9 Management of Cervical Injuries David O. Okonkwo, Rod J. Oskouian Jr., and Christopher I. Shaffrey Cervical spinal injury occurs in 4 to 10% of patients who sustain traumatic brain injuries due to blunt force trauma. Cervical spinal injury should be suspected in any trauma patient with loss of consciousness. All of the following patients should undergo a radiographic search for a cervical spinal injury: patients who present awake with neck pain or tenderness, patients with a neurologic deficit, patients who have sustained a traumatic brain injury, patients with a distracting injury that clouds the cervical spine examination, and patients who present following a sufficient mechanism of injury. This chapter discusses the presentation, classification, radiographic characteristics, and management options for cervical spinal injuries, grouped by injuries of the cranio-cervical junction and injuries of the subaxial cervical spine. Occipital condyle fractures were first described by Bell1 in 1817, and the association of occipital condyle fractures and severe head trauma was initially drawn in autopsy series, with an incidence as high as 4% in fatal head injuries.2 However, the clinical diagnosis and management of occipital condyle fractures are principally phenomena of the computed tomography (CT) era. Conventional cervical spine plain films miss between 50 and 93% of occipital condyle fractures,3,4 which are the most common cervical spine fracture missed by radiology residents.5 With the advent of routine CT scanning in high-energy blunt trauma, the spectrum of this disease has become much better elucidated. Occipital condyle fractures should be suspected in trauma patients with lower cranial nerve palsies, upper cervical spine mobility restriction, or persistent neck pain. The 12th cranial nerve is most commonly involved, due to the proximity of the hypoglossal canal, but palsies of cranial nerves VI, IX, and X also occur with occipital condyle fractures. Cranial nerve palsies resulting from occipital condyle fractures may occur acutely or in a delayed fashion. Prognosis is more favorable with cranial nerve palsies with delayed presentation; acute cranial nerve palsies rarely heal completely. Brainstem compression from displaced fracture fragments, torticollis, and retropharyngeal hematoma with acute respiratory distress have also been reported following occipital condyle fractures.1,6,7 These fractures can also precipitate rotatory subluxation. Full clinical assessment is often difficult because many patients have concomitant traumatic brain injury and altered level of consciousness. Brainstem compression from displaced occipital condyle fractures is rare, reported only five times in the literature, but surgical decompression is indicated. Anderson and Montesano8 first classified occipital condyle fractures in 1988 based on the vector of force precipitating the injury (Table 9.1 and Fig. 9.1): Fig. 9.1 Occipital condyle fractures. (A) Axial computed tomography (CT) image of Anderson and Montesano type II and Tuli type 1 occipital condyle fracture. Note the associated basilar skull fracture. (B) Axial CT and (C) T2-weighted axial magnetic resonance imaging (MRI) of an Anderson and Montesano type III and Tuli type 2A occipital condyle fracture. An intact transverse ligament (arrow) has avulsed the tip of the right occipital condyle. Anderson and Montesano concluded that type I and II fractures were stable, whereas type III fractures were unstable and require rigid immobilization. In 1997, Tuli et al9 proposed an alternative classification scheme for occipital condyle fractures (Table 9.1): In this context, craniocervical junction instability is determined via evidence from MRI demonstrating ligamentous injury or CT with coronal reconstructions revealing joint misalignment in the occiput-C1-C2 complex. Tuli et al9 proposed that type 1 fractures be managed without intervention, type 2A fractures be managed with C-collar immobilization, and type 2B fractures require halo traction or surgical immobilization. Contemporary reports advise C-collar immobilization for both type 1 and 2A fractures.10,11 At the conclusion of the period of immobilization, flexion-extension views should be obtained to confirm the absence of craniocervical junction instability. The recognition of atlanto-occipital dislocation, as with occipital condyle fractures, has increased with the advent of routine CT evaluation of the cervical spine in the trauma survey. Atlanto-occipital (AO) dislocations are high-velocity, high-force injuries. Typically, AO dislocation results from distraction with extreme hyperextension and rupture of the tectorial membrane (the cranial extension of the posterior longitudinal ligament). Atlanto-occipital dislocation accounts for 8 to 35% of fatalities from