Key Points
- ▪
Anesthesiologists rely extensively on physiologic signals and anesthetic dosing strategies to infer and to track states of the brain and central nervous system under general anesthesia.
- ▪
Heart rate and systemic arterial blood pressure changes are the principal physiologic signals used to monitor the anesthetic state of patients receiving general anesthesia.
- ▪
Use of the neurologic examination during induction of and emergence from general anesthesia can provide information regarding loss and recovery of consciousness.
- ▪
Electroencephalogram (EEG)–based indices are used to track the level of unconsciousness of patients receiving general anesthesia. The most commonly used EEG-based indices are the bispectral index (BIS), the Patient Safety Index (PSI), Narcotrend, and Entropy.
- ▪
Real-time analysis of the unprocessed EEG and the spectrogram (density spectral array) is a highly informative way to monitor the level of unconsciousness of patients receiving general anesthesia.
- ▪
Use of the unprocessed EEG and spectrogram for real-time monitoring of the level of unconsciousness is made possible by the fact that the EEG oscillations of anesthetized patients change systematically with anesthetic dose, with anesthetic class (mechanism of action), and patient age.
- ▪
Anesthetic-induced oscillations are one of the primary mechanisms through which these agents induce altered arousal states such as sedation and unconsciousness. Therefore, real-time monitoring of the unprocessed EEG and the spectrogram provide a scientifically based, practical, and patient-specific way to track sedation and unconsciousness in the operating room.
- ▪
Normalized symbolic transfer entropy (NSTE) is an approach for quantifying how changes in frontoparietal functional connectivity relate to anesthesia-induced changes in consciousness.
- ▪
In the future, closed-loop anesthetic delivery (CLAD) systems may provide highly accurate ways to control states of general anesthesia, medical coma, and sedation.
- ▪
Obtaining reliable quantitative markers of nociception is an active area of investigation.
General anesthesia is a drug-induced reversible condition composed of four behavioral and physiologic states: anti-nociception, unconsciousness, amnesia, immobility; and stability of the physiologic systems, including the autonomic, cardiovascular, respiratory, and thermoregulatory systems. Continuously monitoring the status of the patient during general anesthesia is crucial for safe and proper delivery of anesthesia care. The physiologic state of the patient under general anesthesia is commonly monitored using the electrocardiogram and an arterial blood pressure cuff, or an arterial catheter, to monitor the cardiovascular system. In more complex cases, a central venous catheter can be used to monitor central venous pressures, and a pulmonary artery catheter can be placed to monitor cardiac output and pressures in the heart and pulmonary circulation. Transesophageal echocardiography can be used intermittently to gain direct visual information about the anatomy and function of the heart. The capnogram provides a continuous readout of the level of expired carbon dioxide and respiration. In intubated patients, more detailed information about the state of the lungs can be acquired from the pressure and volume tracings on the ventilator. The pulse oximeter estimates the level of hemoglobin saturation in the arterial blood, and the thermometer tracks body temperature. Muscle relaxation, or immobility, is monitored primarily using a train-of-four stimulation device, and more grossly by observing changes in muscle tone or movement.
Monitoring the behavioral states is more challenging. During general anesthesia, amnesia is not monitored directly but implicitly by the extent to which unconsciousness is achieved. If the patient is unconscious and not simply conscious but unresponsive, the patient will likely have amnesia. In this chapter, we discuss approaches for monitoring unconsciousness and analgesia, or more accurately stated antinociception, and each of the three phases of general anesthesia: induction, maintenance, and emergence. This chapter’s focus is on physiologic signs and neurologic examination findings, and the electroencephalogram (EEG)–based measures, used to track these states of general anesthesia.
Induction of General Anesthesia
Monitoring of a patient’s level of consciousness begins at induction of general anesthesia. Induction is usually achieved by an intravenous bolus dose of a hypnotic drug such as propofol, a barbiturate, ketamine, or etomidate. Unconsciousness usually occurs in 10 to 30 seconds. The brain state of the patient, which in this case is the transition into unconsciousness, is tracked by monitoring the patient’s physiologic signs and the EEG-based index.
