Chapter 5
Neuroanatomy of the nociceptive system
At the end of this chapter readers will:
1 Appreciate the different types of peripheral nociceptors and their associated axons.
2 Understand the organization of the dorsal horn and the termination patterns of afferent inputs.
3 Understand the pathways involved in transmitting nociceptive information within the nervous system.
4 Understand the areas of the nervous system involved in the perception, integration and response to nociception.
OVERVIEW
This chapter is concerned specifically with the nervous system structures involved in nociception. The historical framework for studying pain has implied that there is a sensory channel for pain in the manner of sensory channels for other sensations (Willis & Coggeshall 1991). It will be clear from the following review that this is not the case, and that pain is a complex, multidimensional phenomenon, with pain signals being transmitted to many different regions of the nervous system. Melzack and Casey (1968) suggested that pain needs to be considered in three interacting dimensions: sensory–discriminative, cognitive–evaluative and motivational–affective. The sensory dimension refers to the capacity to analyse the intensity, location, quality and behaviour of pain. The cognitive–evaluative dimension is concerned with the phenomena of anticipation, attention, suggestion and the influence of previous experience and knowledge. Finally, the motivational–affective dimension is the emotional response (fear, anxiety) that controls responses to the pain. A study of the anatomical connections of the nociceptive system provides a framework for understanding how all these dimensions of pain are registered and interact within the nervous system.
STRUCTURE AND FUNCTION OF PERIPHERAL NOCICEPTORS
Nociceptors are a class of peripheral receptors that respond to tissue-damaging or potentially tissue-damaging stimuli (from Latin nocere, to injure). The skin is densely innervated by nociceptors, which are present in other body tissues, including bone, muscle, joint capsules, viscera and blood vessels, as well as the meninges and peripheral nerve sheaths. Nociceptors have not been found in articular cartilage, synovial membranes, lung parenchyma, visceral pleura, pericardium, brain or spinal cord tissue. The simplest type of sensory receptor is called the free nerve ending, which terminates in a naked unmyelinated ending in cutaneous or other tissue. Historically, free nerve endings have been believed to subserve only pain, but this is not the case. Moreover, more recent studies of the ultrastructure of these receptors have indicated that their structure is more complex than this descriptive term implies. Its terminals remain ensheathed by Schwann cell processes until they penetrate the epidermal basal lamina (Kruger et al 1981).
The inference that specialized nociceptors existed was made on the basis of experiments on peripheral nerves in humans using graded electrical stimulation and differential nerve blocks (Adrian 1931; Bessou & Perl 1969; Burgess & Perl 1967). These experiments also indicated that pain was signalled by two sets of afferent fibres:
• small-diameter thinly myelinated fibres (Aδ) with a conduction velocity of 5–30 m/s – activation of these fibres is associated with well-localized sensations of sharp, pricking pain.
• small-diameter, unmyelinated C fibres that conduct slowly (0.5–2 m/s) – these fibres carry diffuse pain sensations that can be dull, poorly localized and persistent (Torebjörk & Ochoa 1980). A class of C fibres, called C-tactile afferents, are present only in hairy skin, and mediate pleasant touch (Löken et al 2009).
This terminology is used in relation to cutaneous and visceral axons (Erlanger & Gasser 1937). A different terminology applies in the case of muscle and joint nerves (Table 5.1).
Nociceptors
Nociceptors are complex structures that have heterogeneous properties, responding to multiple stimulus modalities (polymodal). Investigation of responses of specific nociceptors is fraught with difficulty, as a lack of response may indicate a failure to apply a sufficient intensity of stimulation. Moreover, application of the stimulus may induce long-term changes in the response properties of the nociceptor (Meyer et al 2006).
Type I Aδ nociceptors were initially called high-threshold mechanoreceptors (HTM; Burgess & Perl 1967) because they respond with a slowly adapting discharge to strong punctate pressure and were thought to be unresponsive to heat. However, they have been shown to have very high heat thresholds, usually 53°C or higher (Treede et al 1991). With maintained heat stimuli, HTM receptors will respond and become sensitized with tissue injury (Basbaum et al 2009). Meyer et al (1994) have suggested that the Aδ Type I receptors be termed A-fibre mechano-heat sensitive receptors (AMHs), as they are responsive to both mechanical and heat stimuli. Heat sensitivity in AMHs is mediated by the vanilloid receptor-like protein 1 (TRPV2) receptors (Caterina et al 1999). These units are densely distributed in hairy and glabrous skin (Campbell et al 1979). Their receptive fields are distinctive, consisting of a series of sensitive points that may be spread evenly over an area of several square centimetres in proximal areas. In distal areas, such as the glabrous skin of the hands and feet, or on the face, receptive fields are smaller and may comprise only a single point. These units have myelinated axons (Aδ) with a conducting speed of 5–25 m/s, with a few conducting more quickly in the A–β range (55 m/s). They mediate the ‘first’ response to pinprick and other intense mechanical stimuli (Basbaum et al 2009).
