Chapter 24 The most common cause of death in the critically ill surgical patient is multiple organ failure (MOF).1 This concept was first described in the 1960s. As treatments evolved, so did morbidity and mortality, and increasingly patients survived long enough to develop MOF. In 1991, the American College of Chest Physicians and the Society for Critical Care Medicine held a consensus conference leading to more formal definitions of sepsis and multi organ failure. MOF was revised to multiple organ dysfunction syndrome (MODS), defined generally as altered function in at least two organ systems in the setting of acute illness that results in impaired homeostasis, requiring intervention.2 This consensus statement attempted to standardize these terms for the purposes of investigation and prognostication. The subsequent publication of the MODS score in 1995 was the first objective scale to measure the severity of MODS as an outcome in critical illness.3 This score is summarized in Table 1. While the MODS score is not considered a true prognostic score, the risk of intensive care unit (ICU) mortality increases with severity of organ dysfunction, both in the number of organs involved and the degree of dysfunction. Outcomes in MODS are summarized in Tables 2 and 3. The MODS score focuses on objective measures of physiologic dysfunction in six organ systems: respiratory, cardiac, renal, hepatic, neurologic and hematologic. These systems will form the basis of the following discussion of MODS in the surgical patient. The discussion will hopefully increase the awareness of the intensivist to the likelihood of MODS in its earliest phases and prompt timely diagnostic and therapeutic interventions to improve outcome. Table 2. Outcomes in MODS by score.
Multiorgan Dysfunction Syndrome in the Surgical Patient
P. Dhar and G. Papia
Chapter Overview
MODS score | ICU mortality (%) | Hospital mortality (%) |
0 | 0 | 0 |
1–4 | 1–2 | 7 |
5–8 | 3–5 | 16 |
9–12 | 25 | 50 |
13–16 | 50 | 70 |
17–20 | 75 | 82 |
21–24 | 100 | 100 |
Table 3. Outcomes in MODS by organ system involvement.
Number of failing organ systems | Mortality (%) |
0 | <10 |
1 | 0–30 |
2 | 20–50 |
3 | 40–80 |
4 | 60–100 |
5+ | >80 |
Respiratory
Respiratory failure manifests as impaired gas exchange, reflected as hypoxemia and hypercapnia. While multiple clinical syndromes can be grouped in this category, the following sections focus on what are, arguably, the most significant in the surgical population: acute respiratory distress syndrome (ARDS) including the related transfusion related acute lung injury (TRALI) and pulmonary embolus (PE).
ARDS
ARDS is a leading cause of postoperative respiratory failure. The most up to date definition of ARDS is described by the Berlin definition as outline in Table.4 Several prediction models have been developed to identify patients at risk for the development of ARDS, including some that focus specifically on surgical populations. The surgical lung injury prediction (SLIP) score was designed for elective surgical procedures, and includes variables of surgical procedure (high risk cardiac, vascular and thoracic surgery), comorbid conditions (diabetes mellitus, chronic obstructive pulmonary disease, gastroesophageal reflux disease), and modifying conditions (alcohol abuse).5 The recently updated SLIP-2 addresses more heterogeneous high risk surgical populations, including those presenting as emergencies. The SLIP-2 model is summarized in Table 5.6 A number of algorithms have also been developed for the trauma population, the most recent of which was published in 2012.7–9 This model incorporates variables including APACHE II, injury severity score, blunt mechanism of injury, pulmonary contusion, flail chest, massive transfusion and older age.
Table 4. Berlin definition of acute respiratory distress syndrome.
TIMING | — Within 1 week of a known clinical insult or new/worsening clinical symptoms. |
CHEST IMAGING | — Bilateral opacities not fully explained by effusions, lobar/lung collapse or nodules. |
ORIGIN OF EDEMA | — Respiratory failure not fully explained by cardiac failure or fluid overload. |
| — Requires objective assessment e.g., ECGO to rule out hydrostatic edema if no risk factor is present. |
OXYGENATION* | |
Mild | 200 mmHg < PaO2/FiO2 ≤ 300 mmHg |
Moderate | 100 mmHg < PaO2/FiO2 ≤ 200 mmHg |
Severe | PaO2/FiO2 ≤ 100 mmHg |
*With PEEP or CPAP ≥ 5 cm H2O.
