Criteria
SIADH
CSW
DI
Serum sodium mmol/L
<135
<135
>145
Serum osmolarity mOsm/kg
<285
<285
>290
Urine osmolarity mOsm/kg
>200
>200
>200
Urine sodium mmol/L
>25
>25
–
Fluid balance
↑
↓
↑
Case 3: Spinal Cord Injury
A 22-year-old male suffered a complete spinal cord injury at C6 after diving into a shallow pool. The patient presents to the emergency room hypotensive and bradycardic. Other diagnostic studies are negative for further injury. Neurologically, the patient is insensate below C5 and quadriplegic corresponding to American Spinal Injury Association (ASIA) class A injury [30, 31]. However, he remains alert and oriented, maintaining spontaneous ventilation and appropriate oxygen saturation. Of note, all patients suffering traumatic insult severe enough to cause spinal cord injury and hypotension should be evaluated for other injuries; particularly those presenting an immediate threat to life such as intracerebral hemorrhage, tamponade from aortic dissection, pneumothorax, and intra-abdominal injury [30].
Management of acute spinal cord injury aims to prevent secondary injury from neuronal hypoperfusion with associated progression of neurologic deficits. Accordingly, the maintenance of adequate cardiac output and systemic blood pressure for optimal spinal cord perfusion is paramount to the critical care goals for patients with spinal cord injuries [32]. Neurogenic shock often complicates high thoracic and cervical spinal cord injuries. It is a form of distributive shock characterized by autonomic dysfunction with a sympathectomy causing low systemic vascular resistance, decreased venous return, and hypotension [30, 32]. Fluid administration to restore and maintain euvolemia is the primary goal of early resuscitation of distributive shock and often guided by central venous pressures (Fig. 44.1), but clinical evaluation of the patient’s intravascular volume status remains difficult [26].
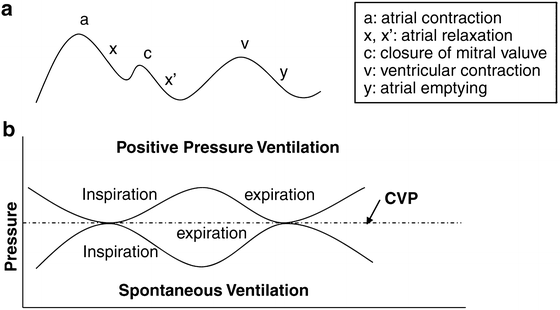
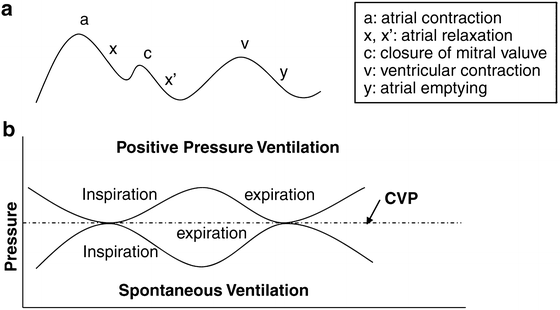
Fig. 44.1
Central venous pressure waveform (a). Variations in central venous pressure (CVP) with positive pressure ventilation versus spontaneous ventilation (b). The true value of CVP should be measured at end-expiration
Monitoring Fluid Status and Cardiac Output
The assessment of fluid status depends on the assimilation of multiple signs, symptoms, monitors, and laboratory values . This patient was managed with volume expansion and blood pressure augmentation to maintain a mean arterial pressure greater than 85 mmHg. Given the need for accurate assessment of intravascular volume and vasopressors to support the blood pressure, the patient had multiple indications for a central venous catheter. The typical CVP waveform is depicted and described in Fig. 44.1a. In order for the CVP to accurately estimate preload, intrathoracic pressure must equal atmospheric pressure, which occurs only at the end of expiration [33]. The CVP waveform varies with the cyclic changes in intrathoracic pressure during spontaneous and positive pressure ventilation [33, 34]. Figure 44.1b depicts the point along the CVP waveform where pressure should be recorded for clinical determination of transmural venous pressure.