Physiologic Signs of Loss of Consciousness
When a hypnotic drug is administered to induce general anesthesia—usually as an intravenous bolus over a 5- to 10-second period—several physiologic signs are observed. If asked to count backwards from 100, the patient typically does not get beyond 85 to 90. This transition into unconsciousness can be followed easily by asking the patient to perform smooth pursuit of the anesthesiologist’s finger. In smooth pursuit, the patient is instructed to move his or her eyes to track the position of the anesthesiologist’s finger. As loss of consciousness ensues, the lateral excursions of the eyes during smooth pursuit decrease, nystagmus may appear, blinking increases, and the eyes fix abruptly in the midline. The oculocephalic reflex and the corneal reflex are lost, but the pupillary response to light can remain intact. The patient typically becomes apneic, atonic, and unresponsive at the point when the oculocephalic reflex is lost.
The oculocephalic reflex is assessed by turning the patient’s head from side to side, while lifting the eyelids. Before administration of the induction anesthetic, when the reflex is intact in a patient with no neurologic deficits, the eyes move in the direction opposite the motion of the head. When the reflex is lost, the eyes stay fixed in the midline. The oculocephalic reflex requires the circuits of cranial nerves III, IV, VI, and VIII to be intact. The motor nuclei associated with cranial nerves III and IV are located in the midbrain, whereas the nucleus of cranial nerve VI is located in the pons. The corneal reflex has traditionally been assessed using a wisp of cotton at the corner of the eye to stroke the cornea. An easier way to assess the reflex is to allow a drop of sterile water to fall on the cornea. Using a drop of sterile water may be safer than using the wisp of cotton, because the former is less likely to cause a corneal abrasion. With either approach, the reflex is intact if the eyes blink consensually, is impaired if there is a blink in one eye and not the other, and is absent if there is no blink. The afferent component of the corneal reflex travels to the sensory nucleus of the cranial nerve V through the ophthalmic branch, whereas the efferent component arises from the motor nucleus of the cranial nerve VII. The nuclei for these nerves associated with the oculocephalic reflex and the corneal reflex lie in close proximity to the arousal centers in the midbrain, pons, hypothalamus, and basal forebrain.
Loss of the oculocephalic reflex suggests that the motor nuclei required for eye movements have been affected by the anesthetic. Similarly, loss of the corneal reflex suggests that the nuclei that control sensation and motor responses to sensation on the eyes and the face have also been affected. Because the loss of the oculocephalic and corneal reflexes occur concomitantly with the loss of responsiveness, the anesthesiologists can also infer that the loss of consciousness is due at least in part to the effects of the anesthetics on the nearby arousal centers. Apnea, which commonly occurs on induction of general anesthesia with bolus administration of a hypnotic agent, is most likely due to the inhibitory effects of the anesthetic on the dorsal and ventral respiratory groups in the medulla and pons, respectively. Atonia can be due to anesthetic action at any one of multiple sites in the motor pathways between the primary motor areas and the spinal cord. The most likely brainstem sites are the pontine and medullary reticular nuclei.
Loss of the oculocephalic reflex, the corneal reflex, apnea, and atonia occur concomitantly with loss of consciousness on induction of general anesthesia because of the actions of the hypnotic drug in the brainstem after an intravenous bolus. Blood containing the anesthetic reaches the brainstem through the basilar artery, which supplies the posterior cerebral arteries that provide the posterior input to the circle of Willis. Before terminating in the posterior cerebral arteries, the basilar artery runs on the dorsal surface of the brainstem and gives off multiple penetrating arteries that perfuse the brainstem nuclei with the anesthetic leading to the observed physiological effects.
Electroencephalogram-Markers of Loss of Consciousness
EEG-based indices are among the most commonly used methods for tracking loss of consciousness induced by general anesthesia. With induction of general anesthesia, these indices usually change from high values that indicate the awake state to lower values that indicate states of sedation and unconsciousness.
