
The fact that total body clearance of propofol exceeds hepatic blood flow is consistent with extrahepatic clearance (pulmonary uptake and first-pass elimination, renal excretion) of propofol.14,16 Pulmonary uptake of propofol is significant and influences the initial availability of propofol. Although propofol can be transformed in the lungs to 2,6-diisopropyl-1,4-quiniol, most of the drug that undergoes pulmonary uptake during the first pass is released back into the circulation.17,18 Glucuronidation is the major metabolic pathway for propofol and uridine 5′-diphospho-glucuronosyltransferase isoforms are expressed in the kidneys and brain.
Despite the rapid clearance of propofol by metabolism, there is no evidence of impaired elimination in patients with cirrhosis of the liver. Plasma concentrations of propofol at the time of awakening are similar in alcoholic and normal patients.19 Extrahepatic elimination of propofol occurs during the anhepatic phase of orthotopic liver transplantation. Renal dysfunction does not influence the clearance of propofol despite the observation that nearly three-fourths of propofol metabolites are eliminated in urine in the first 24 hours.20 Patients older than 60 years of age exhibit a decreased rate of plasma clearance of propofol compared with younger adults. The rapid clearance of propofol confirms this drug can be administered as a continuous infusion during surgery without an excessive cumulative effect. Propofol readily crosses the placenta but is rapidly cleared from the neonatal circulation.21 The effect of instituting cardiopulmonary bypass on the plasma propofol concentration is unpredictable, with some studies reporting a decrease, whereas other observations fail to document any change.22
Clinical Uses
Propofol has become the induction drug of choice for many forms of anesthesia, especially when rapid and complete awakening is considered desirable.3 Continuous IV infusion of propofol, with or without other anesthetic drugs, has become a commonly used method for producing IV “conscious” sedation or as part of a balanced or total IV anesthetic.1,3 Administration of propofol as a continuous infusion may be used for sedation of patients in the ICU.2 In this regard, a 2% solution may be useful to decrease the volume of lipid emulsion administered with long-term sedation. A computer-controlled infusion pump is available to allow the clinician to select the propofol target concentration and the computer calculates the infusion rates that are necessary to achieve this target concentration based on the pharmacokinetics of propofol.23
Induction of Anesthesia
The induction dose of propofol in healthy adults is 1.5 to 2.5 mg/kg IV, with blood levels of 2 to 6 µg/mL producing unconsciousness depending on associated medications and the patient’s age. As with barbiturates, children require higher induction doses of propofol on a milligram per kilogram basis, presumably reflecting a larger central distribution volume and higher clearance rate. Elderly patients require a lower induction dose (25% to 50% decrease) as a result of a smaller central distribution volume and decreased clearance rate and increased pharmacodynamic activity.3 Awakening typically occurs at plasma propofol concentrations of 1.0 to 1.5 µg/mL. The complete awakening without residual CNS effects that is characteristic of propofol is the principal reason this drug has replaced thiopental for induction of anesthesia in many clinical situations. Thiopental is not currently available for use in the United States.
Intravenous Sedation
The short context-sensitive half-time of propofol, combined with the short effect-site equilibration time, make this a readily titratable drug for production of IV sedation.1 The prompt recovery without residual sedation and low incidence of nausea and vomiting make propofol particularly well suited to ambulatory conscious sedation techniques. The typical conscious sedation dose of 25 to 100 µg/kg/minute IV produces minimal analgesic and amnestic effects.3 In selected patients, midazolam or an opioid may be added to propofol for continuous IV sedation. A sense of well-being may accompany recovery from conscious sedation with propofol. When compared with anesthesia based on isoflurane, patients anesthetized with propofol reported less early postoperative pain.24 A conventional patient-controlled analgesia delivery system set to deliver 0.7 mg/kg doses of propofol with a 3-minute lockout period has been used as an alternative to continuous IV sedation techniques. Propofol has emerged as the agent of choice for sedation for brief gastrointestinal endoscopy procedures. So reliable are the pharmacologic properties of propofol that extensive design and testing have gone in to creation of a computer-assisted personalized sedation for upper endoscopy and colonoscopy, called SEDASYS. A comparative, multicenter randomized study concluded that this system could provide endoscopist/nurse teams a safe and effective means to administer propofol to effect minimal to moderate sedation during routine colonoscopy and esophagogastroduodenoscopy without the need for a trained anesthesia provider.25 The SEDASYS system received approval from the United States Food and Drug Administration in 2014 and is expected to be introduced in 2014.