Type II Aδ nociceptors have a lower heat threshold than Type 1 units, but have very high mechanical thresholds, so they are termed heat-responsive MIAs. Mean conduction velocity is 15 m/s. These mediate the ‘first’ acute pain response to noxious heat. They have been reported in the knee joint (Schaible & Schmidt 1985), viscera (Häbler et al 1990) and cornea (Tanelian 1991). Some of the cutaneous MIAs may be chemospecific receptors, while others may respond to intense cold or heat stimuli (Meyer et al 1991). MIAs are not found in glabrous skin. These receptors may become sensitized to mechanical stimuli after injury.
The unmyelinated C fibres are heterogeneous. Most C fibres are polymodal, responding to both mechanical and heat stimuli, hence the term C-fibre mechano-heat sensitive receptor (CMH). Bessou and Perl (1969) have shown that these are the predominant type of C-fibre nociceptor in mammalian skin, comprising about 90% of all afferent C fibres.
The heat responsive terminals of CMHs lie at varying depths beneath the skin (between 20 and 570 μm) and so their heat threshold is dependent on the temperature at the heat responsive terminal and not on the rate of temperature increase (Tillman et al 1995). Mechanical nociceptors with C-axons have a slowly adapting response to mechanical stimuli. They lack the distinctive multipoint receptive fields of the A-fibre units; instead their fields usually consist of a small zone of uniform sensitivity (Iggo 1960; Lynn 1984).
C-tactile fibres are low-threshold mechanoreceptors found in hairy skin. They have small receptive fields (Wessberg et al 2003) and respond vigorously to slow and light stroking (Bessou et al 1971; Vallbo et al 1999). These fibres mediate pleasant touch sensation, i.e. the affective dimension of sensation.
The chemosensitivity of C-polymodal nociceptors has not been studied as much as their sensitivity to heat and pressure. They can be excited by potassium, histamine, serotonin, bradykinin, capsaicin, mustard oil, acetylcholine and dilute acids, by various means (topical application, intradermal injection, arterial injection) and all in doses that would be painful in humans (see Willis & Coggeshall 1991 for review). Chemicals act on nociceptors by altering the conductance of ion channels in the cell membrane and causing depolarization (Rang et al 1991). This may result in sensitization of the nociceptors, such that they have an increased responsiveness to stimulation. Further information on peripheral sensitization will be presented in Chapter 6.
Cold nociceptors, transmitting along C fibres, have been reported in monkeys (LaMotte & Thalhammer 1982) and in humans (Campero et al 1996). These respond strongly to prolonged cooling of the skin by ice and weakly to strong pressure, but are not responsive to heat. The threshold for cold pain is about 14°C. Because of the delay in response between the onset of the stimulus and report of pain, it has been suggested that cold pain is subserved by deeper receptors than heat pain. The sense of cooling is subserved by primary afferents called cold fibres, which are predominantly Aδ in type. However, cold fibres do not faithfully encode stimuli that induce cold pain (Meyer et al 2005). Cutaneous Aδ nociceptors have ongoing activity at room temperature. They also respond to temperatures below 0°C and encode stimulus intensity (Simone & Kajander 1997). Cold pain is thought to be mediated by nociceptors located in cutaneous veins (Klement & Arndt 1992).
Another way of classifying nociceptors is through identification of neuroanatomical and molecular characteristics (Snider & McMahon 1998). A peptidergic population releases neuropeptides such as substance P and calcitonin gene-related peptide (CGRP), as well as the TrkA neurotrophin receptor. A non-peptidergic population expresses the Ret tyrosine kinase receptor and is sensitive to glial-derived neurotrophic factor (GDNF). These two populations of nociceptors have different central projection patterns. The peptidergic population terminates in lamina I and the outer part of lamina II of the dorsal horn, while the non-peptidergic group terminates in the inner part of lamina II. The functional difference between the two populations is not yet clear (Snider & McMahon 1998).