Maintenance of General Anesthesia: Physiologic Signs and the Nociceptive-Medullary-Autonomic Pathway
Despite the many advances in anesthesia care, the physiologic signs of changes in heart rate, arterial blood pressure, and movement are the measurements most commonly used to track the anesthetic state during maintenance of general anesthesia. When the state of general anesthesia is not adequate for the level of surgical (nociceptive) stimulation, heart rate and arterial blood pressure can increase dramatically. The changes in heart rate and arterial blood pressure that anesthetized patients show in response to a nociceptive stimulus can be explained in terms of the nociceptive-medullary-autonomic (NMA) circuit composed of the spinoreticular tract, the brainstem arousal circuits, and the sympathetic and parasympathetic efferent pathways ( Fig. 40.1 ). It is important to understand how the NMA circuit works because it is the pathway most used by anesthesiologists for monitoring the patient’s level of unconsciousness and antinociception. One example is a description of the NMA pathway in a clinical context that is commonly observed in the operating room.

Suppose that a patient is in a stable state of general anesthesia when, to gain better exposure, the surgeon moves the retractor. Suppose also that this maneuver induces an immediate increase in heart rate and arterial blood pressure. Assuming, that there are no occult hemodynamic or respiratory problems or other common issues that increase heart rate and arterial blood pressure, then these increases likely occurred because the level of general anesthesia did not maintain an adequate level of antinociception. By simultaneously monitoring, in addition, the level of muscle relaxation and oxygen saturation, and oxygen delivery, along with EEG-based indices to track level of unconsciousness, the anesthesia provider can determine that these increases are due to inadequate anti-nociception and decide to administer more analgesic.
The ascending nociceptive (pain) pathway begins with A-delta and C-fibers whose free nerve endings bring nociceptive information from the periphery to the spinal cord (see Fig. 40.1 ). In the spinal cord, these fibers synapse in the dorsal horn on projection neurons that travel through the anterolateral fasciculus and synapse at multiple sites in the brainstem, including the nucleus of the tractus solitarius in the medulla. The autonomic response to a nociceptive stimulus is initiated from the nucleus of the tractus solitarius, which mediates sympathetic output through the rostral ventral lateral medulla and the caudal ventral lateral medulla to the heart and peripheral blood vessels through projections to the thoracolumbar sympathetic ganglia. The parasympathetic output from the nucleus of the tractus solitarius is mediated through the nucleus ambiguous, which projects through the vagus nerve to the sinoatrial node of the heart. The nucleus of the tractus solitarius also projects to the periventricular nucleus and supraoptic nucleus in the hypothalamus. Thus the nociceptive stimulus of moving the retractor initiates an increase in sympathetic output and a decrease in parasympathetic output through the NMA circuit that rapidly results in the observed increases in heart rate and arterial blood pressure.
The NMA circuit explains why increases in heart rate and arterial blood pressure are used as a rapid indicator of an inadequate level of antinociception. If unconsciousness is sufficiently well maintained, then no EEG changes will likely be observed. If no acute changes in physiology occur for other reasons, such as bleeding, hypoxemia, disconnection of the breathing circuit, or inadequate dosing of the muscle relaxant, the appropriate treatment is administration of more analgesic medication.
Activity in the NMA circuit can be observed rapidly when a patient is under general anesthesia, because this pathway is a fundamental component of the fight-or-flight response. This circuit is used as a sentinel for detecting nociceptive stimuli that can lead to autonomic, stress, and arousal responses. Heart rate and arterial blood pressure changes are the principal markers of activity under general anesthesia because motor responses are often blocked by muscle relaxation. Neurologists frequently test the NMA circuit with nociceptive stimuli, such as total body pinches, nail bed pinches, and sternal rubs, to evaluate the level of arousal of patients with brain injuries that affect the level of consciousness.
Other signs of inadequate antinociception are perspiration, tearing, pupil dilation, and return of muscle tone and movement. Changes in muscle tone and movement are not observed if the patient is receiving a muscle relaxant. The galvanic skin response has also been studied as a potentially more objective measure of antinociception. However, it is not used in clinical practice.