Pulmonary Artery Catheters
Central venous pressure estimates preload by assuming a normal compliance within the left and right ventricles and pressure measurements that correlate with the left ventricular end diastolic volume or preload. The CVP misrepresents left ventricular filling pressures when ventricular or pulmonary artery compliance decreases or in the case of valvular disease or dysfunction. A pulmonary artery catheter (PAC) “wedged” with balloon occlusion to stop flow measures the pulmonary artery occlusion pressure (PAOP) which estimates left ventricular end-diastolic pressure through the static column of fluid in equilibrium with the left atrium. The PAOP is a better surrogate for preload as it is independent of right ventricular compliance and pulmonary hypertension or mitral stenosis [33, 35]. PACs also provide measures of cardiac output, mixed venous oxygen saturation, and estimates of systemic vascular resistance.
Returning to our patient with acute cervical spinal cord injury and hypotension , should he be refractory to volume resuscitation with progressive end-organ hypoperfusion and tissue hypoxia, he meets the definition of shock. Given traumatic injury in this patient, he could be suffering from hypovolemic, distributive, cardiogenic or obstructive shock secondary to hemorrhage, spinal cord injury, tamponade, or pneumothorax. Table 44.2 uses the common variables obtained from a PAC to describe the various hemodynamic features that differentiate shock states and allows for the appropriate treatment with fluids, blood products, inotropic support, or vasopressors.
Table 44.2
Four types of shock
Type of shock | Clinical example | CVP | SVR | PAOP | CO | SVO2 |
---|---|---|---|---|---|---|
Hypovolemic | Acute hemorrhage | ↓ | ↑ | ↓ | ↓ | ↓ |
Cardiogenic | Myocardial infarction, congestive heart failure | ↑ | ↑ | ↑ | ↓ | ↓ |
Obstructive | Pulmonary embolism, tamponade, tension, pneumothorax | ↑ | ↓ | ↓ | ↓ | ↓ |
Distributive | Sepsis, neurogenic shock, anaphylaxis | ↓ | ↓ | ↓ | ↑ | ↑↓ |
Preload Responsiveness
The first priority for resuscitation of distributive shock with spinal cord injury is volume expansion to increase preload and restore euvolemia. However, the value of CVP or PAOP adequate for optimal spinal cord perfusion varies with each patient and does not always correlate with fluid responsiveness [33]. The Frank Starling Curve describes the relationship that increases in stroke volume result from increased preload but only to a point beyond which inotropic or vasopressor support is necessary to augment blood pressure. Preload responsiveness is traditionally evaluated with a fluid challenge and observation of the resultant change in blood pressure or cardiac index. However, fluid boluses increase the risk of iatrogenic pulmonary edema, total body volume overload, and hyperchloremic metabolic acidosis. In the case of a patient with an indwelling arterial catheter receiving positive pressure ventilation, preload responsiveness can be assessed by observing the variation in pulse pressure or systolic blood pressure [34, 36].
Conclusion
Neurologic intensive care units staffed with nurses and physicians skilled and experienced in caring for patients with neurologic illness improve outcomes. The sophistication of neuromonitoring modalities in conjunction with hemodynamic monitors optimizes the management of critically ill patients and allows the intensive care team to monitor end organ perfusion continuously. In the NICU, the central nervous system is the tissue most at risk of ischemia. As mentioned earlier and in other chapters of this text, there are several monitors of cerebral perfusion and cerebral function available for continuous assessment during critical illness. Each type of monitor has advantages and disadvantages, complications and indications specific to different types of patients, and different classes of hemodynamic shock (Table 44.2). Outcomes literature supporting the use of continuous hemodynamic monitoring is lacking but often the risks are small and the patient morbidities high. This chapter reviewed the clinical role of several measures of cerebral perfusion and function as well as invasive and noninvasive hemodynamic monitors for patients in the ICU after neurologic injury.