Skeletal muscle nociceptors
The terminology of Lloyd (1943) is usually used in relation to muscle and joint nerves (Table 5.1). Group III afferent fibres are small-diameter myelinated fibres; group IV fibres are unmyelinated and constitute the majority of muscle nociceptors. Numerous unencapsulated endings can be located in the connective tissue and in the wall of arterioles in skeletal muscle, and can be subdivided into mechanical and polymodal types (Stacey 1969). Effective stimuli are high-intensity mechanical forces, as well as endogenous pain-producing substances such as bradykinin, serotonin and potassium ions. Hypoxia and impaired metabolism following trauma or unaccustomed exercise and increased levels of adrenaline may also activate nociceptors (Kieschke et al 1988; Mense 1993). Group III afferent fibres are responsive to mechanical stimulation of muscle, including stretch (Mense & Stahnke 1983), and many would be activated by exercise and therefore probably function as ergoreceptors. However, a significant proportion of these are nociceptive. Some group III afferents are responsive to the injection of chemicals such as hypertonic sodium chloride (Abrahams et al 1984). Approximately 40% of group IV afferents are classified as low-threshold mechanosensitive (LTM) units and respond to gentle muscle stretch or contraction (Hoheisel et al 2005). These non-nociceptive units control the adjustment of circulation and respiration to the demands of physical exercise (McCloskey & Mitchell 1972). The remaining proportion of group IV afferents are activated with tissue-threatening mechanical stimulation, e.g. when muscle contractions occur during ischaemia (Mense & Meyer 1985), and are called HTM units (Mense 2009). Many are readily activated by pain producing chemicals (Mense & Meyer 1988) or thermal stimuli (Hertel et al 1976).
One of the most relevant chemical causes of muscle pain is a drop in tissue pH (an increase in proton concentration), possibly related to tonic contractions leading to ischaemia or accumulation of lactic acid (Mense 2009). The mechanism is related to the large number of acid-sensing ion channels (ASICs) in the membrane of muscle nociceptors (Hoheisel et al 2004; Sluka et al 2003). Another cause is a release of adenosine triphosphate (ATP) resulting from damage to muscle cells or an increase in permeability of the muscle cell membrane (Burnstock 2007). Other chemical factors are bradykinin, formed in damaged tissue, and serotonin, prostaglandins and nerve growth factor, which have a sensitizing action of group IV afferents (see Mense 2009 for review).
Joint nociceptors
Nociceptors in joints are located in the joint capsule and ligaments, bone, periosteum, articular fat pads and around blood vessels, but not in the joint cartilage. They have been studied predominantly in the knee joint. The terminals of group III and group IV sensory nerve endings comprise multiple axonal beads ensheathed by Schwann cell processes except for some areas of exposed axon membrane containing structural specializations characteristic of receptive sites. The beads thus represent multiple receptive sites (Heppelmann et al 1990). Some of these receptors may be nociceptive.
Joint nociceptors can be classified as:
• high-threshold units that discharge only in response to noxious pressure or extreme joint movement (Bessou & Laporte 1961)
• units that respond to strong pressure but not to movement
• units that do not respond to any mechanical stimulus in the normal joint (MIAs or silent nociceptors) (Schaible & Schmidt 1988).
In the normal joint, only the first type are activated, but all joint afferents become sensitized if the joint becomes inflamed (see Chapter 6).
Visceral nociceptors
In somatic tissue such as skin and muscle there is a clear distinction between mechanorecptive and nociceptive afferents. However, this is not the case in visceral tissue, for which pain may not be reported even in response to tissue-damaging stimuli. Nociceptors are located in visceral organs, including the heart, gastrointestinal tract and reproductive organs, and in the walls of blood vessels. Pain-producing stimuli in the viscera include inflammation, distension of hollow muscular-walled organs such as the gastrointestinal tract, the urinary tract and the gall bladder, ischaemia in organs such as the heart, and traction in the mesentery. Inflammation of visceral organs can induce central sensitization (see Chapter 6) of dorsal horn neurons and may lead to referred hyperalgesia.
Visceral nociceptors are also sensitive to irritating chemicals. Afferent nociceptive fibres in viscera are found in association with both sympathetic and parasympathetic efferents (Meyer et al 1994).
NON-NEURONAL CELLS
It has been increasingly recognized that non-neuronal cells such as immune cells (macrophages and lymphocytes) and glial cells in the peripheral nervous system (Schwann cells and satellite cells) and central nervous system (astrocytes and microglia) play a critical role in chronic pain processing (see Milligan & Watkins 2009 for review). Nerve injury induces substantial changes in both microglia and astrocytes in the spinal cord (Ji et al 2006).