Maintenance of General Anesthesia: Electroencephalogram-Based Indices of Level of Consciousness
It is widely recognized that the EEG changes systematically in relation to the dose of anesthetic drug administered ( Fig. 40.2 ). As a consequence, the unprocessed EEG and various forms of processed EEG have been used to track the level of unconsciousness of patients receiving general anesthesia and sedation. Several EEG-based index systems have been studied and used in clinical practice. These systems process the EEG and provide an index value or set of values in real time or near real time that can be used to track the level consciousness. In general, the indices are designed to decrease with decreasing level of consciousness and increase as consciousness returns. In this way, the anesthesia provider can use these indices along with the physiological signs to track the patient’s state of unconsciousness and, to some degree, antinociception. A summary of the EEG-based indices that have received the most use in clinical practice and clinical studies follows.

Bispectral Index
The bispectral index (BIS) is an empirically derived scale that was proposed in 1994 by Aspect Medical Systems (later acquired by Covidien [Boulder, Colorado] and lastly by Medtronic [Minneapolis, Minnesota]) as a novel way to monitor anesthetic state in patients receiving general anesthesia and sedation. The algorithm processes the EEG in near real time and computes an index value between 0 and 100 that indicates the patient’s anesthetic state ( Fig. 40.3 ). A value of 100 corresponds to being completely awake, whereas 0 corresponds to a profound state of coma or unconsciousness that is reflected by an isoelectric or flat EEG. The BIS algorithm is proprietary, and the actual computation through which the index is derived is not public knowledge. However, it is known that BIS combines information from three EEG analyses: the spectrogram, the bispectrum, and a time domain assessment of burst suppression. The spectrogram is a decomposition of the EEG into its power content by frequency as a function of time. The bispectrum measures the degree of nonlinear coupling between pairs of frequencies in the spectrogram. The BIS algorithm works by measuring specific features of the spectrogram, the bispectrum, and the level of burst suppression, and uses a predetermined weighting scheme to convert these features into the index value. The EEG also undergoes various artifact corrections. Along with the index value, the unprocessed EEG, the spectrogram, and the level of electromyographic activity are displayed on the monitor. Production of the index is computationally intensive, so that there is a 20- to 30-second lag between the time the EEG is observed and the computation of the corresponding BIS value. A patient is considered to be appropriately anesthetized (i.e., unconscious) when the value of the BIS is between 40 and 60 (see Fig. 40.3 ). The EEG is recorded from a four-lead frontal montage.

Since its approval by the US Food and Drug Administration (FDA) in 1996, the BIS monitor has been studied extensively in clinical trials and used widely in anesthesiology practice. The changes in the index are correlated with the level of sedation and unconsciousness (see Fig. 40.3 ), primarily because for many anesthetics, the EEG shows lower-frequency, higher-amplitude oscillations as patients achieve deeper states of unconsciousness (see Fig. 40.2 ). Three exceptions are the anesthetics ketamine, nitrous oxide, and dexmedetomidine. The dissociative anesthetic state produced by ketamine is associated with prominent high-frequency oscillations rather than slow wave oscillations. As a consequence, patients can be unconscious with ketamine but have high index values.
The effect of nitrous oxide on the BIS index is ambiguous. Most recent studies state that nitrous oxide increases the amplitude of high-frequency EEG activity and decreases the amplitude of low-frequency EEG activity, yet it has little to no effect on the BIS index. However, these studies have not taken into account the state of profound transient large-amplitude slow oscillations, followed by lower amplitude gamma oscillations that have now been documented to be common features observed when nitrous oxide is administered in high-dose (>50%). In the case of dexmedetomidine, slow oscillations and an appreciable decrease in beta oscillation power are prominent during sedation. These dynamics most likely lead to BIS values that are typically in the unconscious range, even though the patient can be aroused by verbal commands or light shaking.
As we discuss in the “Changes in EEG Signatures with Aging” section, anesthetic-induced EEG oscillations change systematically with age. BIS appears to work poorly in older adults (>60), as patients in this age cohort tend to have lower amplitude oscillations, which the BIS algorithm can interpret as an awake state or state of unconsciousness. Similarly, the BIS algorithm is known to inaccurately reflect the anesthetic state of children because they generally have much more power across a broader range of frequency bands when appropriately anesthetized compared with adults in the 18 to 59 year age range. Hence, even though the children may be well anesthetized, the BIS algorithm provides numbers suggesting a state of sedation rather than unconsciousness.
