Non-cholestatic cirrhosis
Hepatitis C
Hepatitis B
Alcoholic liver disease
Autoimmune hepatitis
Cryptogenic cirrhosis
Nonalcoholic steatohepatitis
Others (hepatitis D, hepatitis A, hepatitis coinfection, chronic active hepatitis, other exposure)
Cholestatic liver disease/cirrhosis
Primary biliary cirrhosis
Secondary biliary cirrhosis (Caroli’s Disease, Choledochal Cyst, other)
Primary sclerosing cholangitis (ulcerative colitis, Crohn’s Disease, no bowel disease, other)
Others
Biliary atresia
Alagille syndrome
Hypoplasia
Extrahepatic
Others
Acute hepatic necrosis
Acute hepatic necrosis (hepatitis, drug, unknown etiology, other)
Hepatitis C: chronic or acute
Hepatitis B: chronic or acute
Metabolic diseases
Alpha-1-antitrypsin deficiency
Hemochromatosis–Hemosiderosis
Other hereditary disorders (Wilson’s Disease, tyrosinemia, oxalosis, glycogen storage diseases, others)
Malignant neoplasms
Primary liver malignancy
Hepatocellular carcinoma (with or without cirrhosis)
Cholangiocarcinoma
Hepatoblastoma
Others (fibrolamellar hepatocellular carcinoma, hemangioendothelioma–hemangiosarcoma)
Secondary liver malignancy
Miscellaneous conditions
Budd-Chiari Syndrome, metastatic neuroendocrine tumors, cystic fibrosis, trauma, benign tumors, others)
Retransplantation
Primary nonfunctioning
Acute/chronic rejection
Hepatic artery thrombosis
Chronic Liver Failure: Etiologies, Systemic Manifestations, and Anesthetic Implications
It is important to realize that ESLD causes multiple systemic disorders (Table 28.2). Each systemic manifestation has its significant anesthetic implication during the perioperative period, thus demanding refinement in anesthetic management.
Table 28.2
End-stage liver disease: systemic manifestations
Organ system/manifestation |
---|
Cardiovascular-pulmonary systems |
Hyperdynamic state |
Portal hypertension |
Portopulmonary hypertension |
Right heart failure |
Hepatopulmonary syndrome |
Cirrhotic cardiomyopathy |
Pleural effusion |
Renal-electrolytes system |
Hepato-renal syndrome |
Hyperkalemia |
Metabolic acidosis |
Hyponatremia |
Hematological system |
Coagulopathy |
Anemia |
Thrombocytopenia |
Leukopenia |
Spontaneous bacterial peritonitis |
Gastrointestinal system |
Esophageal varices |
Portal hypertensive gastropathy |
Mucosal dysfunction of the intestine |
Nervous system |
Encephalopathy |
Endocrine system |
Diabetes mellitus |
Abnormal sex hormone metabolism |
Thyroid disease |
Osteoporosis |
Adrenal insufficiency |
Cardiovascular and Pulmonary Systems
Patients with ESLD undergoing liver transplantation often present with hyperdynamic circulatory conditions, portal hypertension, portopulmonary hypertension, right heart failure, hepatopulmonary syndrome, cardiomyopathy, and pleural effusion. Systemic vasodilatation and formation of collateral veins (e.g., porto-systemic shunts) lead to hyperdynamic splanchnic and systemic circularity conditions. Persistent endotoxemia, caused by shunting through porto-systemic anastomoses and enhanced endotoxin absorption from the intestine as a result of bile salt deficiency, may contribute to the vasodilation by activation of cascades of secondary mediators. Portal hypertension results from hyperdynamic splanchnic circulation with increased afterload of the portal venous system with intrahepatic cirrhosis. This leads to ascites, splenomegaly, varicose vein formation in the esophagus, portal hypertensive gastropathy, and spontaneous bacterial peritonitis. Portopulmonary hypertension can be categorized as mild (mean pulmonary arterial pressure [MPAP] 25–44 mmHg), moderate (MPAP 45–59 mmHg), and severe (MPAP ≥ 60 mmHg). Moderate to severe portal hypertension is associated with high perioperative mortality in liver transplantation [3] and is considered a contraindication for isolated liver transplantation unless successfully medically managed pretransplant [4]. Right heart failure can be found in patients with pulmonary hypertension. Dilatation of the right ventricle, decreased wall motion of the right ventricle, tricuspid regurgitation, and dilatation of the right atrium are hallmarks of the condition. Hepatopulmonary syndrome (HPS) is characterized by microvascular alterations and dilatation in the pre-capillary and capillary