Chapter 4
Liberation and Weaning from Mechanical Ventilation and Extubation 
1. Ascertain the patient’s baseline health and respiratory status, i.e., before the development of acute respiratory failure, by obtaining a thorough medical history from the patient or the patient’s family.
2. Determine why mechanical ventilation was first initiated, appreciating both the mechanism and pathophysiology of the patient’s respiratory failure (see Chapter 1).
3. Determine the progress the patient has made toward recovery.
4. Assess the patient’s ability to maintain adequate oxygenation, ventilation, and airway protection.
When to Stop Mechanical Ventilation: The “First Fix What’s Broken” Approach
In this approach, one starts with the underlying assumption that patients cannot be successfully removed from mechanical ventilation unless the problems causing their respiratory failure in the first place are treated and reversed. To accomplish this, one should begin by identifying how the patient’s respiratory failure developed (see Chapter 1). Failure of nonrespiratory organ systems that contributed to the need for mechanical ventilation (e.g., cardiac arrest resulting from a primary cardiac arrhythmia) also need to be addressed appropriately before mechanical ventilation is stopped.
In the aforementioned example, the workload required by the patient’s respiratory pump (also referred to as the ventilatory demand) is increased by the presence of (1) an increased ratio of dead space to tidal volume (VD/VT), (2) airflow obstruction, (3) pulmonary edema, (4) atelectasis, and (5) increased CO2 production (from shivering) (Table 4.1). At the same time, the ventilatory pump capacity may be limited by the surgical incision and pain, loss of lung volumes from multiple causes, respiratory muscle dysfunction caused by phrenic nerve injury, poor diaphragmatic perfusion, electrolyte disorders, and residual effects of neuromuscular blockers (Table 4.2).
TABLE 4.1
Factors and Causes Increasing Ventilatory Demand
Factors | Causes |
Increased VD/VT | Acute respiratory distress syndrome, asthma, emphysema, pulmonary emboli |
Increased oxygen consumption | Fever, increased work of breathing, morbid obesity, sepsis, shivering, trauma |
Increased respiratory quotient (increased CO2 production relative to O2 consumption) | Excessive carbohydrate feeding |
Decreased set point for PaCO2 | Anxiety, central neurogenic hyperventilation, hepatic failure, hypoxemia, metabolic acidosis, renal failure, sepsis |
VD/VT, dead space-to-tidal volume ratio.
From Lanken PN: Respiratory failure: an overview. In Carlson RW, Geheb MA (eds): Principles and Practice of Medical Intensive Care. Philadelphia: WB Saunders, 1993, pp 754-763.
TABLE 4.2
Factors and Examples Reducing Ventilatory Capacity
Adapted from Lanken PN: Respiratory failure: an overview. In Carlson RW, Geheb MA (eds): Principles and Practice of Medical Intensive Care. Philadelphia: WB Saunders, 1993, pp 754-763.
Categories of Problems to Consider and Fix
Neurologic Impairment and Central Nervous System Drive Problems
1. Loss of upper airway protective reflexes
2. Decreased level of consciousness
3. Effects on the central respiratory drive by hypoventilation syndromes or metabolic acid-base disturbances
Loss of Upper Airway Protection
After extubation, some patients may be at risk of clinically significant aspiration or failure to clear their respiratory secretions. Both can prevent patients from being able to safely maintain spontaneous, independent breathing. Before extubation, patients should be assessed for the presence of adequate cough and gag reflexes and their ability to cough well enough to clear their secretions (Table 4.3). Although unsettled, there are data that some patients without a gag reflex can still be successfully extubated. If patients lack a good cough, however, and are unable to clear secretions by themselves, weaning can continue but extubation should be delayed. If, with repeated testing over several days, the patient cannot adequately protect the airway or clear tracheal secretions, the patient should get an elective tracheostomy. This allows a secure access to the airways for suctioning secretions and is more comfortable than continuation of an endotracheal (ET) tube. A tracheostomy is also useful for monitoring aspiration of oral fluids during assessment of swallowing function and usually enhances the patient’s ability to communicate (Chapter 22).
