html xmlns=”http://www.w3.org/1999/xhtml” xmlns:mml=”http://www.w3.org/1998/Math/MathML” xmlns:epub=”http://www.idpf.org/2007/ops”>
A 42-year-old male presents for shoulder arthroscopy. After insertion of an intravenous catheter and placement of standard American Society of Anesthesiologists (ASA) monitors, and prior to the induction of general anesthesia, an interscalene block is performed. This is followed by a loss of consciousness, seizure, and cardiac arrest. Advanced cardiac life support is initiated.
Objectives
1. Describe the signs and symptoms of local anesthetic systemic toxicity.
2. Review the pharmacology and physiology that produces mental status changes, seizures, arrhythmias, and cardiac arrest.
3. List the toxic doses of each local anesthetic.
4. Discuss the appropriate treatment protocol for local anesthetic systemic toxicity.
1. Describe the signs and symptoms of local anesthetic systemic toxicity
Local anesthetic systemic toxicity (LAST) develops as a direct result of local anesthetic (LA) accumulation in the blood, typically from unintentional intravascular injections; although, LAST can develop from excessive amounts injected in any anatomic location. Symptoms are manifested in the neurologic or the cardiovascular system. Initially, patients may complain of lightheadedness, tinnitus, and blurry vision. As plasma levels increase, muscle twitching of the face and distal extremities and tremoring can be appreciated, with neurologic consequences culminating with tonic–clonic seizures [1]. Signs of cardiovascular toxicity occur at higher plasma concentrations than neurologic toxicity; however, the magnitude of this disparity is partly dependent on the lipophilicity of the LA. Initially, hypertension and tachycardia are seen secondary to sympathetic stimulation. As plasma levels climb, myocardial depression ensues with bradycardia, hypotension, peripheral vasodilation, and eventual hemodynamic collapse. Atypical presentations exist and cardiovascular toxicity can present in the absence of neurological toxicity. This is more likely while administering more potent local anesthetics. The use of sedation may mask neurologic signs [2].
2. Review the pharmacology and physiology that produces mental status changes, seizures, arrhythmias, and cardiac arrest
The mechanism of action of LAs relies on their ability to impede nerve impulse transmission through blockade of ion-selective sodium channels. This alteration in sodium permeability slows the rate of depolarization to the point that an action potential cannot be transmitted [3].
In order for signs and symptoms of neurotoxicity and cardiovascular toxicity to manifest, increased LA concentrations must reach an active site for toxicity. Since the risk of LAST increases as the LA blood concentrations rise, drug pharmacokinetics and injection location contribute to toxicity after extravascular injection.
Pharmacokinetic properties also impact plasma LA levels (Table 6.1). Elevated volumes of distribution (e.g., prilocaine) result in lower intravascular levels due to a greater presence in peripheral compartments. Non-protonated LA forms permeate cell membranes, increasing systemic absorption; however, the ionized form interacts with the sodium channel. Although cell membrane permeation is expedited with increased lipophilicity, highly lipid-soluble LAs have lower plasma levels due to their sequestration in tissues including the brain and myocardium [3]. This explains, in part, how potent agents have a lower threshold for developing cardiovascular toxicity and can present without neurologic sequelae. Similarly to lipid solubility, greater protein binding results in a reduced free LA fraction. Although protein binding and lipid solubility are inversely proportional to plasma concentration, toxicity is related to the rate of rise of blood concentrations. For example, bupivacaine is highly protein bound but quickly saturates α1-acid glycoprotein and albumin, leaving free bupivacaine to bind myocardial tissue. Metabolism also plays a critical role. Amides are metabolized by microsomal enzymes in the liver and esters are hydrolyzed by plasma cholinesterase in the blood. Local anesthetics with slower rates of metabolism pose the greatest risk for toxicity related to systemic absorption. This is more likely with amides, as their metabolism is dependent upon adequate liver function compared with the relatively rapid hydrolysis of esters. Consequently, prudent planning of regional blocks involves consideration of both pharmacokinetics (Table 6.1) and patient factors affecting absorption and metabolism, such as cardiovascular status, liver function, pregnancy, and age.
Pharmacokinetic properties of commonly used local anesthetics.
Drug | pKa | Protein binding (%) | Lipid solubility | Vd (L) | T1/2 (min) |
---|---|---|---|---|---|
Esters | |||||
Procaine | 8.9 | 6 | 0.6 | 65 | 9 |
Chloroprocaine | 8.7 | – | – | 35 | 7 |
Tetracaine | 8.5 | 76 | 80 | – | – |
Amides | |||||
Lidocaine | 7.9 | 70 | 2.9 | 91 | 96 |
Prilocaine | 7.9 | 55 | 0.9 | 191 | 96 |
Mepivacaine | 7.6 | 77 | 1 | 84 | 114 |
Bupivacaine | 8.1 | 95 | 28 | 73 | 210 |
Ropivacaine | 8.1 | 94 | – | 59 | 108 |
Vd (L): volume of distribution; T1/2 (min): half life.