pulmonary arterial circulation. HPS is defined as a widened alveolar-arterial oxygen gradient (AaPO 2) on room air in the presence or absence of hypoxemia (AaPO 2 = 15 mmHg, or 20 mmHg in patients more than 64 years old) as a result of intrapulmonary vasodilation. HPS can be graded on the basis of the degree of hypoxemia: mild (PaO 2 ≥ 80 mmHg); moderate (PaO 2 = 61–80 mmHg), severe (PaO 2 = 50–60 mmHg), and very severe (PaO 2 < 50 mmHg) [5]. Cirrhotic cardiomyopathy is a recently recognized condition and can be caused by any etiology of ESLD. It presents with systolic incompetence under hemodynamic stress, diastolic dysfunction related to altered diastolic relaxation and electrophysiological abnormalities in the absence of any known cardiac disease. The underlying pathogenetic mechanisms include abnormalities in the β-adrenergic signaling pathway, altered cardiomyocyte membrane fluidity, increased myocardial fibrosis, cardiomyocyte hypertrophy, and ion channel defects with widening of the QRS complex causing prolonged QT intervals. The clinical manifestations of this condition become relevant only in decompensated conditions or immediately after liver donor graft reperfusion with significant volume overload. Pleural effusion can be of a significant amount and may contribute to intraoperative hypoxemia due to atelectasis and decreased ventilation of the affected side of the lung.
Renal and Electrolyte Systems
Hepato-renal syndrome (HRS) [6] results from the cascade of events caused by ESLD with portal hypertension and mesenteric hyperemia; the two conditions cause relative renal hypo-perfusion, resulting in severe renal arterial vasoconstriction and progressive renal failure. Two types of HRS are observed in clinical practice [7]. Type 1 HRS is an aggressive form with a very poor prognosis, and type 2 HRS develops slowly over weeks; these patients usually have diuretic-resistant ascites and have a slightly better prognosis than those with type 1 HRS.
As a result, hyperkalemia and metabolic acidosis can be seen in patients with ESLD. Hyponatremia is also a common finding in ESLD patients. The pathogenesis is directly related to the vasodilatation and secondary neurohumoral adaptations that occur, including activation of endogenous vasoconstrictors such as antidiuretic hormone. This process leads to an impaired ability to excrete ingested water. Severity of the hyponatremia is related to the severity of ESLD.
Hematologic System
Decreased synthetic function of the liver with ESLD leads to decreased production of procoagulant factors including vitamin K-dependent factors, factor V, and factor XI. Dysfibrinogenemia is caused both by the decreased production and by increased consumption of fibrinogen due to altered production of activators and inhibitors of fibrinolysis, activation of coagulation cascade by endotoxemia, and decreased clearance of fibrinolytic proteins. Of note, traditional laboratory-based coagulation tests, including prothrombin time, partial thromboplastin time, and fibrinogen level, do not necessarily reveal the entire picture of coagulation. This point is important as the coagulation status is a fine balance between these two opposing factors [8]: pro-coagulants and anti-coagulants. Therefore, aggressive correction of coagulation abnormalities measured by these laboratory tests with exogenous coagulation factors and blood products occasionally results in thromboembolic complications at liver transplantation [9]. Thrombocytopenia is a common feature in ESLD. This is primarily due to hypersplenism secondary to portal hypertension, but decreased production of hepatic thrombopoietin synthesis as well as direct bone marrow suppression with alcohol exposure or hepatitis C virus also play a role. Anemia is seen due to a combination of hemorrhage from the gastrointestinal tract, decreased production of red blood cells (bone marrow suppression and/or folate deficiency), and hemodilution due to water retention. Leucopenia can be seen due to bone marrow suppression with viral hepatitis B or C, and excessive alcohol consumption. Together with leukopenia and decreased production of the compliments, patients can be prone to infections including spontaneous bacterial peritonitis.