TABLE 4.3
Criteria for Adequate Protection of Upper Airway∗
∗Criteria 1 through 3 must be met before extubation. If only criterion 4 is not fulfilled, the patient can be extubated except if there is a high risk of massive aspiration, such as with partial small bowel obstruction. In all cases, swallowing function after extubation must be carefully tested before any oral intake (see Chapter 22).
The presence of swallowing dysfunction should be assessed in all recently extubated patients before oral intake. This is especially important in patients with poor or absent gag reflexes, patients with tracheostomies, or those with a history of suspected aspiration events (Chapter 22).
Decreased Mental Status
Several pivotal trials have demonstrated that strategies that minimize sedation can positively influence key patient outcomes, including ventilator weaning. Daily interruption of sedation—with sedatives held in mechanically ventilated patients until they are wakeful—has been found to reduce the average number of days patients spend requiring assisted ventilation. Additional approaches to minimize sedation, including the use of shorter-acting agents or administration of sedative medications in bolus form, especially if done according to a goal directed sedation protocol (carried out by the ICU nursing staff) as opposed to continuous intravenous (IV) infusions, have also been shown beneficial in weaning (Chapter 5). Furthermore, combining the practice of daily sedation interruptions with spontaneous breathing trials (SBTs) resulted in a greater number of ventilator-free days in mechanically ventilated medical patients in a multicenter trial in the United States. Interruption of sedation, however, may increase the risk of self-extubation, and patients should be closely monitored as they resume consciousness.
In addition to medication-induced alterations in consciousness, it is important to assess for hypoventilation resulting from central sleep apnea (CSA), as this may also compromise weaning. CSA occurs as a result of impaired sensitivity to PaCO2 or Pao2 levels and can manifest in several ways, including as obesity hypoventilation syndrome (see Chapter 80) or periodic breathing (Cheyne-Stokes breathing). This is particularly problematic for patients ventilated with weaning modes that require them to initiate breaths. Patients with CSA may not respond to increasing hypercapnia with the expected elevation in respiratory rate or tidal volume. Moreover, these patients may appear comfortable during a weaning trial, despite an increased PaCO2 level, which is only detected by measuring arterial or central venous blood gases. Not considering hypoventilation syndromes in the differential for difficult weaning may make patients seem unweanable when, in fact, they only require scheduled ventilatory support at night and with naps (Chapter 25).
In contrast to patients with hypoventilatory syndromes who do not breathe enough, some patients breathe too much. This includes some patients with brain stem strokes who present with marked tachypnea as a result of central neurogenic hyperventilation. This is challenging because, in general, adults cannot sustain persistent respiratory rates of more than 36 to 40 breaths per minute for too long before they develop respiratory muscle fatigue. Suppression of respiratory drive by high-dose opioids may be successful in such patients, allowing them to be weaned.
Metabolic Acid-Base Disorders
In addition to alterations in ventilation secondary to sedation or CSA, the CNS control of respiratory drive is also affected by both metabolic acidoses and alkaloses. Normally, patients compensate for a metabolic acidosis (serum HCO3− <18 to 20 mEq/L) by “blowing down” (compensating) their PaCO2 to maintain their arterial pH in or near the normal range. This respiratory compensation is achieved by hyperventilating, which increases alveolar and minute ventilation (see Chapter 83), but ultimately it may exceed a person’s ventilatory capacity and lead to respiratory muscle fatigue. Thus, in addition to correcting the underlying cause of the metabolic acidosis, patients may require bicarbonate supplementation in order for them to wean.
Alternatively, patients with a severe metabolic alkalosis (serum HCO3– >45 to 55 mEq/L) may have an elevated arterial pH, which results in a decreased stimulus to breathe, even when their PaCO2 levels increase (Chapter 83). This can promote development of hypercapnia, as respiratory compensation for the metabolic alkalosis. Hypercapnia, in these situations, may be mistakenly attributed to respiratory muscle fatigue, an assumption that can delay weaning.