As vascularity is proportional to systemic uptake, the location of the LA injection is important. Vigilance is essential when using intravenous LAs for analgesic and anesthetic purposes. Intravenous regional anesthesia should be performed, when possible, with ester LAs in order to hasten metabolism and limit LAST. Tourniquet pressures should be decreased sequentially to decrease systemic delivery, especially in procedures of short duration when little anesthetic has metabolized. Further, forearm intravenous regional anesthetics, as opposed to applying the tourniquet to the upper arm, have been shown to limit the volume of LA and incidence of LAST [4–5]. Intravenous infusions of lidocaine are also often used as an anesthetic adjunct. Cumulative administration must be closely monitored to avoid toxic plasma levels.
Among peripheral nerve blocks, airway topicalization followed by intercostal blocks carries the highest risk of systemic toxicity. Recently, Barrington and Kluger described an increase in the risk of toxicity with paravertebral and brachial plexus blocks compared with neuraxial and lower extremity ones [6]. Still, it is difficult to tier sites that carry risk of toxicity with certainty, as patient factors other than relative vascularity contribute to LAST [7]. The addition of epinephrine (5 µg/ml) to LAs has been shown to diminish plasma levels through vasoconstriction; however, the degree to which this occurs depends on the LA chosen.
As the myocardium is more resistant to toxicity, CNS toxicity occurs first and with lower LA doses. Certain symptoms may precede CNS toxicity and afford a chance to halt the progression to seizures through early recognition. Low LA levels can precipitate a metallic taste, tinnitus, or perioral paresthesias likely due to the vascular nature of these tissues. As plasma levels increase, LAs cross the blood–brain barrier and CNS toxicity can be described in two phases: an excitatory phase and a depressive phase [1]. The excitatory phase is caused by blockade of ion-selective sodium channels of inhibitory pathways [1]. This results in restlessness and vestibular abnormalities. As plasma levels increase, dysarthrias are followed by twitching and ultimately tonic–clonic seizures. The depressive phase follows and results in blockade of excitatory pathways, similar to the mechanism described above; symptoms of this phase include unconsciousness and apnea
Although CNS toxicity occurs at lower LA doses with seemingly no cardiovascular consequences, the excitatory phase of CNS toxicity can also result in hypertension and tachycardia. As discussed previously, LAs with increased protein binding are less likely to manifest with systemic toxicity due to a relatively lower free plasma concentration. However, with toxic doses, protein binding sites are quickly saturated, leaving an abundance of unbound drug [3]. Direct cardiotoxicity is caused by LAs blockade of cardiac sodium channels. This causes decreased Purkinje fiber and ventricular depolarization and decreased action potential duration. As plasma concentrations increase, conduction time is prolonged and automaticity is decreased [1, 3]. These pharmacodynamic properties also confer arrhythmogenicity and negative inotropy to LAs. The degree of cardiotoxicity is variable depending on the choice of LA, with bupivacaine seemingly most toxic due to its lipophilicity and decreased propensity to diffuse away from sodium channels. Although lidocaine rapidly blocks sodium channels, it also rapidly diffuses away from the receptors. Bupivacaine significantly slows rapid depolarization leading to unidirectional conduction blocks and reentrant arrhythmias [8]. The recovery phase of sodium channels is prolonged and thus the refractory period increases, predisposing to ventricular dysrhythmias. The negative inotropy may also be explained by bupivacaine’s blockade of calcium channels and adrenergic receptors [9–10]. Furthermore, bupivacaine sequesters fatty acids, which are necessary for normal metabolic processes within the mitochondria of the myocardium (Table 6.1).
3. List the toxic doses of each local anesthetic
Toxicity is based on plasma concentrations because of the variable absorption and metabolism pharmacokinetics (Table 6.2). Threshold doses for toxicity create a safety margin to prevent LAST; however, these published doses do not take into account the injection site or patient physiology as discussed previously. Furthermore, these dose limits are not evidence based and there is no support for choosing weight-based dosages and designating them as maximal allowable ones [7]. Instead, dosing of LA should be patient and technique specific. Doses should be tailored based on the specific block site, use of vasoconstrictors, underlying organ dysfunction, pregnancy, and age. Kidney failure causes decreased clearance while uremia enhances absorption due to an upregulated circulation [7]. Hepatic dysfunction may alter the metabolism of certain amides while heart failure negatively affects blood flow to these organs. Neuronal sensitivity to LAs is increased during pregnancy due to normal hormonal variations while protein binding is decreased. In addition, neuraxial techniques in parturients require dose reduction due to a narrowed epidural space, lower cerebrospinal fluid volume, and engorgement of epidural veins.
