Clinical feature
Patients, n (%)
Muscular weakness
46 (78)
Back pain
43 (73)
Bladder dysfunction
42 (71)
Altered sensation
40 (68)
Leg pain or sciatica
33 (56)
Cutaneous stigmata
15 (25)
Foot deformities
13 (22)
Muscular atrophy
13 (22)
Leg length discrepancy
5 (8)
Spinal deformity
5 (8)
Fecal incontinence
4 (7)
Sexual dysfunction
2 (3)
As a result of the mechanical tethering of the spinal cord, there is a reduction in blood flow to the lower segments of the spinal cord, particularly in the conus. This leads to a derangement of oxidative metabolism in the grey matter of the cord with an impaired reduction/oxidation state in the cytochrome a, a3 system. Energy metabolism is therefore impaired. Spectrophotometry and Doppler flowmetry studies in humans and in experimental animal models show that this process can be reversed by surgical release of the cord resulting in improved blood flow and return to a more normal redox state [5, 7]. Neurologic improvement then parallels these changes. Surgical intervention is usually recommended for symptomatic patients, but it is still controversial whether to intervene on asymptomatic patients with evidence of tethered cord [5, 6]. Surgical treatment is aimed at the most complete untethering and release of the spinal cord as is possible utilizing microsurgical techniques. The goal is to relieve or stabilize symptoms and avoid further deterioration of neurologic function. Data show that the symptom most responsive to treatment is pain of the back or legs (up to 83 % improvement), followed by weakness (up to 69 %). Patients with a back and radicular pain history shorter that 1 year had better pain relief after untethering compared to those presenting with symptoms of longer duration [8].
Several studies have shown that up to 90 % of all patients brought to surgery will experience either improvement or stabilization of their complaints (Table 37.1) [4].
Case Presentation
A 47-year-old man presented to the neurosurgeon with a history of nonradiating pain in the midthoracic region of his back. In addition, he complained of dull, aching pain in the posterior aspect of the left thigh and in the left buttock. He had noticed progressive weakness in his left leg and foot along with numbness and tingling in the left leg. When asked, he also admitted to intermittent difficulty voiding his bladder. His medical history consisted of essential hypertension, social alcohol consumption, and a 4–5 MET (metabolic equivalent of task) exercise tolerance. He was taking lisinopril for his blood pressure with good control. Physical examination revealed a decrease in sensation to touch in the left leg along the anterolateral aspect including the dorsum of the foot and great toe. His muscle strength was 5/5 in the arms and right leg, but was 3–4/5 in the left foot dorsi- and plantar flexion. Radiographic studies consisted of X-rays of the thoracic and lumbosacral spine as well as full spine MRI. The MRI showed a cystic lesion in the thoracic spine at the T6–7 level, and the appearance of a short and thickened filum terminale (Fig. 37.1). He was scheduled for a thoracic laminectomy and cyst removal and a lumbar exploration and untethering of the spinal cord.