motor vehicle accidents and 10% of cervical spine injury fatalities.12 Classically, AO dislocation was considered fatal. Survival is now common, perhaps related to increased sensitivity of detection during the trauma survey. Twenty percent of survivors have normal neurology; 10% have lower cranial nerve palsies, 34% hemiparesis or hemiplegia, and 38% quadriparesis or quadriplegia.13 Death from AO dislocation results most commonly from respiratory depression secondary to neurogenic shock.2 Patients surviving AO dislocation frequently have neurologic deficits secondary to injuries other than the AO dislocation, such as traumatic brain injury (24%), carotid or vertebral artery injury, or brachial plexus injury.14,15 Both type I and type II odontoid fractures increase the risk for AO dislocation due to weakening or rupture of the alar ligament, apical ligaments, or tectorial membrane. The more classic association is with type I odontoid fractures; in fact, any type I odontoid fracture diagnosis should prompt an evaluation for AO dislocation. Fig. 9.2 Three methods for measuring atlanto-occipital dislocation are depicted. (A) The Power’s ratio is the ratio of the distance from the tip of the basion to the posterior arch of C1 (B-C) divided by the distance of from the tip of the opisthion to the tip of the odontoid (O-O). Ratios greater than 1 are consistent with the diagnosis of anterior atlanto-occipital dislocation. (B) For Lee’s lines, two intersecting lines are drawn, one from the tip of the basion to the anterior aspect of the posterior ring of C2 and a second from the tip of the opisthion to the posteroinferior aspect of the C2 body. The first line should pass across the superior-posterior aspect of the odontoid process, and the second should pass just anterior to the posterior portion of the ring of C1. (C) Harris’s measurements involve two calculations: the basion-dens interval (BDI), the distance between the inferior and posterior tip of the basion and the superior tip of the odontoid; and the basion-posterior axial interval (BAI), the distance from the posterior axial line to the inferior and posterior tip of the basion. For both the BDI and BAI, a value greater than 12 mm is indicative of an anterior atlanto-occipital dislocation. The diagnosis of AO dislocation is frequently delayed or missed in patients who present neurologically intact or with incomplete neurologic syndromes.13 The radiographic evaluation of AO dislocation involves the calculation of Power’s ratio, Lee’s line measurements, or Harris’s measurements. Power’s ratio is the distance from the tip of the basion to the posterior arch of C1 (B-C) divided by the distance from the tip of the opisthion to the tip of the odontoid (O-O) (Fig. 9.2A). Ratios greater than 1 suggest anterior AO dislocation. Ratios less than 1 are normal unless a posterior AO dislocation is present. Caution must be used employing Power’s ratio in the setting of congenital anomalies of the craniocervical junction or with C1 fractures such as Jefferson fractures. The X-line method, or Lee’s lines, involves assessment of relationships of bony elements at the craniocervical junction. Two intersecting lines are drawn, one from the tip of the basion to the anterior aspect of the posterior ring of C2 and a second from the tip of the opisthion to the posteroinferior aspect of the C2 body (Fig. 9.2B). The first line should pass across the superior-posterior aspect of the odontoid process, and the second should pass just anterior to the posterior portion of the ring of C1. Lee’s method sought to improve the sensitivity of plain film radiographs in the diagnosis of AO dislocation, because proper identification of the midpoint of the posterior arch of C1 for calculation of Power’s ratio can be difficult on lateral x-rays. Harris’s measurements, or the rule of 12s, involve two calculations: the basion-dens interval (BDI) and the basion-posterior axial interval (BAI) (Fig. 9.2C). The BDI is the distance between the inferior and posterior tip of the basion and the superior tip of the odontoid. To calculate the BAI, a vertical line is drawn along the posterior aspect of the odontoid, extending above the level of the foramen magnum (the posterior axial line). A perpendicular line is drawn from this posterior axial line to the inferior and posterior tip of the basion, and the distance is measured. For both the BDI and BAI, a value greater than 12 mm is indicative of an anterior atlanto-occipital dislocation (hence, the rule of 12s). With modern continuous slice acquisition CT and three-dimensional reconstructions of the spine and head, Power’s ratio, Lee’s method, or Harris’s measurements may be employed with reliability and accuracy for evaluation for AO dislocation.16 Spontaneous reduction of AO dislocation can occur such that