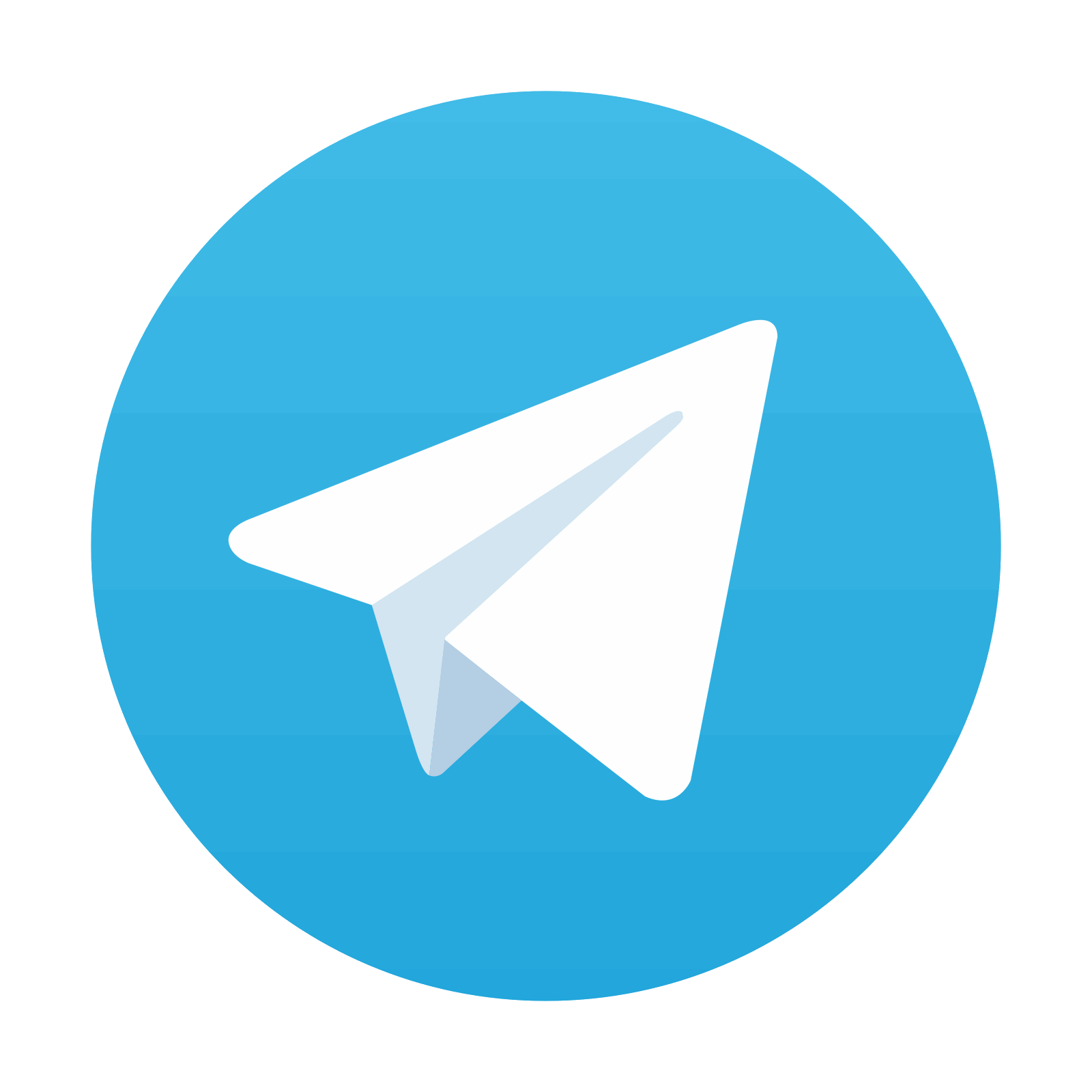
Stay updated, free articles. Join our Telegram channel

Full access? Get Clinical Tree
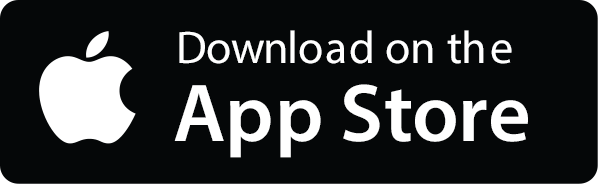
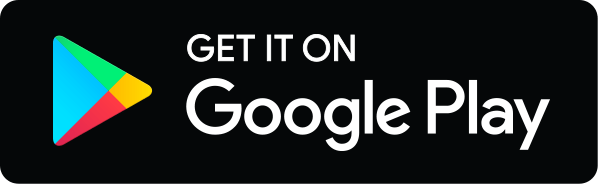