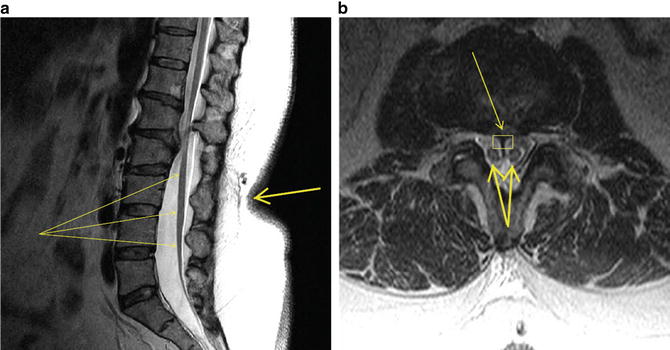
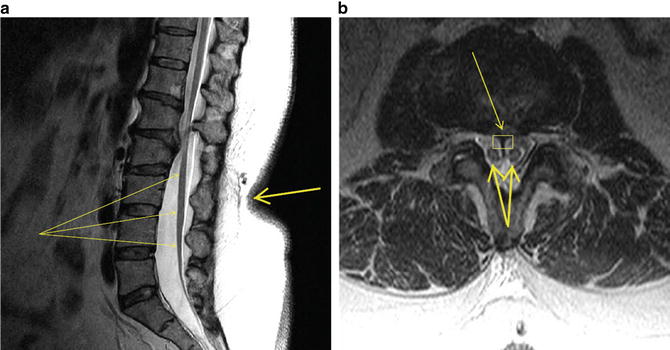
Fig. 37.1
MRI of lumbosacral spine. (a) Sagittal view. Note thickened filum terminale indistinguishable from low-lying conus medullaris (fine arrows) and adherent to posterior elements in caudal canal. Also note skin indentation on back with hair growth (thick arrow), which is pathognomonic of spinal dysraphism. (b) Transverse view. Note septum (fine arrow and rectangle) bifurcating the conus medullaris (thick arrows)
Monitoring Modalities
Given the location of the spinal cyst and the proposed untethering procedure, neurologic structures at risk include the thoracic spinal cord (both corticospinal tracts and posterior columns) and the nerves of the cauda equina. These structures may be injured through various mechanisms including vascular compromise (surgical interruption, vasospasm, arterial hypotension, anemia, hypoxemia), compression from surgical retractors, overheating from electrocautery, or traction from improper positioning or surgical manipulation [9]. Paramount during surgery is the need to preserve motor and sensory function of the lower extremities as well as bowel, bladder, and sexual function. This necessitates the identification and protection of multiple nerve roots in the cauda prior to surgical resection of the filum terminale or any other fibrous adhesions causing the tethering phenomenon, as well as protection of the motor and sensory tracts. Mapping techniques are useful in identifying and sparing functional neural structures. They are also helpful for identifying nonfunctional neural tissue to allow for complete untethering. Due to distorted anatomy in some patients, identification of neural structures based on anatomy and visual appearance only could be challenging. On the other hand, some patients have lost function of neural structures that can only be identified as nonfunctional with intraoperative neurophysiologic studies. Intraoperative neurophysiologic monitoring has also been used in a recent study by Jackson et al. [10] to confirm functional level in patients 18 months to 5 years old presenting for untethering after having fetal repair of myelomeningocele .
During the procedure, two modalities will be used to meet the objectives. First, mapping will identify and separate functional neural elements from nonfunctional fibrous bands and the filum. Second, monitoring will continuously assess the functional integrity of neural tracts. In addition, the surgeon will benefit from immediate warning of impending damage when contacting any neural structures in the surgical field with the expectation that changes can then be made to minimize or avoid permanent injury. Neurophysiologic monitoring is needed not only to predict but also to prevent nerve injury. Therefore, monitoring modalities available to achieve this include posterior tibial and median nerve somatosensory-evoked potentials (SSEPs), pudendal and individual spinal nerve root sensory-evoked potentials, transcranial motor-evoked potentials (MEPs), spontaneous and evoked electromyography (EMG) of musculature in the myotomes from L2 to S4, the bulbocavernosus reflex (BCR), and pressure urometry.
Anesthetic Management
To optimize outcome, the anesthetic technique was tailored to minimize deleterious effects on the monitoring modalities employed (SSEPs, MEPs, EMG), allowing the best feedback to the surgeon regarding impending neurologic injury. A total intravenous anesthesia (TIVA) technique was employed with judicious use of neuromuscular blockade. The patient was premedicated with 2 mg intravenous (IV) midazolam. Electrocardiogram, noninvasive blood pressure, bispectral index analysis (EEG, BIS), and pulse oximeter probes were attached, and the patient was preoxygenated with 10 L O2 by mask for 3 min. Anesthesia was induced with 1.5–2.0 mg/kg propofol and 15 μg sufentanil IV. After confirmation of the ability to provide ventilation by face mask, 50 mg rocuronium was given IV. When adequate muscle relaxation was confirmed, the trachea was intubated with a 8.0-mm ID endotracheal tube and secured at 23 cm depth. No additional neuromuscular blocking agents were administered for the duration of the case. Ketamine , 1 mg/kg, was given intravenously. For maintenance of anesthesia, an intravenous infusion was begun using a mixture consisting of 10 mg propofol and 0.5 mg ketamine per cc for hypnosis and infused at a rate between 100 and 300 μg/kg/min of the propofol. Sufentanil was used for analgesia. An additional 45 μg was given IV bolus (titrated) to achieve a loading dose of 1 μg/kg as tolerated while maintaining mean arterial blood pressure (MABP) of greater than 70 mmHg, and a continuous infusion was begun at a rate of 0.3 μg/kg/h. The propofol/ketamine and sufentanil infusions were adjusted during the case to maintain the bispectral index at 40–60 and the MABP between 70 and 90 mmHg. A radial artery cannula was placed to continuously monitor blood pressure and the patient was placed in the prone position with both arms tucked to the sides for surgery after placement of the intraoperative neuromonitoring (IONM) needles, grounding pad, and protective pads.