Predictor variables | Slip points |
Surgical Procedure | |
High risk vascular surgery | 32 |
High risk cardiac surgery | 19 |
High risk thoracic surgery | 16 |
Comorbid conditions | |
Diabetes mellitus | 6 |
COPD | 10 |
GERD | 7 |
Modifying conditions | |
Alcohol abuse | 11 |
The ability to predict those surgical patients at high risk for the development of respiratory failure is helpful in studying potential interventions. The so-called magic bullet for ARDS in the general critical care population has yet to be identified.
Ventilation Strategies
Lung protective ventilation is one intervention which is regarded as both a preventative and treatment strategy for ARDS. It is based on the rationale that low tidal volume ventilation is less likely to cause alveolar injury from over distension. This ventilation strategy, targeting a tidal volume of 6 mL/kg of ideal body weight, has been shown to be of benefit in a number of trials demonstrating decreased mortality and days of mechanical ventilation.10 High frequency oscillation (HFOV) is another ventilation strategy, incorporating the rationale for low tidal volume ventilation that has been studied in the treatment of ARDS. Recent randomized trials suggest that HFOV has no mortality benefit in ARDS, and may actually cause harm.11,12 Despite these recent studies, HFOV is still considered potential rescue therapy in the management of ARDS.
Prone Positioning
Early randomized trials investigating the use of prone positioning in ARDS did not show a mortality benefit, and the management strategy fell out of favor in many centers. Based on the rationale of improved ventilation-perfusion matching in ARDS afflicted lungs, this technique was recently readdressed in the literature with the PROSEVA trial.13 This study used a more prolonged period of prone positioning of 16 hours compared to previous trials. Patients categorized as severe ARDS (criteria PaO2:FiO2 <150 mmHg, FiO2 ≥0.6, PEEP ≥ 5 cm H2O) did actually show a mortality benefit, without an increase in complications.
Pharmacologic
Inhaled vasodilators have been investigated in the treatment of ARDS. Meta-analyses have shown that nitric oxide (NO) at least transiently improves oxygenation, without an improvement in mortality or duration of mechanical ventilation.14 NO therapy is expensive, with a theoretical risk of methemoglobinemia, and a reported increased risk of renal impairment. At this time, the use of NO therapy in ARDS is limited to the setting of refractory hypoxemia.
The use of steroids in ARDS remains an area of controversy. Several meta-analyses have addressed the use of glucocorticoids in this setting, but the benefit remains unclear. There is a suggestion that steroids given early in the clinical course may be of some benefit, where as those patients treated after 14 days may actually have higher mortality.15 This potential benefit has to be weighed against the morbidity of steroids in the critical care setting, including neuromuscular weakness and the potential for major fractures and potentiation of sepsis.
Extracorporeal Membrane Oxygenation (ECMO)
ECMO has been studied as salvage therapy in patients with hypoxemic respiratory failure refractory to conventional ventilatory management. In venovenous ECMO, a cannula is placed in a central vein which facilitates withdrawal of blood into an extracorporeal circuit that directly oxygenates and removes carbon dioxide from the blood. The oxygenated blood is returned to a central vein, bypassing the need for gas exchange at the level of the lung. It is important to note that patients must be anti-coagulated to maintain the ECMO circuit, and this must be taken into account in the surgical patient with ARDS. Current guidelines suggest that ECMO therapy should be considered for patients with a PaO2/FiO2 < 150 on FiO2 > 90% and is indicated in those with a PaO2/FiO2 < 100 on FiO2 > 90%.16 These guidelines also suggest that a decision to initiate ECMO should be made within seven days of mechanical ventilation at high settings (e.g., FiO2 > 90% and plateau pressures greater than 30 cm H2O).