A related problem occurs in those patients with COPD who have chronic CO2 retention and develop a compensatory elevated serum bicarbonate level. If their PaCO2 is normalized because of mechanical ventilation, their kidneys no longer need to compensate and their serum bicarbonate level may fall, albeit to a seemingly normal value. Although their arterial blood gas (ABG) may appear “normal,” prior to discontinuation of mechanical ventilation, these patients often fail extubation because of increasing respiratory acidosis, which causes dyspnea, tachypnea, and respiratory muscle fatigue. The preferred strategy for patients with COPD who have chronic CO2 retention is to use ventilator settings that maintain their PaCO2 levels at their baseline elevated values (see Appendix B). This approach also tends to result in sustained elevation of their serum HCO3– so that, when the patient’s pulmonary function has returned toward its baseline, weaning has a reasonable chance to be successful.
Chest Bellows and Peripheral Nervous System Problems
Respiratory Muscle Weakness
In some patients, respiratory muscle weakness occurs as a primary event (e.g., in some neuromuscular disorders; see Chapter 67). In other patients, decreased respiratory muscle strength may occur secondary to the effects of critical illness or respiratory failure (see Chapter 48 and Table 4.2), such as when fatigued respiratory muscles need additional time to recover (which may take up to 1 day). Moreover, with muscle disuse—as occurs with modes of mechanical support that do not require significant engagement of the patient’s respiratory muscles—or protein malnutrition, the muscles atrophy. Indeed, evidence of histologic myofiber atrophy has been demonstrated in the diaphragm of humans mechanically ventilated for less than 1 day, possibly because of altered proteolysis. In response to concerns regarding ICU-acquired weakness, several clinical trials were found that, compared to usual care, daily physical and occupational therapy coupled with sedative interruptions resulted in earlier weaning, as evidenced by a greater number of ventilator-free days and a larger number of patients who regained their baseline functional independence (Chapters 5 and 21) .
Changes in Chest Bellows Function
Various factors can limit lung or chest expansion. In postoperative states, both the architecture of the incisions as well as the degree of pain may limit expansion. Prior to modern pain management strategies (see Chapter 87), on the first postoperative day, a patient’s vital capacity often decreased to only ~25% of preoperative values after thoracotomy as well as after upper abdominal surgeries (e.g., open cholecystectomy). Other common factors that can alter expansion include the presence of a flail chest (resulting from trauma or closed chest cardiac resuscitation), pleural effusions, major atelectasis as well as abdominal distention (from air, ascites, dialysate, or edema). Similarly, intra-abdominal hypertension and abdominal compartment syndrome (Chapters 90 and 97)—often a result of extensive fluid resuscitation—can limit diaphragmatic excursion, thereby decreasing forced vital capacity (FVC) and increasing dead space ventilation.
Airways Problems
Upper Airway Injuries
An alternative approach to assess upper airway patency is to perform an “air leak” test—that is, to measure the air leak produced by deflation of the ET tube cuff—as the patient receives a defined tidal volume (e.g., 500 mL) during volume cycled mechanical ventilation. In patients with high risk for postextubation stridor, if the patient’s air leak around the deflated cuff is nil or modest (<100 mL per breath of 500 mL), one can assume that supraglottic obstruction may be present and delay extubation until after treatment of presumed laryngeal edema (i.e., IV dexamethasone and sitting the patient more upright in bed). However, because prospective studies have reported false positives (i.e., patients who had decreased air leaks but were extubated successfully) when applied to general ICU patients, some clinicians elect to limit its use to selected high-risk patients.