Intraoperative Neuromonitoring
Pursuant to the goals of mapping, monitoring, and warning, as stated above, several authors have advocated a multimodality approach to monitoring the neural structures at risk [11–21]. In particular, Krassioukov et al. [14] studied a group of 61 patients undergoing complex lumbosacral procedures; among them were 15 cases of tethered cord. All patients were monitored with a combination of evoked and spontaneous EMG and posterior tibial nerve SSEPs . Of the three patients who awoke with new neurologic deficits, only one had a significant change in SSEPs. The presence or absence of responses during stimulated EMG resulted in the alteration of the course of the surgical procedure in 24 cases (42 %). Regarding the efficacy of multimodality monitoring, Gunnarsson et al. [15] performed a retrospective analysis of 213 cases of thoracolumbar surgery, three of which were for tethered cord, using a combination of EMG and SSEPs . In their series, 14 patients had new neurologic deficits all of which had significant EMG activation, but only four had a change in SSEPs considered significant. In their study, EMG had a sensitivity of 100 %, and SSEPs had a specificity of 94.5 %. This reinforced the use of more than one monitoring technique. In a series of 44 adult patients undergoing surgery for tethered cord, Paradiso et al. [16] found that the combined use of SSEPs and EMG limited neurologic morbidity. They had one patient with a transient and one patient with a permanent neurologic deficit postoperatively. In one patient, a significant change in SSEP monitoring resulted in a change in surgical strategy. Their calculated measures of efficacy showed a sensitivity of EMG of 100 % and a specificity of SSEPs of 100 %—very similar to the Gunnarsson study. Beyazova et al. [22] recommended the inclusion of direct stimulation of nerve roots since it produced a positive response that differentiated neural tissue from nonfunctional connective tissue in three of ten patients while SSEPs or MEPs had not changed throughout the procedure. Sala et al. [23] recently confirmed that multimodal intraoperative neuromonitoring techniques, particularly the use of MEPs that includes anal sphincter and BCR, reduced morbidity in a series of 47 patients. This study found that 12 % of patients presented unexpected muscle responses when tissue considered as nonfunctional was stimulated [19]. Garg et al. [5] retrospectively studied 24 adult patients who presented for tethered cord procedures. Neurophysiological monitoring was not conducted since it was not available. In one patient, weakness increased due to the accidental section of a functional neural structure. This situation could have been prevented by the use of intraoperative neuromonitoring, as it has been prevented in other series of patients. Sala et al. [23] (64 patients) and Valentini et al. [21] (149 patients) reported the use of multimodal neuromonitoring approach in their institutions for complex spinal cord untethering and occult spinal dysraphism, respectively. Both concluded that the combination of mapping and other monitoring techniques such as EMG, MEPs, and BCR improved the level of untethering while decreasing morbidity [20, 21]. For rare dysraphic malformations like retained medullary cord, a form of severe tethering lesion, the use of mapping was considered indispensable since anatomical appearance alone will not be sufficient to resect the retained cord safely [23].
In this patient, the modalities employed were SSEPs , MEPs, stimulated and spontaneous EMG, the bulbocavernosus reflex, and pressure urometry. Posterior tibial and median nerve SSEPs were chosen to assess the functional integrity of the posterior columns below the level of surgery and to use the arms as a control above the level of surgery as well as to monitor for positioning injury. To monitor the stimulated and spontaneous EMG, specific muscles were chosen to cover the myotomes from L2 to S4 as inclusively as possible given the amount of channels available. Also, bladder pressure urometry, through the use of a urinary catheter attached to a pressure transducer system, allowed monitoring of the bladder detrusor muscle function.