any of the aforementioned measurement techniques no longer detect it. An increased distance between the posterior elements of C1 and C2 may be the only clue on CT, with subsequent magnetic resonance (MR) evaluation revealing substantial ligamentous injury at the craniocervical junction. Retropharyngeal hematomas occur in all AO dislocations and, if noted on CT or plain film radiographs, should prompt MR evaluation of the cervical spine (Fig. 9.3). Traynelis and colleagues17 classified three types of AO dislocations (Table 9.2): Note that Power’s ratio may be falsely negative in detecting type III (posterior) AO dislocations. Fig. 9.3 Radiographic images of a patient with anterior atlanto-occipital dislocation. (A) Sagittal CT reconstruction at presentation demonstrates a clear dissociation of bony elements at the craniocervical junction and a Power’s ratio greater than 1. (B) Sagittal T2-weighted MRI obtained hours later demonstrates interval decrease in the basion-dens interval, indicating spontaneous reduction of the atlanto-occipital (AO) dislocation. However, associated disruption in the posterior ligamentous complex is seen. (C) Axial T2-weighted MRI reveals an epidural hematoma adjacent to the upper cervical spinal cord. The patient had a left hemiparesis. Patients with AO dislocation should be treated to avoid progression of neurologic deficits due to the highly unstable nature of these fractures. Occipital-cervical fusion is the treatment of choice, although halo ring-vest orthosis is an alternative for patients where occipital-cervical fusion is contraindicated. Early surgical intervention does not increase risk of neurologic worsening or late instability. In most case of AO dislocations, traction results in longitudinal distraction. It would therefore not be indicated in patients whose spinal column was in good alignment and whose primary pathology was longitudinal distraction (type II). For type II AO dislocations, traction is controversial and is associated with a 10% risk of neurologic deterioration because it could increase the distraction distance.18 For type I and III AO dislocations, if the spine is not in alignment, then gentle traction at the bedside but without weights can be warranted, especially if there is a neurologic deficit present to realign the spine. Most often just by changing the head position the alignment can be normalized. Table 9.3 Classification of Isolated C1 (Atlas) Fractures
Craniocervical Junction
Occipital Condyle Fractures
Atlanto-Occipital Dislocation
Type I: Fractures of a single arch |
Type II: Burst fracture (classic Jefferson fracture with a four-point burst fracture of the ring of C1) |
Type III: Lateral mass fractures of the atlas |
Source: From Landells CD, Van Peteghem PK. Fractures of the atlas: classification, treatment and morbidity. Spine 1988;13:450–452.
Isolated C1 Fractures
Isolated fractures of C1 rarely cause neurologic sequelae. Improper management of these fractures, however, can lead to delayed neurologic deficit. Atlas fractures may involve the lateral mass (13–37%), the anterior or posterior ring (31–45%), or a Jefferson fracture of both the anterior and posterior ring (37–51%) (Table 9.3).
The critical clinical feature to discern in isolated C1 fractures is integrity of the transverse ligament. Integrity of the transverse ligament may be assessed on anteroposterior (AP) or open-mouth odontoid plain film radiography or coronal reconstruction CT by measuring the lateral mass displacement. If the cumulative displacement of the lateral masses is ≥ 7 mm, then the transverse ligament is considered incompetent (the rule of Spence).19 Panjabi’s group20 found that an atlanto-dens interval (ADI) greater than 3.5 mm on flexion radiographs was the most reliable predictor of transverse ligament disruption. Transverse ligament disruption can also be diagnosed on magnetic resonance imaging (MRI).
There is no class I or class II evidence regarding treatment for isolated C1 fractures. Fractures of the C1 lateral mass, which are unlikely to be associated with disruption of the transverse ligament or an increase in the ADI, can be treated with external cervical immobilization. There is no evidence to support the use of a halo orthosis over rigid cervical collars. External immobilization for 8 to 12 weeks is sufficient, and healing should be assessed with flexion-extension plain film radiographs to exclude pseudarthrosis and craniocervical instability. Some clinicians advocate use of a halo orthosis for comminuted lateral mass fractures to increase fusion rates over rigid cervical collar immobilization, but this decision should be multifactorial and take into consideration the overall medical condition, as older patients with significant medical comorbidities do not tolerate external immobilization well.