Use of the BIS monitor has been proposed as a way to prevent intraoperative awareness, which is defined as the patient having explicit recall of events that transpired during the time that he or she was under general anesthesia. Use of the BIS monitor as a way to prevent intraoperative awareness was studied in the B-Aware Trial. This study compared patients at high risk for awareness who were randomly assigned to monitoring with BIS using a target range of 40 to 60 and patients monitored with the standard of care at that particular institution. The patients in the BIS group had a significantly lower incidence of awareness.
The findings from this study were called into question because of several design concerns, and for this reason the B-Unaware Trial was conducted. The B-Unaware Trial was a multicenter investigation that randomly assigned patients to either BIS monitoring or monitoring of the end-tidal anesthetic gas concentration to compare the two approaches for preventing awareness. The end-tidal gas concentration was maintained between 0.7 and 1.3 of the age-adjusted minimum alveolar concentration (MAC) of the anesthetic administered to the patient (discussed under End-Tidal Anesthetic Concentration). As in the B-Aware Trial, the objective in the BIS-monitored group was maintenance of the BIS value between 40 and 60. This study found no significant difference in the incidence of awareness among the patients monitored with BIS compared with those monitored with the end-tidal gas concentration. The authors interpreted these findings to mean that BIS monitoring was not more effective than the end-tidal anesthetic criterion in preventing awareness in patients receiving general anesthesia using volatile anesthetics. Several concerns were expressed about the findings of this study as well. The most notable were subject selection and whether the study had sufficient power to detect actual differences, had they been present.
As a follow-up to this study, the investigators in the B-Unaware Trail conducted the BIS or Anesthetic Gas to Reduce Explicit Recall (BAG-RECALL) trial, a second trial investigating whether BIS was superior to the end-tidal anesthetic gas concentration in preventing intraoperative awareness in a larger group of patients at high risk for awareness. In this trial, the investigators found that the BIS-guided protocol was not superior to the end-tidal anesthetic concentration-guided protocol in preventing awareness. Because the B-Unaware trial and the BAG-RECALL trial used inhaled anesthetics as the primary agents, their conclusions do not apply to patients receiving total intravenous anesthesia.
Preventing awareness under general anesthesia is a solvable problem if strategies used to monitor the brain states of patients use markers that relate directly rather than indirectly to the mechanisms through which the anesthetics act at specific receptors and neural circuits to alter level of arousal. Unlike the current EEG-based indices, these markers will differ with patient age and different anesthetics, (See the Unprocessed EEG and the Spectrogram section).
Patient Safety Index
The Patient Safety Index (PSI) is like the BIS index—a proprietary algorithm that assesses anesthetic state based on the EEG for patients receiving general anesthesia or sedation. The PSI was approved by the FDA in 2000 and was originally developed by Physiometrix (North Billerica, Massachusetts), and was ultimately acquired by Masimo (Irvine, California). The development of the PSI was the outgrowth of several years of research conducted by E. Roy John at the Brain Research Laboratory at the New York University School of Medicine. Like the BIS index, the PSI is also scaled between 0 and 100 ( Fig. 40.4 ). However, the PSI range to ensure that the patient is unconscious is between 25 and 50.

The original formulation of the PSI used an electrode montage that included occipital and frontal EEG leads to monitor the phenomenon of anteriorization as a marker of change in anesthetic state. Anteriorization is the forward shift of spectral power from the occipital area to the frontal area during loss of consciousness and the posterior shift of this power from the frontal areas to the occipital areas during the return of consciousness. The current formulation of the PSI uses a four-lead frontal EEG montage. In addition to displaying the PSI, this monitor also shows in real time the unprocessed EEG and its spectrogram from both the left and right sides of the head, the level of electromyographic activity, an artifact index, and the suppression ratio. The suppression ratio is a number between 0 and 100 that measures the fraction of time the EEG is in burst suppression. The monitor also allows the user to change the viewing screen among various combinations of the unprocessed EEG traces, the spectrogram, and the time series of the PSI values.