ANATOMY OF REFERRED PAIN
Pain from stimulation of viscera is frequently localized to the surface of the body, a phenomenon termed referred pain. This may be explained by the convergence of nociceptive input for deep and cutaneous tissues onto common somatosensory spinal neurons that also receive afferents from topographically separate body regions (the so-called projection–convergence theory; Ruch 1946). There are no separate ascending spinal pathways dedicated to the signalling of sensations from the viscera. These sensations are represented within the known somatosensory pathways. The level of the spinal cord to which visceral afferent fibres from the internal organs project depends on their embryonic innervation. Many viscera migrate well away from their embryonic origin during development, and therefore referred pain from the viscera may be perceived at locations remote from the actual site of the stimulus. For example, the heart is derived from endoderm in the neck and upper thorax, with the result that nociceptive afferents from the heart enter the spinal cord through the dorsal roots C3–T5 rather than lower down. Similarly, afferents from the gall bladder enter the spinal cord at T9 rather than at L1, its location later in life. The pain signals carried by these fibres may be referred to the areas of skin via Aδ fibres to the same segment of the spinal cord. Hence, a heart attack causing ischaemia of cardiac muscle can often present as pain in the left shoulder passing into the left arm (the cutaneous segments supplied by C3–T5). In the same way, an inflamed gall bladder can frequently cause pain at the tip of the right scapula, supplied by T9.
Pain may also be referred from tissues other than viscera, and frequently occurs in musculoskeletal conditions. This type of referred pain has been explained with reference to patterns of dermatomal, myotomal and sclerotomal territories. However, there is enormous individual variation in these territories, as well as variability in presenting symptoms (Grieve 1994).
DORSAL ROOT GANGLION CELLS
The somas of nociceptive afferents are located in the dorsal root ganglia (DRG) and the equivalent ganglia of cranial nerves V, VII, IX and X. DRG cells are pseudo-unipolar neurons conveying information from the periphery into the spinal cord. These cells can be grouped into two classes based on variations in soma size, diameter of axons, morphology of peripheral terminals and site of central terminations. The division into two size classes has a functional correlate in that, generally, large cells giving rise to large-diameter axons relay low-threshold mechanical and proprioceptive stimuli, and small cells relay nociceptive and thermal stimuli (Lawson 1992). Small DRG cells stain intensely and contain many organelles and peptides, including substance P (SP), somatostatin, calcitonin gene-related peptide (CGRP), vasoactive intestinal peptide (VIP) and galanin (Willis & Coggeshall 1991).
PRIMARY AFFERENTS
As primary afferent fibres in peripheral nerves travel towards the spinal cord, they group together to form spinal nerves, each spinal nerve supplying a discrete area of skin (dermatome), and overlapping to a greater or lesser degree the dermatomes of neighbouring spinal nerves. Each spinal nerve splits to form a ventral and dorsal root. The dorsal roots are purely sensory, but a considerable number of small-diameter unmyelinated afferent fibres are located in the ventral root (ventral root afferents; Coggeshall et al 1974; Light & Metz 1978). The majority of these fibres appear to end blindly, with the rest either looping within the ventral roots or branching into the dorsal root (Willis & Coggeshall 1991). The function of the ventral root afferents is, as yet, unclear.
Sorting of primary afferent fibres occurs in the dorsal roots in primates (Snyder 1977). Large-diameter afferents subserving mechanoreception enter the spinal cord in the medial division of the dorsal root, while small-diameter fibres form a lateral bundle. The fibres enter the cord at the dorsal root entry zone. The bundle of small-diameter fibres contains those involved in nociception, together with fibres involved in temperature and visceral sensation. They divide into short ascending and descending branches that run longitudinally in the dorsolateral fasciculus of Lissauer. Within several segments they leave the tract to synapse on neurons in the dorsal horn.
THE DORSAL HORN
The dorsal horn of the spinal cord is the first site for integration and processing of incoming sensory information. The dorsal horn has historically been divided into three broad regions: the marginal zone, the substantia gelatinosa and the nucleus proprius. Rexed (1952, 1954) divided the grey matter of the dorsal horn into six laminae based on cytoarchitectural criteria, the most dorsal being lamina I (Fig. 5.1). Further anatomical and physiological studies have since confirmed functional differences in dorsal horn neurons in different laminae, as well as different patterns of projections. In addition, cells, axons and terminals in the different laminae of the dorsal horn have a distinctive chemical profile, which has been shown to change following a lesion (see Willis & Coggeshall 1991 for review).