Fractures of either the anterior or posterior arch should be assessed for concomitant instability or disruption of the transverse ligament. Instability is characterized by an ADI > 3.5 mm, lateral mass displacement > 7 mm, or MR evidence of transverse ligament avulsion or disruption. Isolated C1 ring fractures of either the anterior or posterior arch may be treated with cervical collar immobilization, sternal occipital mandibular immobilization (SOMI) brace, or halo for up to 3 months. There is no evidence to support the use of one option over the others.21,22
Fractures of both the anterior and posterior arch carry special treatment considerations. Sir Geoffrey Jefferson23 in 1920 initially described his fracture of the C1 ring as bilateral fractures of the junction of the lateral masses and the anterior and posterior arches. This fracture of both the anterior and posterior arch is also referred to as a C1 burst fracture. Treatment of C1 burst fractures is dictated by the presence or absence of instability, defined as either an ADI > 3.5 mm or lateral mass displacement > 7 mm on CT or disruption of the transverse ligament on MRI. A C1 burst fracture with an intact transverse ligament may be treated with external cervical immobilization (rigid cervical collar or halo vest orthosis) for up to 3 months. An unstable burst fracture may be treated with either a halo device for 3 months or C1-C2 internal fixation and fusion, with postoperative immobilization dictated by the fusion procedure chosen.
Surgical options for C1-C2 fusion for unstable C1 burst fractures include the Harms technique (C1 lateral mass and the C2 pedicle, pars interarticularis or translaminar fixation with cervical polyaxial screws and rods),24 C1-C2 transarticular screw fixation,25,26 or a Brooks, Gallie, or Sonntag posterior wiring fusion.
Dickman and Sonntag27 reported their long-term results for C1-C2 transarticular screw fixation and noted a substantially higher fusion rate for C1-C2 transarticular screws over Brooks, Gallie, or Sonntag posterior wiring techniques.27 The Harms technique of C1-C2 fixation was described in 2001, but large case series with long-term data are not yet available.24 However, the Harms C1-C2 fusion has biomechanical advantages over the Magerl technique of transarticular screw fixation, is less likely to injure the vertebral artery, does not require structural bone graft or wiring, and is less likely to be contraindicated by anatomy. In addition, Harms and Melcher24 reported a 100% fusion rate in their initial series of 37 patients. Postoperative external cervical immobilization is not necessary following a Harms C1-C2 fusion, in contradistinction to Sonntag’s original description of his modification of the posterior wiring technique, further limiting postoperative morbidity of the Harms C1-C2 fusion.
More recently, Harms described a transoral reduction and C1 osteosynthesis technique for young patients as an alternative function-preserving option for unstable Jefferson fractures.28
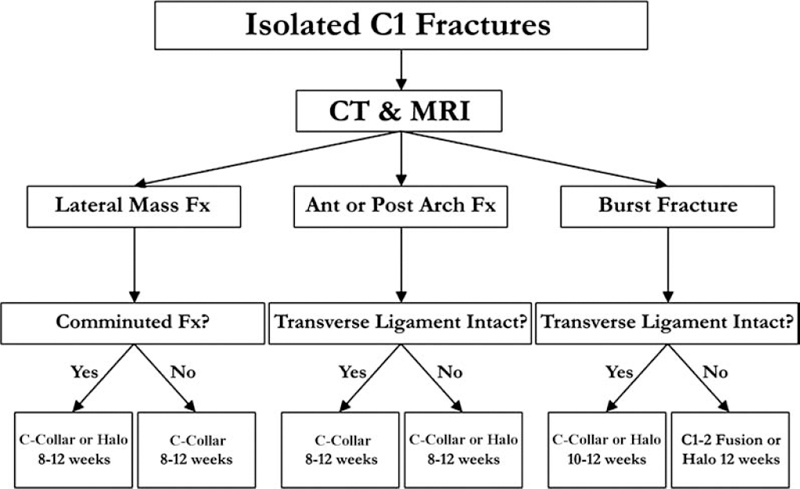
Fig. 9.4 Treatment algorithm for management of isolated fractures of the atlas.