PE
Pulmonary embolism is implicated in approximately 10% of hospital deaths.17 The physiologic implications of PE involve multiple systems including cardiovascular (e.g., cardiogenic shock) and respiratory (e.g., pulmonary infarction, hypoxemic respiratory failure). The patients who develop symptomatic or clinically significant PE in the acute setting are vulnerable to developing multi-organ dysfunction, either as a precipitating event or as a complication of ICU admission. Even relatively small PEs may be tolerated poorly by the critically ill. There are multiple risk factors for PE in the surgical patient, including the type and duration of surgery, trauma, postoperative immobilization, previous venous thromboembolism (VTE), malignancy, and inherited or acquired hypercoaguable states. The current gold standard for diagnosis of pulmonary embolism is CT-angiography. When IV contrast cannot be administered, V/Q scanning and/or lower extremity doppler ultrasound can be obtained. If the patient is too unstable for these imaging techniques bedside echocardiography can be performed to identify secondary signs of pulmonary embolism (e.g., right ventricular systolic pressure [RVSP], evidence of right ventricular strain, septal flattening).18 The approach to management of pulmonary embolism is divided into two arms: its prevention and its treatment. The American College of Chest Physicians (ACCP) publishes updated guidelines for both the prevention and treatment of VTE.19
Prevention
Several models of risk assessment for VTE in surgical patients have been developed. The 2012 guidelines use a modification of the Caprini risk assessment model which stratify patients into categories of very low risk, low, moderate and high risk for postoperative VTE with recommendations for perioperative thromboprophylaxis accordingly. These guidelines also differentiate between orthopedic and non-orthopedic surgical procedures, the former conferring higher risk of VTE. It is established that patients who have had surgery prior to ICU admission are at higher risk for VTE, but this risk is compounded in critical illness with its associated immobilization and increased risk of postoperative bleeding. Implementation of evidence-based care bundles in ICU patients has been shown to improve outcomes. VTE prophylaxis is included in the so-called ventilator bundle. Appropriate use of chemoprophylaxis, or alternatively mechanical VTE prophylaxis, in the critically ill is now used as a quality indicator in the ICU.
Treatment
Treatment of PE is determined by the patient’s clinical stability, diagnostic certainty and any contraindications to anticoagulation. Cardio-respiratory compromise requires stabilization of airway and hemodynamics in the critical care setting. Patients with confirmed PE and persistent hypotension are appropriate candidates for systemic thrombolysis.19 The risks of major bleeding are accepted for the patient in shock, but the role of thrombolysis — both systemic and catheter — directed — in patients who are hemodynamically stable but with evidence of right ventricular strain or extensive clot burden, is less clear. Patients who are hemodynamically stable are typically started on parenteral anticoagulation for initial management, with transition to oral agents once stabilized.19 Those patients who are hemodynamically stable but have contraindications to anticoagulation are considered for inferior vena cava filter placement to prevent propagation of clot from the lower extremities, until they become candidates for full anticoagulation.
Cardiovascular
Cardiovascular (CV) dysfunction in multiorgan failure is an interesting problem because it has direct implications on every other organ system. The MODS score uses heart rate (HR), central venous pressure (CVP), and mean arterial pressure (MAP) to determine the pressure-adjusted heart rate (PAR) to measure degree of cardiac dysfunction. With this equation (PAR = HR × CVP/MAP), higher values reflect worsening CV dysfunction and therefore MODS scores. The practical clinical manifestation of CV dysfunction in this setting is essentially hypotension refractory to increasing preload.20
The discussion of primary CV dysfunction resulting in myocardial infarction, is beyond the scope of this discussion of MODS. The pathophysiology of secondary CV dysfunction in multiorgan failure is thought to involve a number of sequential and parallel steps:
1. Tissue injury/sepsis results in endothelial damage, neuroendocrine activation and release of inflammatory mediators.
2. Massive immune/inflammatory response results in:
— Peripheral vasodilation mediated by NO;
— Increased capillary permeability;
— Microvascular stasis and thrombi causing arteriovenous shunting resulting in high mixed venous oxygen saturation.