In head-to-head comparisons, the PSI correlates strongly with the BIS readings in terms of tracking a patient’s anesthetic state. This monitor has been less frequently studied in clinical investigations and has not received the same level of clinical use as the BIS monitor. In our experience, the PSI can also give ambiguous information for ketamine, nitrous oxide, dexmedetomidine, and pediatric patients.
Narcotrend
The Narcotrend, produced by MonitorTechnik (Bad Bramstedt, Germany), is an EEG-based device designed to monitor the anesthetic state in patients receiving general anesthesia or sedation. This monitor was developed at the University Medical School of Hanover, Germany, and has been approved by the FDA for use in the United States for patient care. Like the BIS and PSI, the Narcotrend uses a proprietary algorithm that converts the EEG into different states, denoted as A to F ( Table 40.1 ). Stage A corresponds to the patient being wide-awake, whereas stage F corresponds to increasing burst suppression down to an isoelectric state. The newer version of the Narcotrend monitor includes a Narcotrend Index, which is scaled between 0 and 100. In addition, the Narcotrend monitor displays the unprocessed EEG signal and its spectrogram. This monitor has also been validated with respect to the BIS index and separately. Its performance has been variable. Narcotrend has received less clinical use than BIS and PSI.
Narcotrend Stage | Narcotrend Index | |
---|---|---|
Awake | A B 0 | 95-100 90-94 |
Sedated | B 1 B 2 | 85-89 80-84 |
Light anesthesia | C 0 C 1 C 2 | 75-79 70-74 65-69 |
General anesthesia | D 0 D 1 D 2 | 57-64 47-56 37-46 |
General anesthesia with deep hypnosis | E 0 E 1 E 2 | 27-36 20-26 13-19 |
General anesthesia with increasing burst suppression | F 0 F 1 | 5-12 1-4 |
Entropy
The use of entropy to track the anesthetic state of patients is a relatively new monitoring approach. Entropy is a well-known concept in the physical sciences, mathematics, and information theory. Entropy measures the degree of disorder or the lack of synchrony or consistency in a system. Entropy-based analyses have been applied to the EEG and have been used to construct EEG-based indices meant to indicate the depth of anesthesia. The Entropy monitor was developed by Datex-Ohmeda, which is now part of GE Healthcare (Little Chalfont, United Kingdom). The algorithm in the GE device uses frequency domain analysis, combined with burst suppression to measure the entropy of the EEG in patients receiving anesthetic drugs. Unlike several of the other algorithms we have discussed, the entropy algorithm used in the GE entropy device is publicly available.
A readily apparent feature of the EEG, as patients proceed through deeper anesthetic states, is that the patterns become more regular and ordered (see Fig. 40.2 ). That is, we observe an apparent decrease in the entropy of the EEG signal. The Entropy monitor reports two entropy numbers to aid in interpreting this monitor’s EEG analysis ( Fig. 40.5 ). The first is the response entropy (RE) and the second is the state entropy (SE). The RE tracks the changes in the EEG power in the higher frequency range 0.8 to 47 Hz, whereas the SE tracks the changes in the EEG power in the lower frequency range of 0.8 to 32 Hz. The relative changes in the RE and the SE have been proposed as a way to distinguish between real brain state changes versus those that are due to muscle activity on the electromyogram. In general, electromyographic activity shows up in the higher frequency range tracked by the RE. As a patient becomes more profoundly unconscious, the RE declines faster than the SE, thereby making it possible to distinguish unconsciousness from movement artifacts. Monitoring entropy is consistent with changes with the BIS.