Figure 9.4 depicts an evidence-based treatment algorithm for management of isolated fractures of the atlas. Treatment choice must be weighed in the context of other traumatic injuries, medical comorbidities, and patient preference.
Isolated C2 Fractures
Approximately 20% of all cervical spine trauma cases involve C2. The spectrum of C2 fractures includes hangman’s fractures (bilateral traumatic spondylolisthesis of the atlas), odontoid fractures, and miscellaneous fractures of the C2 vertebral body. The incidence of neurologic deficit in surviving patients is low (7.5% in one series), likely due to the larger spinal canal diameter at this level.29 C2 fractures are common in the elderly and carry a significant in-hospital mortality rate, perhaps as high as 25%.30,31 However, recent evidence suggests that surgical management of C2 fractures among the elderly is associated with acceptable rates of morbidity and lower mortality for this group of fractures, arguing for more routine use of surgical management in appropriate patients in this population.32
Odontoid Fractures
Odontoid fractures are the most common traumatic injuries of the axis and account for 7 to 14% of all cervical spine injuries. The most common symptom is high cervical pain, as neurologic deficits are infrequent. However, spinal cord compression may result from subluxation of the fracture dens, and maintenance of spinal alignment must be a primary goal of the evaluation and management of these fractures.
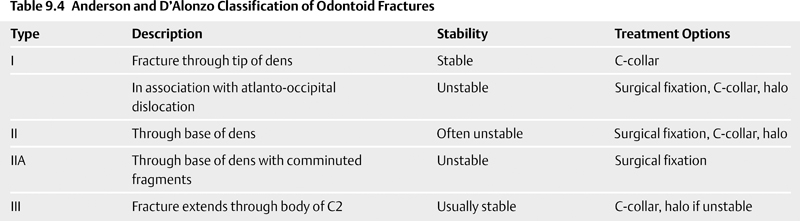
Anderson and D’Alonzo33 proposed a classification system for odontoid fractures in 1974 that is still in use today (Table 9.4). Type I fractures are oblique fractures through the tip of the odontoid process. Type I fractures are rare, representing less than 1% of odontoid fractures, but may occur during atlanto-occipital dislocation.34,35 Type I fractures may lead to os odontoideum due to resorption of the lower dens from avascular necrosis. Type II fractures are those occurring at the base of the odontoid process at the junction with the body of C2. Type III odontoid fractures extend through the dens and into the body of C2. Anderson and D’Alonzo themselves stated that type III odontoid fractures should be considered fractures of the body of C2. In fact, later authors argued that type III odontoid fractures should be grouped with other, miscellaneous fractures of C2. Nevertheless, the designation of type III odontoid fracture remains in clinical use today.
Mark Hadley and colleagues36 modified the Anderson and D’Alonzo classification scheme to distinguish comminuted fractures with free fragments of the base of the odontoid. These fractures are termed type IIA fractures. Although rare (only 5% of the Hadley series of type II fractures), the type IIA fracture is unstable, and early surgical intervention is recommended.
The distinction between type II and type III fractures can be difficult because no clear definition exists to distinguish a “high” type III from a “low” type II fracture.37 Grauer and colleagues38 proposed an alternative modification to the Anderson and D’Alonzo classification scheme to better define suitability of treatment options such as anterior odontoid placement. The authors defined type IIA fractures as minimally or nondisplaced type II fractures with no comminution, type IIB fractures as displaced fractures extending anterior-superior to posterior-inferior or traverse fractures, and type IIC fractures as fractures extending from anterior-inferior to posterior-superior. The authors report that type IIA fractures can often be managed conservatively, type IIB fractures were suitable for anterior odontoid screw placement, and type IIC fractures are treated with posterior atlantoaxial stabilization.