Somatosensory-Evoked Potentials
Posterior tibial nerve SSEP monitoring allows continual functional assessment of the posterior columns with sensory input from the dermatomes of L4 through S1 with the primary contribution from L5 and S1. Given the dual surgical approaches in the thoracic spine and the lumbosacral spine, posterior tibial SSEPs provided essential information about injury to dorsal spinal cord structures, especially in the thoracic region, and sensory nerves in the lower levels. In this situation, electrodes were placed in the popliteal fossa to record nerve action potentials (thus insuring adequacy of stimulus), over the cervical spine to record subcortical responses, and in the scalp to record cortical responses. Subcortical cervical responses help to differentiate whether changes in the cortical response are due to anesthetic effects or from other causes since, as mentioned in an earlier chapter, these are resistant to the effects of anesthetic drugs.
The median nerve is formed from fibers from the roots of C6–T1 with occasional contributions from C5. Median nerve SSEPs therefore monitor the functional integrity of the posterior columns above the thoracic level of this patient’s cyst. Electrodes are placed over the brachial plexus, cervical spine, and scalp for the same reasons as mentioned previously for posterior tibial nerve SSEPs. These will not be affected by any surgical maneuvers in the thoracic or lumbosacral spine and can therefore aid in the differential diagnosis of changes, being used as a control compared with the posterior tibial responses. They can also be used to detect and prevent impending positional injury to the brachial plexus or median nerve—an issue when the patient is prone.
While posterior tibial SSEPs will provide information from the L4 to S1 level, the entire cauda is at risk during untethering. To enhance input to the surgical team, somatosensory-evoked potentials (SSEPs) from the levels S2 to S4 can be obtained from stimulation of the pudendal nerve. This is accomplished by stimulating the nerve on the dorsum of the penis or the clitoris. However, since the responses obtained are of low amplitude and require a high number of repetitions to generate repeatable responses, thus making reliable acquisition difficult, the utility of this technique has been questioned and was not used on this patient [13, 17, 18, 24].
Transcranial Motor-Evoked Potentials
The surgical approach to the thoracic spine cyst places the corticospinal tracts at risk for injury from multiple factors including physiologic derangements such as hypotension. SSEP monitoring alone will not suffice to monitor for this injury as SSEPs are not a measure of motor tract function and are mediated by anatomical structures with a separate vascular supply. Therefore, MEP monitoring is essential. Monitoring the functional integrity of the motor tracts in tethered cord surgery is important because the presence of the muscle response at closure correlates with preserved muscle control and the absence of postoperative motor deficits [18]. Intraoperative motor-evoked potential improvement has been reported after complete and successful untethering. This was associated with clinical improvement in the immediate postoperative period [25]. Responses were monitored in the upper and lower extremities for the same reasons as stated for SSEPs: to be used as control responses and to monitor for positional injuries. Motor-evoked potentials were monitored using transcranial electrical stimulation of the motor cortex and recording the compound muscle action potential (CMAP) generated from the abductor pollucis brevis (APB) , abductor hallucis (AH), and tibialis anterior (TA) muscles. For the lumbar approach to the untethering of the spinal cord, D wave monitoring of the MEPs would not be helpful since these are not recordable below the level of the conus medullaris. Electrodes placed in the external anal sphincter will provide feedback on the motor elements of the pudendal nerve (S2–S4).