Propofol has been administered as a sedative during mechanical ventilation in the ICU in a variety of patient populations including postoperative patients (cardiac surgery, neurosurgery) and patients with head injury.2 Propofol also provides control of stress responses and has anticonvulsant and amnestic properties. After cardiac surgery, propofol sedation appears to modulate postoperative hemodynamic responses by decreasing the incidence and severity of tachycardia and hypertension.26 Increasing metabolic acidosis, lipemic plasma, bradycardia, and progressive myocardial failure has been described, particularly in children who were sedated with propofol during management of acute respiratory failure in the ICU.27
Maintenance of Anesthesia
The typical dose of propofol for maintenance of anesthesia is 100 to 300 µg/kg/minute IV, often in combination with a short-acting opioid.3 General anesthesia that includes propofol is typically associated with minimal postoperative nausea and vomiting, and awakening is prompt, with minimal residual sedative effects.
Nonhypnotic Therapeutic Applications
In addition to its clinical application as an IV induction drug, propofol has been shown to have beneficial effects that were not anticipated when the drug was initially introduced in 1989.28
Antiemetic Effects
The incidence of postoperative nausea and vomiting is decreased when propofol is administered, regardless of the anesthetic technique.28 Subhypnotic doses of propofol (10 to 15 mg IV) may be used in the postanesthesia care unit to treat nausea and vomiting, particularly if it is not of vagal origin. In the postoperative period, the advantage of propofol is its rapid onset of action and the absence of serious side effects. Propofol is generally efficacious in treating postoperative nausea and vomiting at plasma concentrations that do not produce significant sedation. Simulations indicate that antiemetic plasma concentrations of propofol are achieved by a single IV dose of 10 mg followed by 10 µg/kg/minute.29 Propofol in subhypnotic doses is effective against chemotherapy-induced nausea and vomiting. When administered to induce and maintain anesthesia, it is more effective than ondansetron in preventing postoperative nausea and vomiting.30
Propofol has a profile of CNS depression that differs from other anesthetic drugs. In contrast to thiopental, for example, propofol uniformly depresses CNS structures, including subcortical centers. Most drugs of known antiemetic efficacy exert this effect via subcortical structures, and it is possible that propofol modulates subcortical pathways to inhibit nausea and vomiting or produces a direct depressant effect on the vomiting center. Nevertheless, the mechanisms mediating the antiemetic effects of propofol remain unknown. An antiemetic effect of propofol based on inhibition of dopaminergic activity is unlikely given that subhypnotic doses of propofol fail to increase plasma prolactin concentrations. A rapid and distinct increase in plasma prolactin concentrations is characteristic of drugs that block the dopaminergic system.31 Subhypnotic doses of propofol that are effective as an antiemetic do not inhibit gastric emptying and propofol is not considered a prokinetic drug.32
Antipruritic Effects
Propofol, 10 mg IV, is effective in the treatment of pruritus associated with neuraxial opioids or cholestasis.33 The mechanism of the antipruritic effect may be related to the drug’s ability to depress spinal cord activity. In this regard, there is evidence that intrathecal opioids produce pruritus by segmental excitation within the spinal cord.