No treatment intervention is considered inappropriate for any type of odontoid fracture except in extreme circumstances (e.g., age > 100, low Karnofsky score, hospice patients).39 Type I odontoid fractures, unless in association with atlanto-occipital dislocation, may be managed with cervical collar alone, with fusion rates of 100% reported (Table 9.3).33,40
Type II odontoid fractures are the most common subtype and have presented the greatest treatment dilemmas to the spine surgeon. Nonunion rates for conservative management of type II odontoid fractures are as high as 75%. Risk factors associated with nonunion of type II odontoid fractures include age > 50 and dens displacement ≥ 6 mm. Lennarson and colleagues41 in Iowa provided class II evidence (case-control study) that substantiates surgical treatment for type II odontoid fractures in patients over age 50. Seybold and Bayley42 reached a similar conclusion, in that patients over age 60 had high symptomatic nonunion rates (19.5%) and lower cervical range of motion when treating type II and III odontoid fractures with halo immobilization. Pepin et al43 noted poor halo tolerance in elderly patients and argued for early surgical intervention in older patients with type II odontoid fractures. Andersson and colleagues44 noted poor union rates in elderly patients treated nonsurgically for type II odontoid fractures and further noted that posterior approaches were superior to anterior odontoid screw in this patient population. Surgical intervention for type II odontoid fractures should also be considered if there is failure to maintain spinal alignment following traction and external immobilization. As mentioned above, type IIA fractures should be treated with early surgical intervention when clinically feasible.36
Type III odontoid fractures may be treated with cervical immobilization with a fusion rate over 80%. Although type III (and type II) odontoid fractures have historically been treated with halo vest immobilization, cervical collars are equivalent to halo devices in prevention of late nonunion or late need for surgical stabilization.45,46 Surgical fixation for type III odontoid fractures may be reserved for patients with nonunion/late instability following attempted nonsurgical management with cervical immobilization or failure to maintain spinal alignment with external immobilization. Our preference is rigid cervical collar over halo orthosis; however, patient compliance and confounding factors such as smoking history and other traumatic injuries must be considered.
Surgical options for odontoid fractures include posterior atlantoaxial wiring techniques (Brooks, Gallie, or Sonntag methods), the Magerl C1-C2 transarticular screw techniue,25 the Harms C1-lateral mass-C2 pedicle screw technique,24 or an anterior odontoid screw in certain cases. Posterior C1-C2 fusion procedures result in greater loss of motion of the atlantoaxial joint but higher fusion rates and fewer contraindications than an anterior odontoid lag screw fusion.24,44
Traumatic Spondylolisthesis of the Axis (Hangman’s Fracture)
A hangman’s fracture is the colloquial name given to a fracture of the pedicles or pars interarticularis (isthmus) of the axis (C2 vertebra). Schneider et al47 coined the term “hangman’s fracture” in 1965 in their series of eight patients whose injuries mimicked the fracture pattern of judicial hanging described by Wood-Jones48 in the first volume of Lancet in 1913.