3. Redistribution of regional blood flow results in selective tissue hypoperfusion.
4. Acidosis and impaired cellular function result in myocardial depression, and autonomic dysfunction.
The management of CV dysfunction in MODS is primarily supportive. Vasoactive medications including pressors and inotropes are used for hemodynamic support after a trial of fluid resuscitation. Determining fluid responsiveness in the critically ill population is an area of extensive study (see chapter on Shock and cardiovascular dynamics). At a physiologic level, responsiveness is measured as an increase in stroke volume with fluid challenge. Based on the Frank Starling curve, as preload increases, left ventricular stroke volume increases until optimal preload is achieved. After this point, stroke volume remains relatively constant and the patient is no longer fluid responsive and vasoactive medications should be considered. Guidelines for resuscitation, such as the Surviving Sepsis Campaign, identify end points of resuscitation such as central venous pressure, mean arterial pressure, urine output, normalization of lactate and mixed venous oxygen saturation although central venous pressure is no longer regarded as a reliable index of volume responsiveness.21
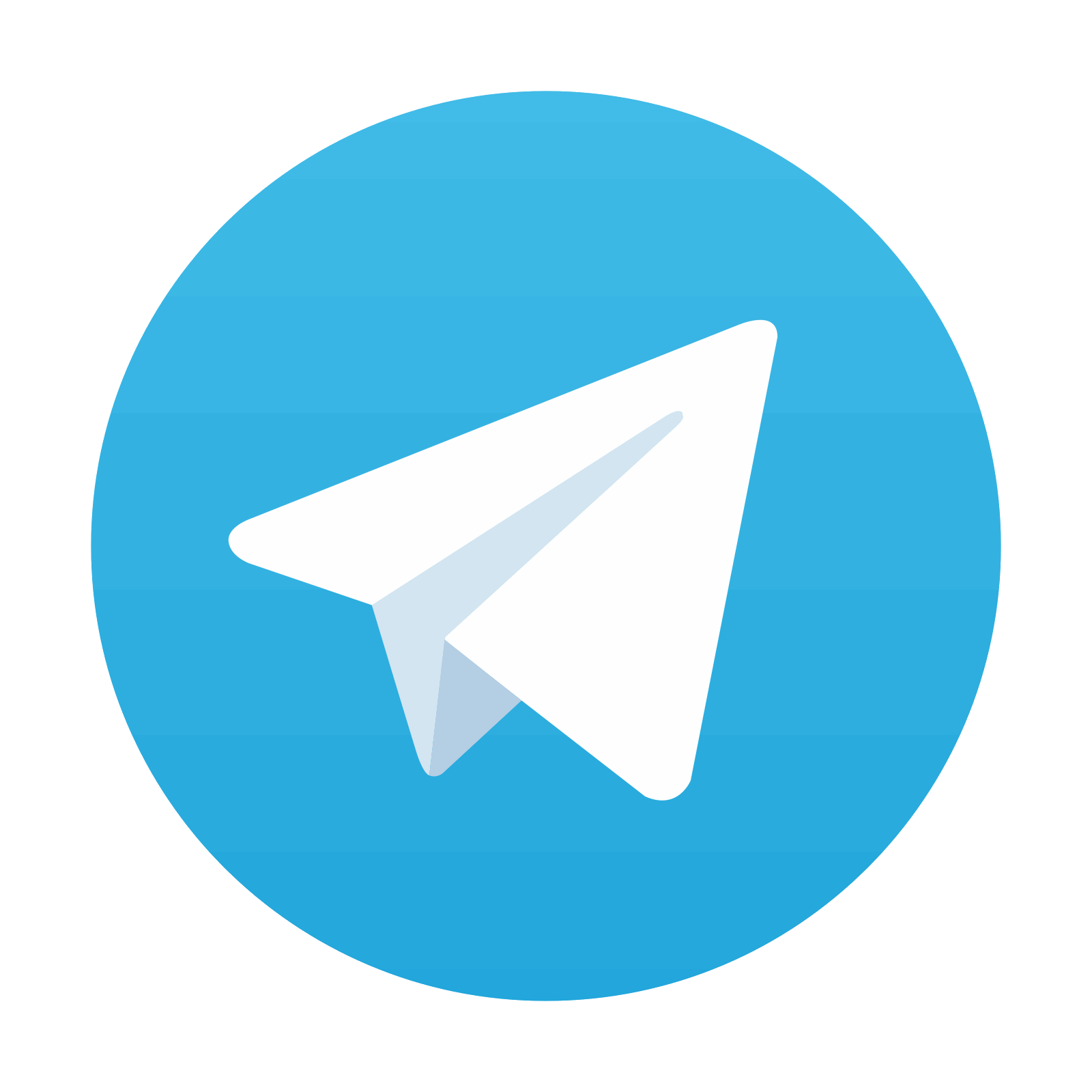
Stay updated, free articles. Join our Telegram channel

Full access? Get Clinical Tree
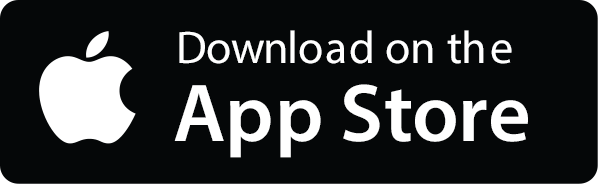
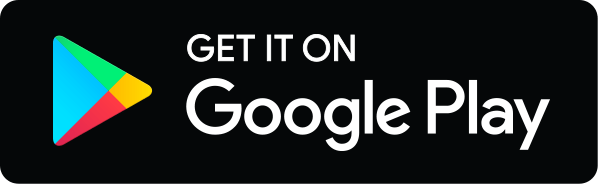