Fig. 5.1 The laminae of the spinal cord based on the description of Rexed (1952, 1954). Laminae I–VI comprise the dorsal horn.
Primary afferent fibres terminate in different laminae depending on their function (Fig. 5.2).
Fig. 5.2 The terminations of afferent fibres in the dorsal horn vary by depth. Large fibres (Aα and Aβ) enter in the medial division and small fibres (Aδ and C) enter in the lateral division of the dorsal root.
Lamina II is also called the substantia gelatinosa. The most prominent structures in lamina II are complex structures called glomeruli through which a primary afferent terminal can make synaptic contact with several peripheral dendrites, axonal terminals and cell bodies (Kerr 1975). Glomeruli are key structures of the dorsal horn because they offer a morphological basis for both presynaptic and postsynaptic modulation of the primary afferent input. They comprise a central primary afferent terminal that makes contact with a group of between four and eight surrounding dendrites and other peripheral axon terminals, and are set apart from the surrounding tissue by glial processes (Fig. 5.3). Using morphological criteria, the peripheral terminals appear to have the characteristics of inhibitory synapses and may contain the inhibitory neurotransmitters γ-aminobutyric acid (GABA) or enkephalin. The central terminals have the characteristics of excitatory terminals and may contain CGRP, glutamate, SP, cholecystokinin or serotonin. The glomerulus therefore comprises a complicated arrangement of inhibitory synaptic terminals surrounding an excitatory primary afferent ending.
Fig. 5.3 Diagram of a glomerulus based on the work of Kerr (1975). A central terminal; B, dendrites; C, dendritic spines from lamina II neurons. Shaded areas represent glial processes.
A population of neurons responding to noxious mechanical and thermal stimuli has been reported in lamina X in the vicinity of the central canal of the spinal cord. In addition to these high-threshold type cells, lamina X also contains neurons that are low threshold and have a wide dynamic range. Many of the cells have convergent input from visceral afferent fibres, and some of these respond only to visceral stimuli (Honda 1985; Honda & Perl 1985).
Terminations of afferent fibres in the dorsal horn
The incoming afferent fibres of all types establish a web of connections with dorsal horn neurons, exerting a changing pattern of excitatory and inhibitory inputs that determines the firing of dorsal horn projection neurons and of interneurons that mediate spinal reflex responses (Table 5.2). Normally there is a certain degree of segregation in the termination pattern for different afferent fibre classifications in the dorsal horn, but this may not be the case in pathological situations. Woolf et al (1992) showed that peripheral nerve injury triggers sprouting of myelinated afferents into lamina II, resulting in the possibility of functional contacts of low-threshold mechanoreceptive afferents with cells that normally have only C-fibre input.
Table 5.2
Terminations of afferent fibres in the dorsal horn
Afferents | Terminal zones in dorsal horn |
Large diameter myelinated fibres | III, IV and V |
Small diameter myelinated fibres (Aδ) | I and V |
Unmyelinated fibres (C ) | II, III |
Visceral projections | II, IV–V and X |
Large-diameter myelinated fibres
Collaterals of the large Aβ fibres initially travel ventrally through the dorsal horn but reverse when they reach the deeper laminae, and break up to give rise to large flame-shaped arbors as they course dorsally. These terminations are dense in laminae III, IV and V. It has been shown that the distal parts of the arbors of hair-follicle afferents enter the innermost part of lamina II (Brown 1981).
Small-diameter myelinated fibres
Collaterals of Aδ fibres terminate both superficially and deep within the dorsal horn. High-threshold afferents terminate profusely with arborizations in lamina I. Low-threshold hair afferents pass through lamina I to terminate in lamina V (Mense & Prabhakar 1986).
Unmyelinated fibres
Unmyelinated afferents terminate in the superficial dorsal horn. Lamina II is the site of termination of primary afferent fibres from the skin, with visceral afferents terminating in laminae I and II with some extension to lamina III (Gobel et al 1981; LaMotte 1977).
Peptidergic C fibres terminate in lamina I and the dorsal part of lamina II. Non-peptidergic fibres terminate in mid-lamina II. The ventral part of lamina II has excitatory interneurons expressing the gamma isoform of protein kinase C, which has been implicated in persistent pain post-injury (Malmberg et al 1997).
Visceral projections
Myelinated axons innervating abdominal and pelvic viscera can be classified into high- or low-threshold mechanoreceptive groups. They project to lamina I or deeper laminae V–VI (de Groat et al 1981; Morgan et al 1981).