Gastrointestinal System
Esophageal varices and portal hypertensive gastropathy are primary abnormalities that occur due to ESLD. In general, varices can form at any portion of the alimentary tract from the esophagus to the rectum, but the distal esophagus is the most common site for varices in ESLD. Esophageal varices result from portal hypertension and often bleed. Child-Pugh score, variceal size, and presence of red wale markings can be used to calculate a prognostic index that quantifies the risk of variceal hemorrhage [10]. An endoscopic banding procedure is occasionally performed during the pretransplantation period either to therapeutically treat bleeding varices or for prophylactic purposes. The timing of this banding procedure and the severity of the varices are important to consider for intraoperative placement of transesophageal echocardiography (TEE) . Portal hypertensive gastropathy [11] has the characteristic endoscopic features of a mosaic pattern with or without red spots. It is most frequently located at the fundus and body of the stomach. Acute bleeding from portal hypertensive gastropathy is usually mild and seen in the presence of severe portal hypertension. Mucosal dysfunction of the intestine due to portal hypertension leads to malabsorption and bacterial translocation [12]. The former leads to malnutrition; the latter leads to bacteremia and spontaneous bacterial peritonitis as well as the main pathogenesis of hepatorenal syndrome due to splanchnic and systemic vasodilation.
Nervous System
Hepatic encephalopathy [13] indicates the spectrum of potentially reversible neuropsychiatric abnormalities observed in patients with liver dysfunction. There are three types of hepatic encephalopathy: Type A is associated with acute liver failure; Type B is associated with portal-systemic bypass and no intrinsic liver disease; and Type C is associated with ESLD. Therefore, hepatic encephalopathy of liver transplant patients are categorized as Type C, and are further subcategorized into those with episodic hepatic encephalopathy, persistent hepatic encephalopathy, and minimal hepatic encephalopathy. In terms of symptom severity, the West Haven criteria [14, 15] for semi-quantitative grading of mental status have been used to score the grade of clinical severity: mild (Grade 1), moderate (Grade 2—lethargy/minimal disorientation/subtle personality change), severe (Grade 3—somnolence/confusion/disorientation), or Grade 4 (coma). Several metabolic factors contribute to the development of hepatic encephalopathy, which include ammonia, inhibitory neurotransmission through gamma-aminobutyric acid receptors in the central nervous system, and changes in central neurotransmitters and circulating amino acids [16].
Endocrine System
Diabetes mellitus is seen in 15–30 % of patients with cirrhosis [17]. Insulin resistance is present in many patients with nonalcoholic steatohepatitis and chronic hepatitis C. Cirrhosis has also been linked to abnormalities in the other endocrine glands, including abnormal sex hormone metabolism, thyroid disease (hypo- and hyperthyroidism), osteoporosis, and adrenocortical dysfunction.
MELD Score
The Model for End-Stage Liver Disease (MELD) score is a grading system for evaluating the severity of chronic liver diseases for patients age 12 and older. Candidates age 11 and younger are graded with the Pediatric End-Stage Liver Disease (PELD) scoring system . The MELD system was originally developed to predict 3 month-mortality in patients undergoing a transjugular intrahepatic portosystemic shunt (TIPS) procedure [18]. It has been used for the allocation of livers to adults since February 2002 in the United States; this system better predicts liver transplantation outcome compared to the traditional Child-Pugh score. MELD score is calculated based on the three laboratory values (bilirubin, creatinine, and international normalized ratio [INR]): [19]
where Ln is the natural logarithm.