Traumatic bilateral spondylolisthesis of the pars interarticularis of the axis is a hyperextension and compression with axial loading that is often seen with motor vehicle accidents, falls, and diving accidents. The classic scenarios are that of an unrestrained occupant in a motor vehicle accident who is thrown forward and hits the windshield, or a swimmer who dives and hits the bottom of a shallow pool. In both instances the combined forces of hyperex-tension and axial compression occur. The fracture seen in trauma is similar to that induced by judicial hangings; however, the true judicial “hangman’s fracture” results from distraction and hyperextension, not compression and hyperextension. There is often anterior subluxation of C2 on C3, and it is usually stable, with neurologic deficits being rare. Instability can usually be identified if there is ≥ 4 mm of subluxation of C2 on C3 or ≥ 50% subluxation of C2 on C3, excessive angulation, or significant motion on flexion-extension films
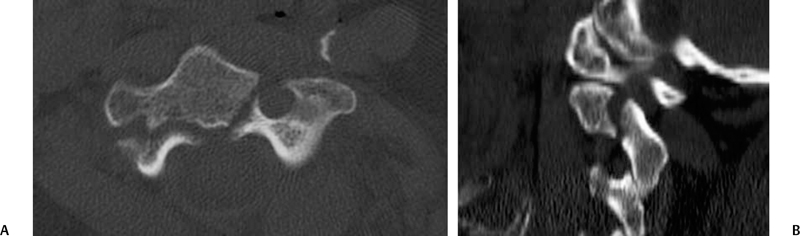
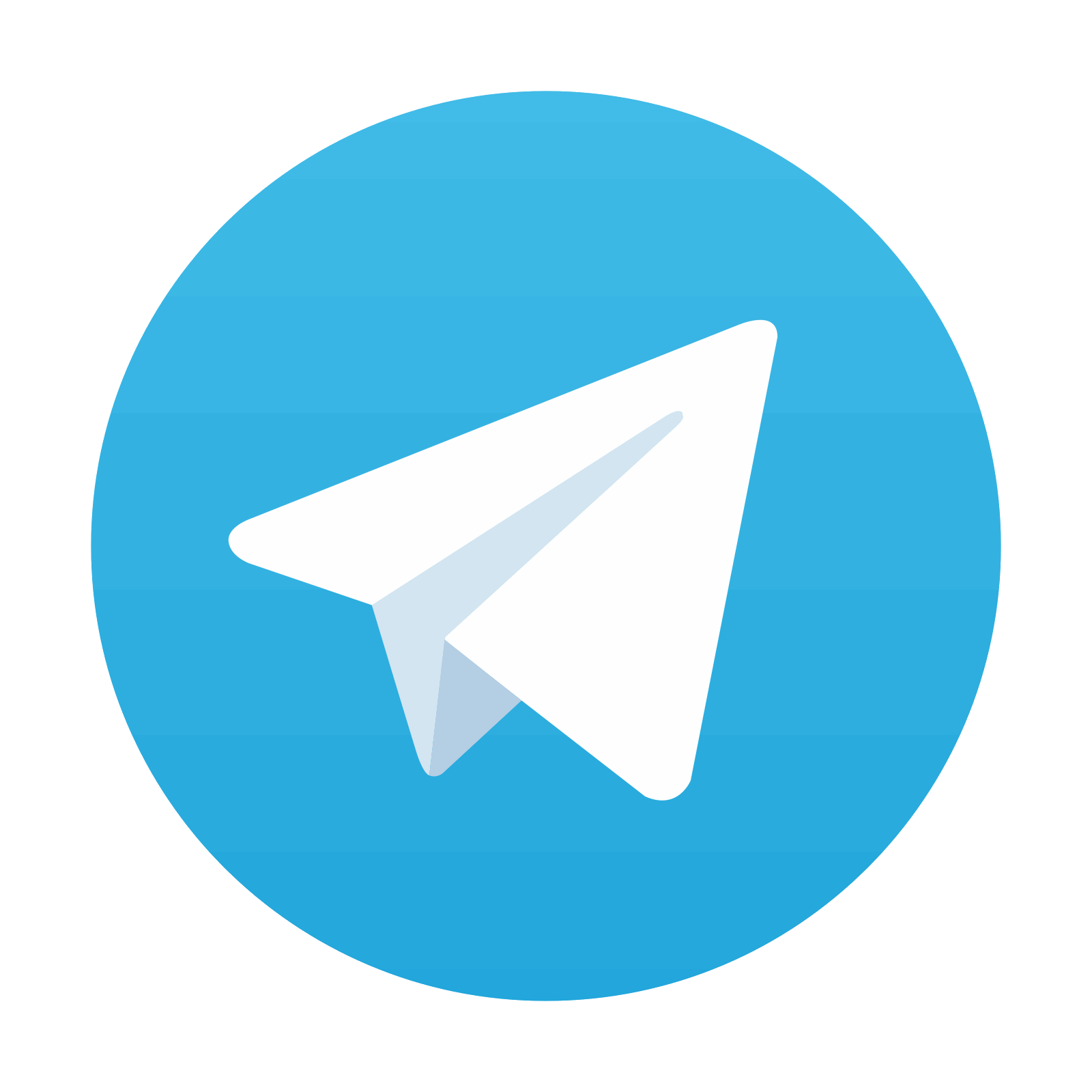