Visceral afferent fibres terminate predominantly in lamina I, but have also been reported in laminae II, IV–V and X (Sugiura et al 1989).
Somatotopic organization of dorsal horn
The dorsal horn in the cervical and lumbar enlargements appears to be somatotopically organized with distal regions being represented medially and proximal areas laterally. There is a rostrocaudal representation of the digits, with the first digit being represented most rostrally and the fifth digit most caudally (Brown et al 1989; Florence et al 1988; Wilson et al 1986).
Response properties of dorsal horn neurons
Three major classes of dorsal horn neurons have been recognized (McMahon 1984):
1. low-threshold mechanosensitive (LTM), in which the cells are only excited by low-threshold innocuous stimuli such as hair movement, touching or brushing the skin
2. nociceptive-specific (NS), where only high-threshold noxious or near-noxious levels of peripheral stimulation excite the cells
3. wide dynamic range (WDR) or convergent cells, where the firing rate of the cells is increased by innocuous events, but further increased when stimulus intensity is raised to noxious levels.
This classification does not imply that each class of neurons is homogeneous. The properties of neurons in the dorsal horn depend to some extent on their location. A proportion of lamina I neurons is NS (Christensen & Perl 1970), but WDR cells form the largest population of neurons in this lamina. The dendrites of lamina I cells remain within the lamina and these cells give rise to an ascending projection to the thalamus (Trevino & Carstens 1975). Lamina II cells are predominantly of the WDR type, while lamina III cells are predominantly LTM.
Wall (1967) demonstrated that lamina IV cells were predominantly of the LTM type and often received input from large-diameter afferents only. Their receptive fields, located in distal regions of the body, tend to be smaller and have distinct edges. In lamina V, all three classes of cells are represented but the WDR type more frequently. Many of these cells have long ascending axons that reach supraspinal levels, some of them directly reaching the thalamus. WDR cells are excited by C fibres as well as by A fibres, and this input reaches them either via lamina II cells or by synapses on their distant dendrites. Many lamina V cells have dendrites which reach lamina II.
SPINAL CORD TRANSMISSION PATHWAYS
Ascending tracts
Somatosensory signals are conveyed along two major ascending systems in the spinal cord: the anterolateral system and the dorsal column-medial lemniscal system. The latter carries information about tactile sensation and limb proprioception. The anterolateral system relays information predominantly about pain and temperature, but also some tactile information. It comprises three pathways: the spinothalamic tract, the spinoreticular tract and the spinomesencephalic tract (Fig. 5.4).
Fig. 5.4 Organization of the anterolateral system. Primary axons terminate in the dorsal horn. Second-order axons cross the midline and ascend in the anterolateral funiculus in the spinal cord and the spinal lemniscus in the brainstem to terminate in the thalamus. Collaterals of these axons terminate in the reticular formation (spinoreticular axons) and in the periaqueductal grey matter (spinomesencephalic axons) (shaded). Thalamocortical axons then project to the somatosensory cortex.
The spinothalamic tract (STT) originates from neurons along the length of the spinal cord, with a particularly large concentration of STT cells in the uppermost cervical segments, including a large ipsilateral group of neurons in the ventral horn. Below this level, the majority of STT neurons are contralateral to their target. In the primate, STT neurons are located in three main regions: laminae I, IV–V and VII–VIII and comprise both nociceptive-specific and wide dynamic range neurons. Some STT neurons are also in laminae II, III and X (Apkarian & Hodge 1989a). The axons cross the midline in the dorsal and ventral white commissures at a level near the cell body and ascend to the thalamus in the lateral funiculus on the contralateral side. While most of the axons occupy the ventral lateral quadrant of the spinal white matter, some axons, particularly those originating from lamina I cells, ascend in the dorsal lateral quadrant (Apkarian & Hodge 1989b). The STT in the lateral funiculus has a somatotopic organization. Axons from the most caudal regions of the spinal cord occupy the most dorsolateral position, with axons from progressively more rostral levels joining the tract in more ventromedial positions (Applebaum et al 1975). In the brain stem the STT passes dorsolateral to the inferior olivary nucleus in the medulla, then ascends dorsolateral to the medial lemniscus through higher levels of the brain stem to the thalamus. The spinothalamic tract transmits nociceptive and thermal information, as well as touch sensations.