![$$ \mathrm{MELD}=3.8\ \left[\mathrm{L}\mathrm{n}\ \mathrm{serum}\ \mathrm{bilirubin}\ \left(\mathrm{mg}/\mathrm{dL}\right)\right]+11.2\ \left[\mathrm{L}\mathrm{n}\ \mathrm{I}\mathrm{N}\mathrm{R}\right]+9.6\ \left[\mathrm{L}\mathrm{n}\ \mathrm{serum}\ \mathrm{creatinine}\ \left(\mathrm{mg}/\mathrm{dL}\right)\right]+6.4 $$](/wp-content/uploads/2018/07/A312535_1_En_28_Chapter_Equa.gif)
The United Network for Organ Sharing (UNOS) modifies the currently-used MELD system in two ways. In order to eliminate negative values, the lowest values of each laboratory tests is set at 1.0 (i.e., creatinine of 0.8 mg/dL is automatically changed to 1.0). Therefore, the minimum MELD score becomes 6. In order to avoid giving an unfair advantage to patients with intrinsic renal disease, the maximum serum creatinine level is set at 4.0 mg/dL, which is also the value automatically assigned to patients on dialysis. For allocation purposes, the upper level of the MELD score is capped at 40. Thus, UNOS modified the MELD score for liver transplantation allocation to range from 6 to 40. A higher MELD score indicates increased mortality in the waiting period, thus patients are prioritized for liver transplantation with a higher ranking in the waiting list of a given blood type. MELD scores are updated regularly, especially for patients with severe illness. For example, patients with MELD scores ≥25 have their scores updated weekly. Under the current deceased donor liver allocation system, patients with acute liver failure are exempt from the MELD-based prioritization process outlined above. Patients with acute liver failure are prioritized as UNOS Status 1A or Status 1B. Status 1A patients have a life expectancy of hours to a few days without a liver transplant. Status 1B is reserved for very sick, chronically ill pediatric patients (age less than 18). Also, several conditions receive “Standard MELD Exceptions ”; they receive a higher than calculated MELD score due to higher mortality. These conditions include hepatocellular carcinoma (HCC), hepatopulmonary syndrome, portopulmonary hypertension, familial amyloid polyneuropathy, primary hyperoxaluria, cystic fibrosis, and hilar cholangiocarcinoma. The anesthesia team should be well aware of patients’ MELD scores, since patients with high MELD score have been demonstrated to have a higher incidence of complications and mortality at liver transplantation.
The PELD system is calculated using parameters including bilirubin, INR, albumin, growth failure, and age (less than 1 year old or not).
Other Recipient Conditions
The following recipient conditions warrant special anesthetic considerations from the anesthesiologist team. Fulminant hepatic failure is defined as acute liver necrosis without any preexisting liver disease. This condition is one of the MELD-exempt conditions; the severity and priority for liver transplantation would be gauged with another grading system (i.e., King’s College Criteria [20]). In addition to rapidly progressing coagulopathy, renal failure, metabolic acidosis, and respiratory failure, increased intracranial pressure can be an important challenge to successful perioperative anesthetic management (Chap. ##). Patients with hepatopulmonary syndrome or portopulmonary hypertension also receive MELD-exception treatment. Severe intraoperative hypoxemia or acute right heart failure could be major challenges in the care of these patients during the peri-transplantation period. These conditions are discussed further in Chap. ##. Emergent retransplantation is indicated for a primary nonfunctioning liver graft. Primary nonfunctioning is defined as an aggravated form of reperfusion injury resulting in irreversible graft failure without detectable technical or immunological problems [21, 22]. It is the most common reason for early retransplantation [23], with a reported incidence of 4–8 % [24]. These patients present at the ICU after recent liver transplantation with or without hepatectomy of the nonfunctioning liver graft. These patients are transferred from the ICU to the operating room with full monitoring and vascular accesses and the retransplantation is performed with a minimal dissection stage; however, a prolonged “anhepatic state” due to graft failure often causes severe coagulopathy and metabolic derangement including hyperkalemia and metabolic acidosis. Retransplantation for chronic rejection is often associated with adhesion and a prolonged dissection phase with surgical bleeding and incurred risk of massive transfusion. Budd-Chiari syndrome is a rare cause of portal hypertension and liver failure, which often results from hypercoagulable states induced by polycythemia vera, essential thrombocytosis, and myeloid metaplasia [25, 26]. These patients can present for liver transplantation with or without porto-systemic shunt surgeries or transjugular intrahepatic porto-systemic shunts (TIPS). Intraoperatively, establishment of vascular accesses could be challenging and a thorough review of the preoperative venography is important for planning.