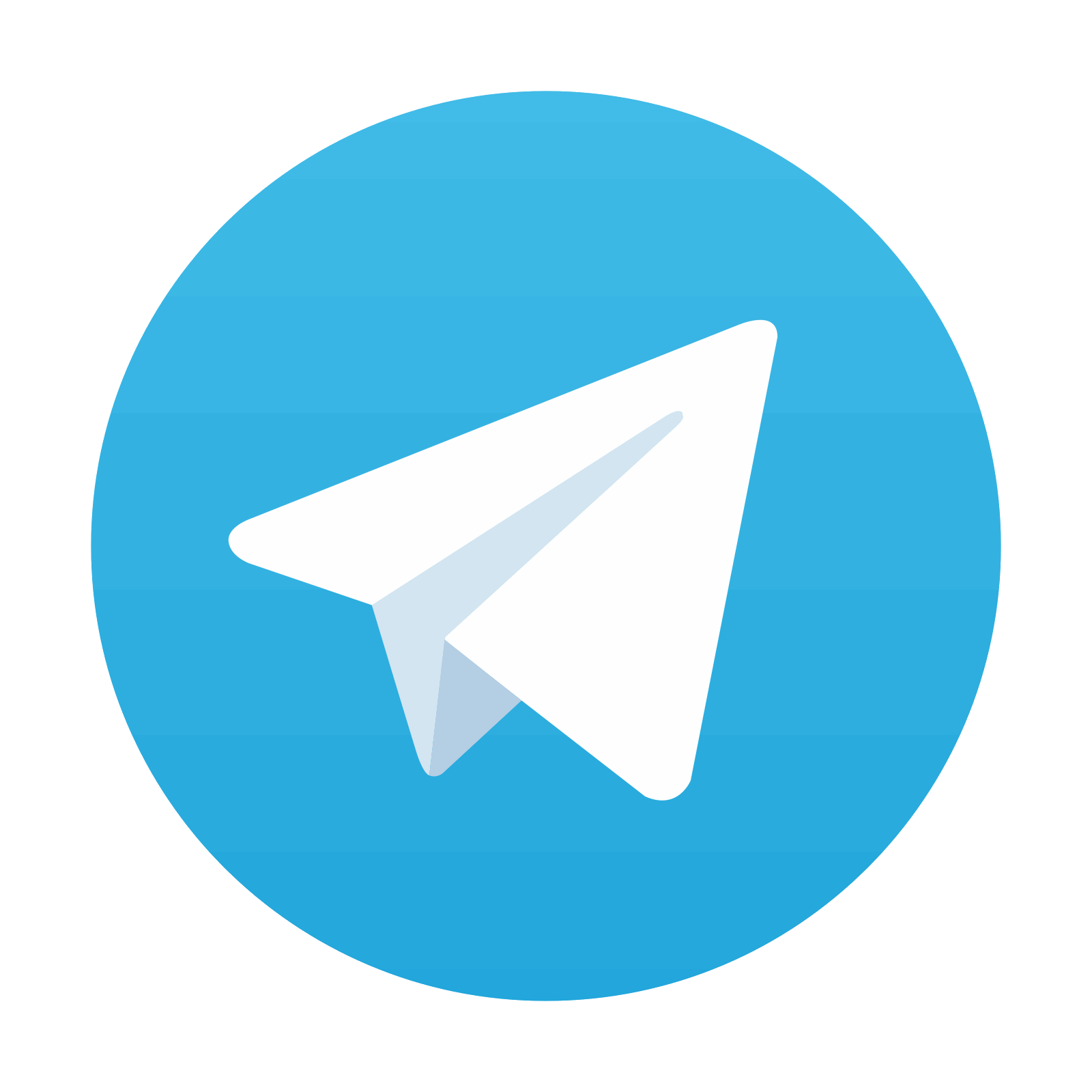
Stay updated, free articles. Join our Telegram channel

Full access? Get Clinical Tree
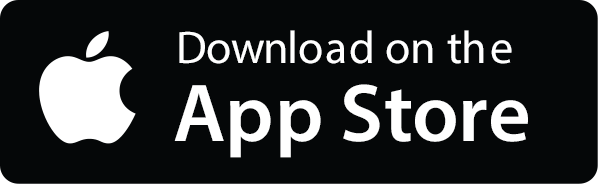
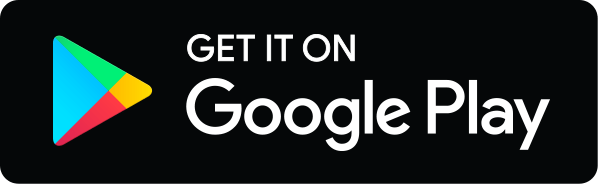