The spinoreticular tract (SRT) in the primate originates from neurons in laminae VII and VIII, as well as the lateral part of lamina V. Some neurons are in lamina X. The majority of neurons giving rise to the SRT are in the uppermost cervical segments, although cells from all levels of the spinal cord are involved (Kevetter et al 1982). There are two components: a projection to the lateral reticular nucleus (a pre-cerebellar nucleus) and a projection to the pontine and medullary reticular formation, which gives rise to descending pathways to the spinal cord. Most of the projections from the cervical and lumbar enlargements cross the midline near the level of the cell bodies, but some axons originating from cervical segments remain uncrossed. They ascend in the ventral lateral column of the spinal cord with the STT and form a prominent bundle lateral to it in the brain stem. The SRT has no obvious somatotopic organization and terminates in the following nuclei of the reticular formation: nucleus medullae oblongatae centralis, lateral reticular nucleus, nucleus reticularis gigantocellularis, nucleus reticularis pontis caudalis and oralis, nucleus paragigantocellularis dorsalis and lateralis, and nucleus subcoeruleus (Mehler et al 1960).
The spinomesencephalic tract (SMT) is a collection of pathways from the spinal cord to several different midbrain nuclei. It originates primarily from neurons in laminae I, V, VII and X (Zhang et al 1990). The majority of axons cross the midline and ascend with the STT and SRT in the ventral lateral column. The SMT projects to the nucleus cuneiformis, the parabrachial nucleus, the intercollicular nucleus, the deep layers of the superior colliculus, the nucleus of Darkschewitsch, the anterior and posterior pretectal nuclei, the red nucleus, the Edinger–Westphal nucleus, the interstitial nucleus of Cajal and the periaqueductal grey (Yezierski 1988). The SMT is roughly somatotopically organized, with the projection from the cervical enlargement terminating more rostrally than that from the lumbosacral region. The periaqueductal grey contains neurons that are part of a descending pathway that regulates pain transmission.
Other tracts in the dorsolateral funiculus and the dorsal columns also convey nociceptive information (Fig. 5.5). The spinocervical tract (SCT) originates from laminae III and IV at all levels of the cord where neurons respond predominantly to tactile stimuli, but some are activated by noxious stimuli. The axons project ipsilaterally in the dorsolateral funiculus to the lateral cervical nucleus, located just ventrolateral to the dorsal horn in segments C1–C3. The lateral cervical nucleus is quite small in the primate (Mizuno et al 1967), and may be present in some humans, although it is possible that the nucleus may not be distinctly separate from the dorsal horn in some cases (Ha & Morin 1964; Kircher & Ha 1968; Truex et al 1965). Most of the neurons in the lateral cervical nucleus cross the midline in the ventral white commissure and ascend in the medial lemniscus to midbrain nuclei and to the thalamus.
Fig. 5.5 Organization of the dorsal column-medial lemniscal system. Large-diameter axons enter the spinal cord and ascend in the dorsal columns to the medulla, where they synapse in the dorsal column nuclei (nucleus gracilis and nucleus cuneatus). Second-order axons cross the midline and ascend in the medial lemniscus to terminate in the VPL nucleus of the thalamus. Thalamocortical axons then project to the somatosensory cortex.
The dorsal column-medial lemniscal system consists of branches of primary afferent fibres conveying tactile and proprioceptive information, as well as the axons of neurons in laminae IV–VI. There are also unmyelinated primary afferent fibres that synapse in the dorsal column nuclei, presumably arising from nociceptors (Patterson et al 1990). In addition, there is a visceral pain pathway ascending in the dorsal column (Al-Chaer et al 1998; Hirschberg et al 1996; Willis et al 1999). The medially located fasciculus gracilis contains a representation of the lower part of the trunk and lower extremity, whereas the fasciculus cuneatus lateral to it contains a representation of the upper part of the trunk and the upper extremity. This tract projects to the dorsal column nuclei in the medulla, from which axons ascend in the medial lemniscus to the thalamus. The sensory representation in the dorsal column nuclei is somatotopic with caudal parts of the body represented medially in the nucleus gracilis and the rostral regions laterally in the nucleus cuneatus. The trunk representation is in a region between the two nuclei. The distal extremities are represented dorsally and the proximal body ventrally (Johnson et al 1968).