Types of Liver Grafts and Their Implications
A liver transplantation involves the whole liver or a reduced-sized liver (a split graft or a liver segment). Transplantation of the latter would allow two liver recipients to receive a liver from one deceased donor or allow for living donor liver donation. A reduced-size liver transplant may result if the donor liver is too large for the recipient. Liver grafts can be donated from either deceased or living donors. The former can be categorized into donation after brain death (DBD) donors and donation after circulatory (or cardiac) death (DCD) donors . Living donor grafts consist of right lobe donations for mainly adult recipients or left lobe donations for mainly pediatric recipients. In 2011 in the United States, 5805 adult liver transplants were performed, which included transplant of 5351 livers from DBD donors, 266 from DCD donors, and 188 from living donors [1]. Concerns about donor safety has decreased the enthusiasm for living donor liver transplantation in the United States, and recently the number of donations from living donors plateaued at about 250 annually. For deceased donors, extended (or expanded) criteria donation (ECD) liver grafts have been utilized increasingly due to the shortage of donors with standard criteria donation (SCD) . The definition of ECD has been defined well in kidney transplantation; however for liver transplantation, the criteria are not necessarily unanimous and are defined per transplantation center [27].
The course of anesthesia for liver transplantation is often dictated by the category and the quality of the donor liver graft. For example, living donor liver transplantation for an adult recipient usually takes place alongside donor hepatectomy; therefore, coordination of the timing of two operations (donor and recipient) is important. In general, the cold ischemic time in living donor liver transplantation is markedly shorter than that in deceased donor liver transplantation. Liver transplantation using ECD graft [28, 29] or grafts with higher donor risk indexes [30] may result in primary nonfunctioning and delayed functioning. These conditions can present with significant hemodynamic derangement post re-perfusion with refractory coagulopathy and lactic acidosis.
Surgical Methods and Their Anesthetic Implications
Discussion with the transplant surgical team regarding the surgical method of the liver transplantation is crucial as each surgical team and institution has its own method. Basically, the liver graft is implanted either with preservation of the retrohepatic inferior vena cava (IVC) (the so-called “piggyback” method) or the traditional retrohepatic caval resection (the so-called “standard” method). The piggyback method , first described by Tzakis et al. in 1989 [31], has become widely adopted and is the contemporary technique of choice for liver transplantation [32]. This technique preserves the venous return from the lower body via the IVC with application of a side clamp on the IVC to exclude the hepatic vein for circulatory system for hepatectomy of the diseased liver. In such a way, the venous stasis of the lower body and the kidneys is avoided and the preload of the heart during the anhepatic stage can be maintained. On the other hand, the retrohepatic caval resection technique was described as the original method of liver transplantation hepatectomy [33]. Clamping at the supra-hepatic IVC as well as at the infra-hepatic IVC are required to exclude the liver from the circulation for hepatectomy, which often results in venous stasis in the lower body as well as the kidneys; venous return is compromised and the preload of the heart is decreased. When venous collateral vessels are well established, this drawback can be minimized. Prior to the procedure, the surgical team may test hemodynamic conditions with a test application of the IVC clamp. Therefore, the anesthesiology team should prepare for potential hypotension after the application of the IVC clamping, which requires inotropes with judicious usage of volume infusion. Alternatively, to minimize the drawbacks associated with the IVC clamping as well as minimize the venous stasis of the portal system, veno-venous bypass (VVB) was developed [34] and has been used in selected centers. The idea is to insert drainage cannula both in the portal vein and the femoral vein and return the venous blood to the upper body venous system using a centrifugal pump. Traditionally, the return cannulas are placed in the axillary vein with a surgical cut down technique; however, the percutaneous technique using the internal jugular vein is advocated to avoid wound complications by axillary cut down (infection, seroma, or nerve damage). The anesthesiology team may be asked to place the return cannula via the internal jugular vein and play a major role in the VVB initiation and termination [35].