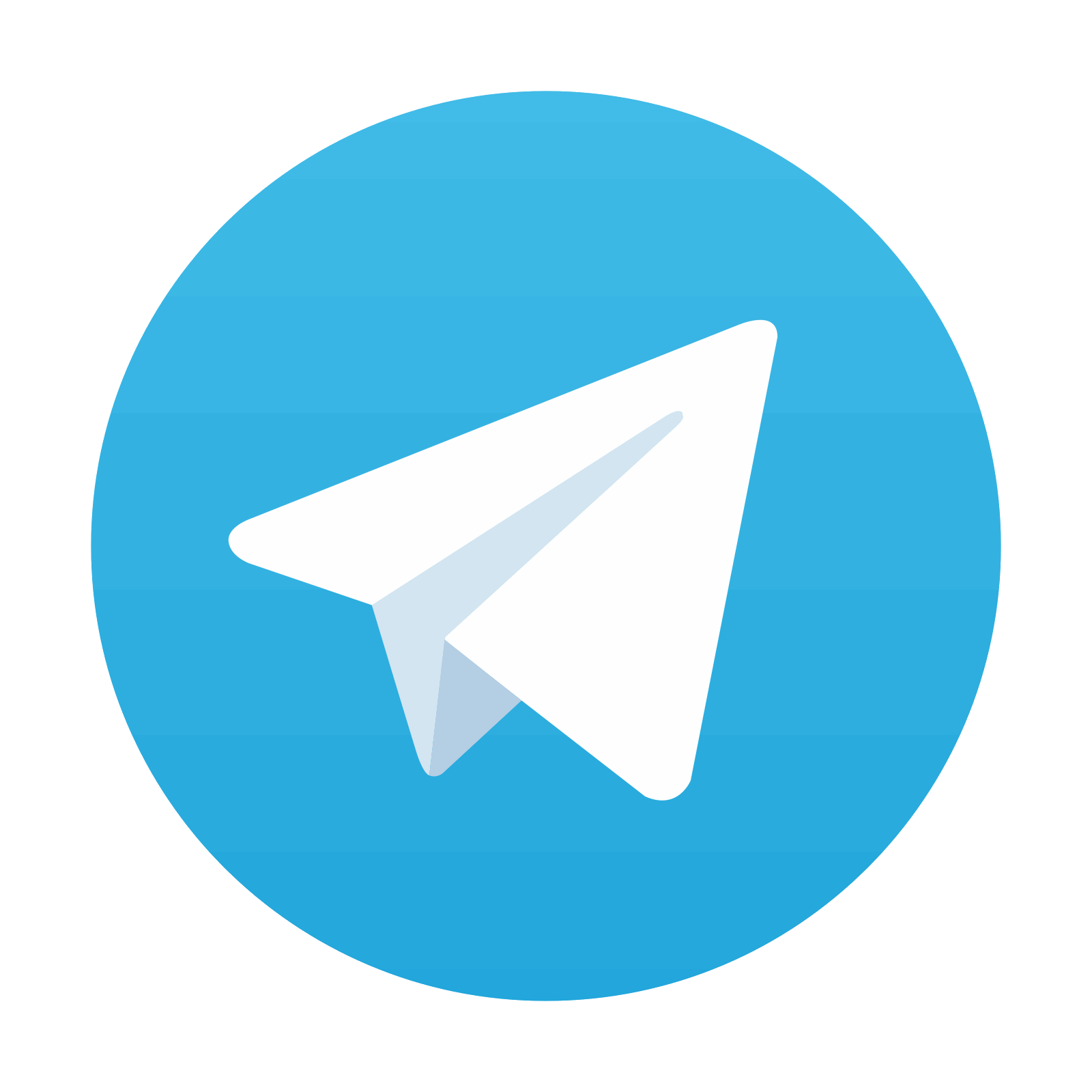
Stay updated, free articles. Join our Telegram channel

Full access? Get Clinical Tree
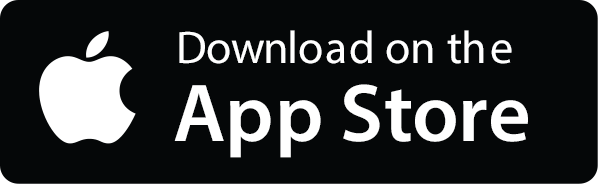
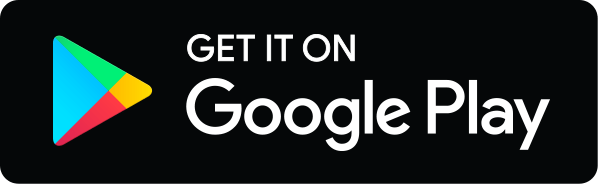