TRIGEMINAL SYSTEM
The sensory nuclei of the trigeminal nerve consist of three different parts. From caudal to rostral these are the nucleus of the spinal tract, the main or principal sensory nucleus and the mesencephalic sensory nucleus (Fig. 5.6
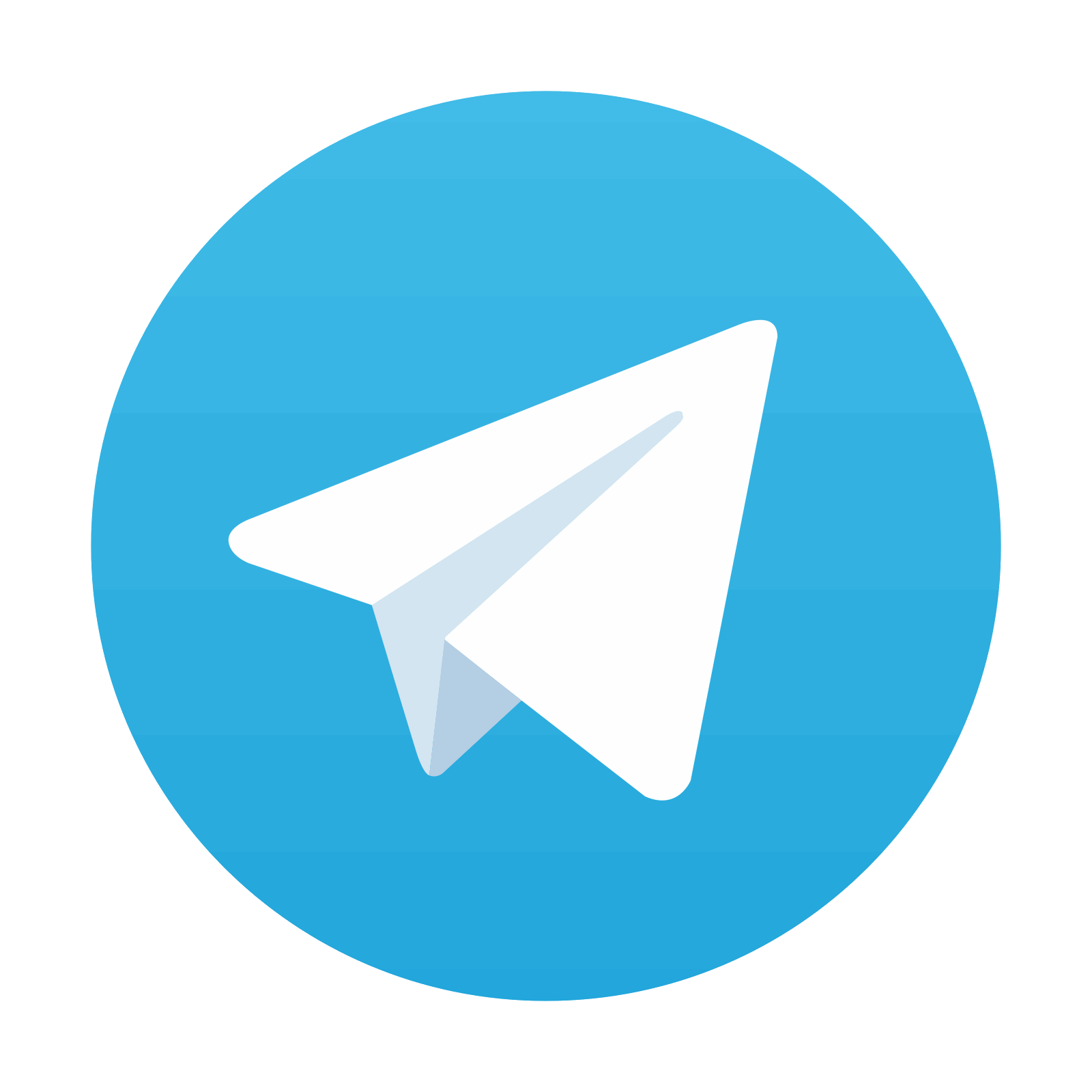
Stay updated, free articles. Join our Telegram channel

Full access? Get Clinical Tree
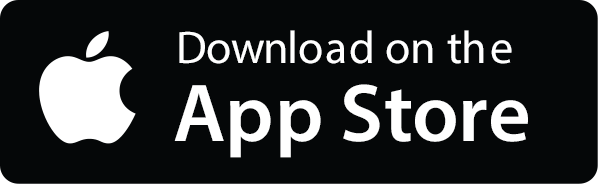
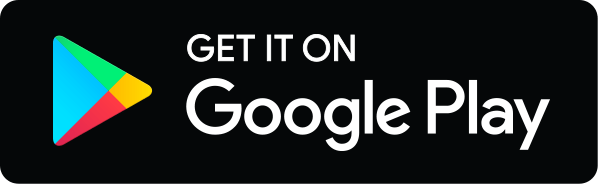