Intraoperative Anesthetic Management
Induction and Maintenance of General Anesthesia and Anesthetic Agents of Choice
The recipients’ preoperative condition is reviewed and examined for any further changes; any sign of deterioration of the condition compared to the pretransplantation workup should be investigated. Judicious use of anxiolytics is recommended to avoid oversedation prior to the induction of general anesthesia, as the bioavailability of benzodiazepine usually high due to low serum albumin. Given the urgency of the transplantation and possible delayed gastric emptying due to ESLD, the patient should be treated as if they have a full stomach and rapid sequence induction to secure the airway is warranted; however, routine use of succinylcholine in this scenario should be avoided due to a concern for acute hyperkalemia associated with this agent. Intravenous induction agents including propofol or etomidate can be safely used. When post induction hypotension is a concern, the latter agent may be preferred. Potential adrenal suppression with etomidate may be mitigated by the glucocorticoid administration for immunosuppression regimen. Maintenance of anesthesia can be easily achieved with the balanced technique using inhalational agents, nondepolarizing muscle relaxants, and opioids. If fast track anesthesia is planned to remove the endotracheal tube early in the postoperative period, the rapid offset agents can be selected, including sevoflurane or desflurane, rocuronium if sugammadex as a reversal agent is available, and remifentanil infusion.
Several inotropes of choice (epinephrine, dopamine, or norepinephrine) should be prepared for any unexpected hemodynamic changes. Vasopressin should also be available in case of refractory hypotension. A cell salvage device and a rapid infusion system should also be available in the operating room and ready for use if indicated. Prophylactic antibiotics (a third generation cephalosporin of choice) should be given prior to the skin incision and timely re-dosing during the operation. In case of massive bleeding, the timing of re-dosing should be shortened to maintain the effective plasma concentration of antibiotics. For intraoperative fluid maintenance, any isotonic-potassium and glucose-free crystalloids should be adequate. Excessive usage of normal saline solution should be avoided, since a sudden increase in serum sodium level may result in acute central pontine myelinolysis [36].
Vascular Accesses and Monitoring
The degrees of cardiovascular and pulmonary system involvement in ESLD as well as the invasiveness of the surgical transplant method dictate selection of invasive hemodynamic monitoring. However, each transplantation institution has its own institutional guidelines based on its philosophy and historical practice [37]. These institutional practices can vary significantly from a minimalist approach (one arterial line, several large bore intravenous lines with or without central venous line) to a maximalist approach (two arterial lines, two central lines with a pulmonary arterial [PA] catheter with continuous cardiac output measurement and TEE). The advocates of two arterial lines (one radial arterial line and the other via a central arterial system: contralateral side of the brachial artery or the femoral artery) are based on observations that a central arterial line would better represent the central arterial pressure than a radial artery, especially under hypotensive conditions, would serve as a failsafe measure during the surgery, and would allow continuous monitoring during phlebotomy via the other arterial line. The two central lines may assure the two independent rapid infusion sites of blood and fluid at the time of hemodynamic disaster, while allowing placement of a PA catheter. A PA catheter provides direct measurement of right-sided pressures as well as pulmonary arterial pressure, which is crucial when the recipient has preexisting pulmonary arterial hypertension. The catheter can provide continuous monitoring of pulmonary arterial pressure postoperatively in the ICU. TEE can be especially useful to evaluate right ventricular function that demonstrates pulmonary hypertension to rapidly diagnose the potential cause of cardiac collapse (hypovolemia, myocardial depression, and clot/air embolism), and to evaluate the performance of VVB. If VVB placement using the axillary cut down technique is anticipated, a venous access on the ipsilateral side of the arm should be discouraged, since infusion via the distal site of the same side of the axillary cut down will be obliterated.