Local anesthetic plasma concentration limits and recommended dosing to minimize risk of LAST.
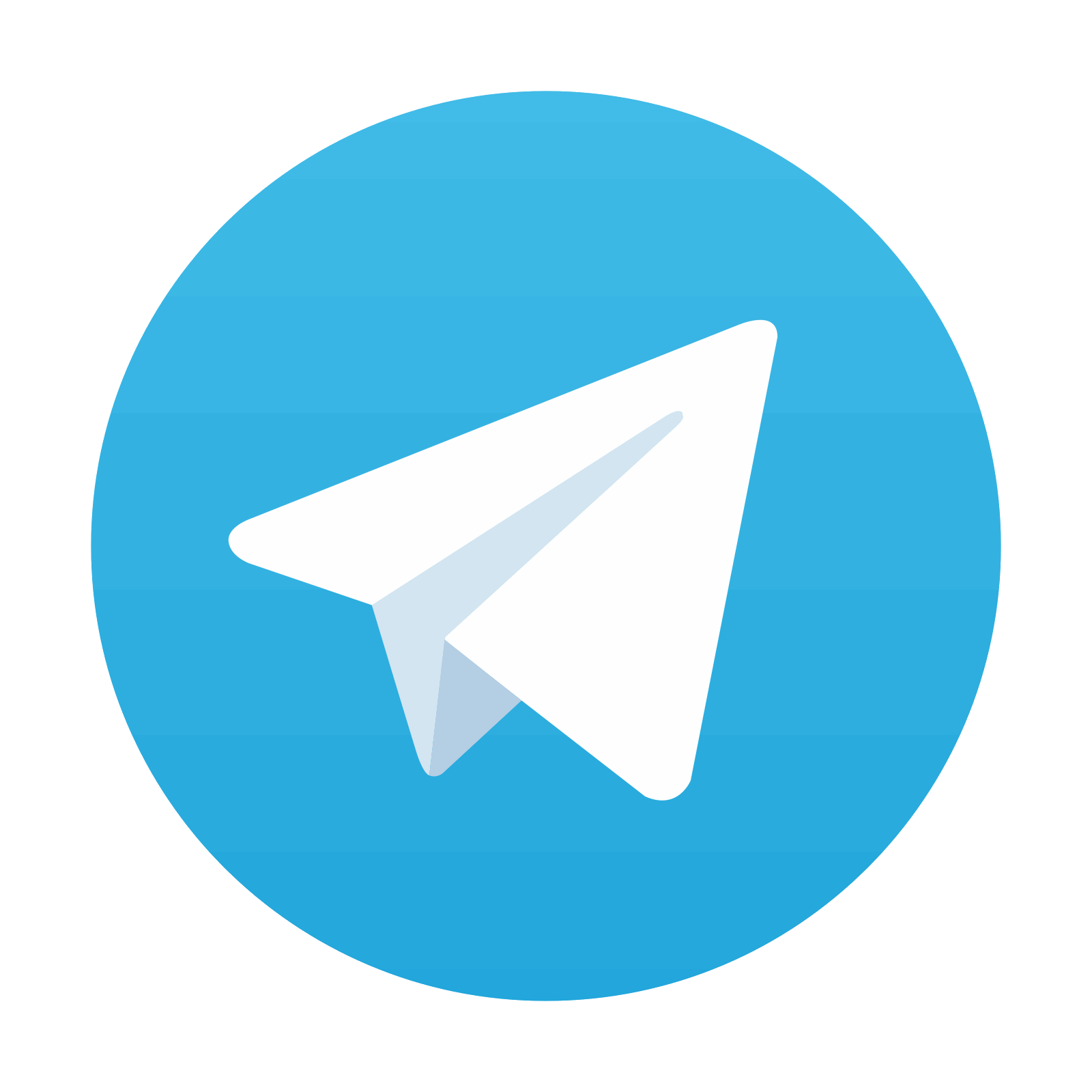
Stay updated, free articles. Join our Telegram channel

Full access? Get Clinical Tree
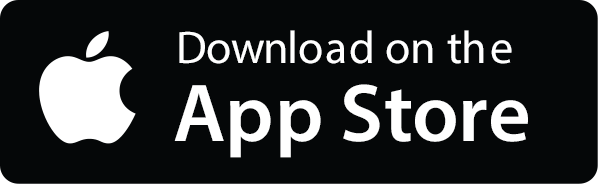
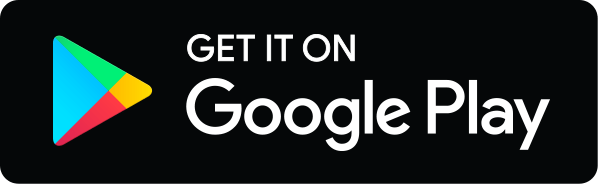