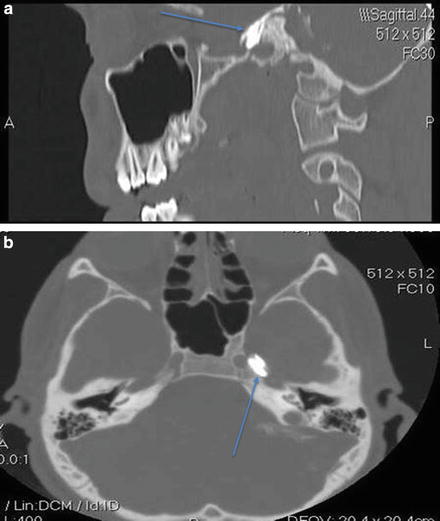
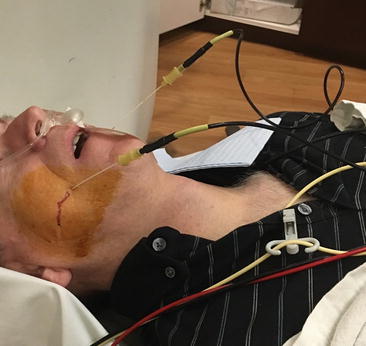
Fig. 39.1
Patient positioned for neurolytic (radiofrequency thermocoagulation) Gasserian ganglion block for chronic intractable facial pain due to idiopathic trigeminal neuralgia. Bilateral subzygomatic 15 cm cannulas have been placed into the foramen ovale under CT scan guidance in a lightly sedated patient who provides verbal feedback (Photo courtesy of Kenneth D. Candido, M.D.)
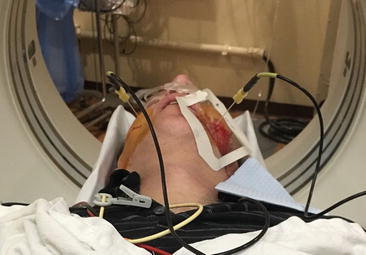
Fig. 39.2
Caudal-cranial view of bilateral subzygomatic 15 cm cannulas placed into the foramen ovale using CT scan guidance. Radiofrequency (RFA) electrodes have been placed through the cannulas and continuous RF energy is being applied at 80°C for 90 s for thermocoagulation of the Gasserian ganglion (Photo courtesy of Kenneth D. Candido, M.D.)
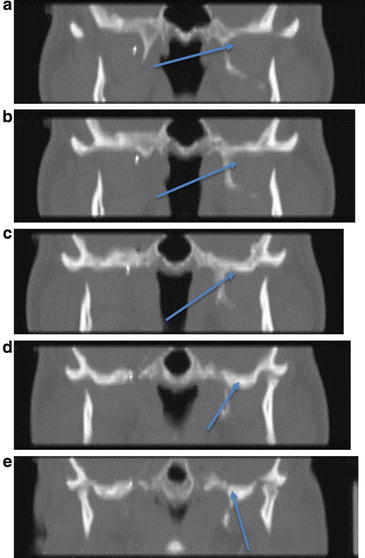
Fig. 39.3
(a–e) CT scan images of needle entering the foramen ovale : rostral views; arrows indicate needle tip (Photos courtesy of Kenneth D. Candido, M.D.)
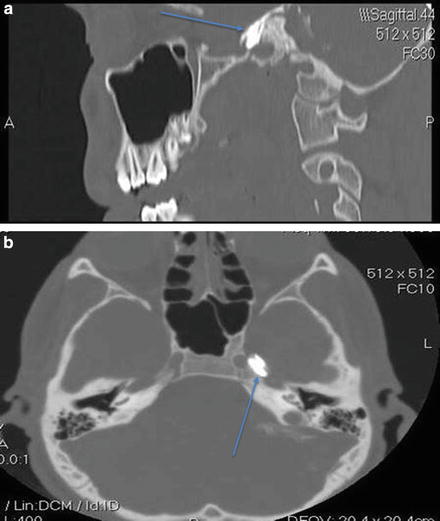
Fig. 39.4
(a) Sagittal view of contrast injection into foramen ovale for TGN block (Photo courtesy of Kenneth D. Candido, M.D.). (b) Axial view of contrast injection into foramen ovale for TGN block (Photo courtesy of Kenneth D. Candido, M.D.)