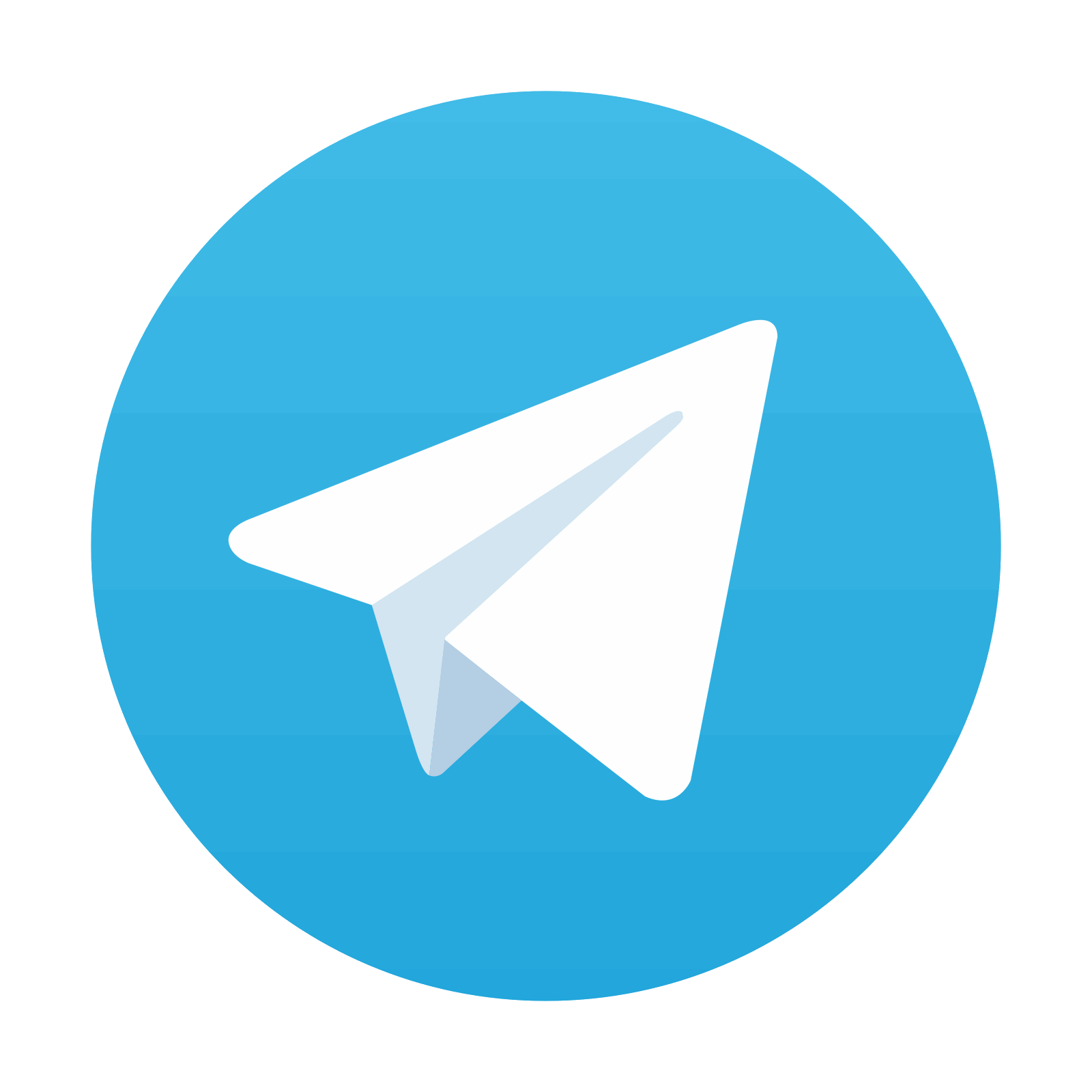
Stay updated, free articles. Join our Telegram channel

Full access? Get Clinical Tree
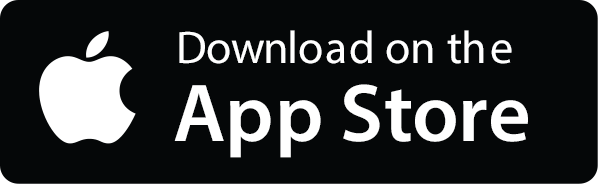
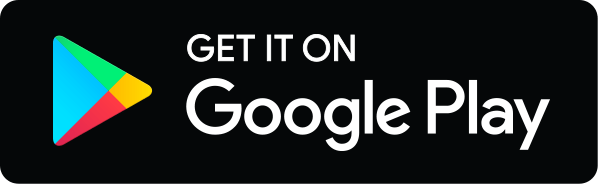