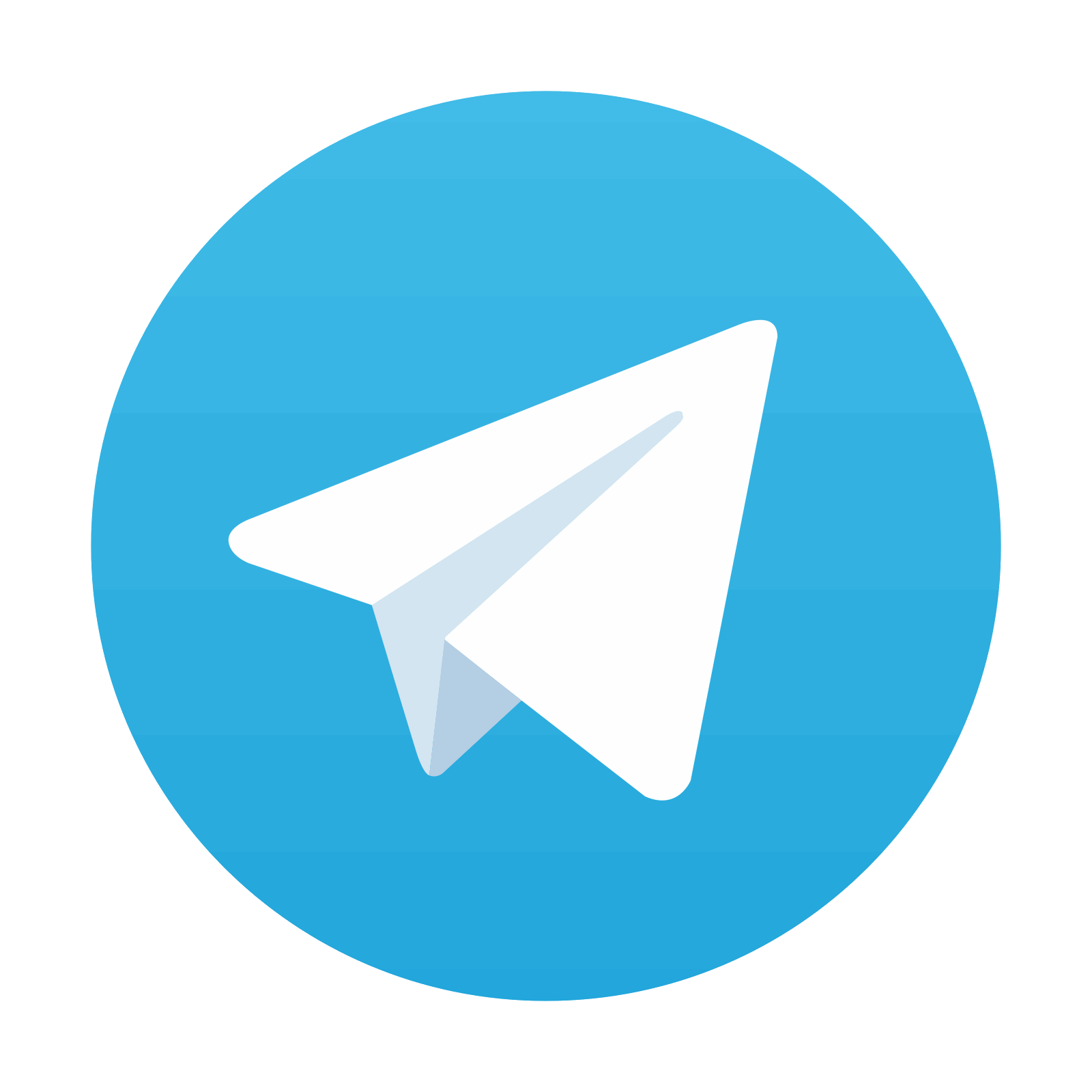
Stay updated, free articles. Join our Telegram channel

Full access? Get Clinical Tree
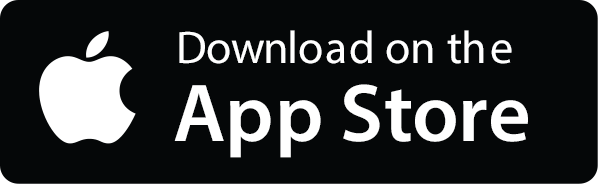
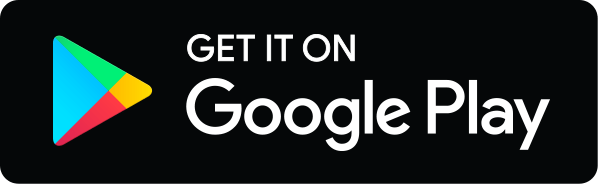