Anticonvulsant Activity
Propofol possesses antiepileptic properties, presumably reflecting GABA-mediated presynaptic and postsynaptic inhibition of chloride ion channels. In this regard, propofol in doses of greater than 1 mg/kg IV decreases seizure duration 35% to 45% in patients undergoing electroconvulsive therapy.34
Attenuation of Bronchoconstriction
Compared with thiopental, propofol decreases the prevalence of wheezing after induction of anesthesia and tracheal intubation in healthy and asthmatic patients (Fig. 5-2).35 However, a newer formulation of propofol uses metabisulfite as a preservative. Metabisulfite may cause bronchoconstriction in asthmatic patients. In an animal model, propofol without metabisulfite attenuated vagal nerve stimulation–induced bronchoconstriction, whereas propofol with metabisulfite did not attenuate vagally or methacholine-induced bronchoconstriction and metabisulfite alone caused increases in airway responsiveness.36 Following tracheal intubation, in patients with a history of smoking, airway resistance was increased more following the administration of propofol containing metabisulfite than ethylenediaminetetraacetic acid (EDTA).37 Therefore, the preservative used for propofol can have effects on its ability to attenuate bronchoconstriction. Nevertheless, propofol-induced bronchoconstriction has been described in patients with allergy histories. The formulation of propofol administered to these patients was Diprivan containing soybean oil, glycerin, yolk lecithin, and sodium edetate.38

Analgesia
Propofol does not relieve acute nociceptive pain. However in animal models, low-dose propofol equivalent to antiemetic concentrations earlier was highly effective in relieving nociceptive responses to neuropathic pain.39
Effects on Organ Systems
Central Nervous System
Propofol decreases cerebral metabolic rate for oxygen (CMRO2), cerebral blood flow, and intracranial pressure (ICP).40,41 Administration of propofol to produce sedation in patients with intracranial space-occupying lesions does not increase ICP.42 However, large dose propofol may decrease systemic blood pressure sufficiently to also decrease cerebral perfusion pressure. Cerebrovascular autoregulation in response to changes in systemic blood pressure and reactivity of the cerebral blood flow to changes in PaCO2 are not affected by propofol. Cerebral blood flow velocity changes in parallel with changes in PaCO2 in the presence of propofol and midazolam (Fig. 5-3).43 Propofol produces cortical electroencephalographic (EEG) changes that are similar to those of thiopental, including the ability of high doses to produce burst suppression.44 Cortical somatosensory evoked potentials as used for monitoring spinal cord function are not significantly modified in the presence of propofol alone but the addition of nitrous oxide or a volatile anesthetic results in decreased amplitude.45 Propofol does not interfere with the adequacy of electrocorticographic recordings during awake craniotomy performed for the management of refractory epilepsy, provided administration is discontinued at least 15 minutes before recording.46 At equal levels of sedation, propofol produces the same degree of memory impairment as midazolam, whereas thiopental has less memory effect and fentanyl has none.47

Development of tolerance to drugs that depress the CNS is a common finding, occurring with repeated exposure to opioids, sedative-hypnotic drugs, ketamine, and nitrous oxide. However, tolerance to propofol does not develop in children undergoing repeated exposure to the drug during radiation therapy.48
Cardiovascular System
Propofol produces decreases in systemic blood pressure, which are greater than those evoked by comparable doses of thiopental (Fig. 5-4).49 These decreases in blood pressure are often accompanied by corresponding changes in cardiac output and systemic vascular resistance. The relaxation of vascular smooth muscle produced by propofol is primarily due to inhibition of sympathetic vasoconstrictor nerve activity.50 A negative inotropic effect of propofol may result from a decrease in intracellular calcium availability secondary to inhibition of trans-sarcolemmal calcium influx. Stimulation produced by direct laryngoscopy and intubation of the trachea reverses the blood pressure effects of propofol. Propofol also effectively blunts the hypertensive response to placement of a laryngeal mask airway. The impact of propofol on desflurane-mediated sympathetic nervous system activation is unclear. In one report, propofol 2 mg/kg IV blunted the increase in epinephrine concentration, which accompanied a sudden increase in the delivered desflurane concentration but did not attenuate the transient cardiovascular response.51 Conversely, in another report, induction of anesthesia with propofol, but not etomidate, blunted the sympathetic nervous system activation and systemic hypertension associated with the introduction of rapidly increasing inhaled concentrations of desflurane.52 The blood pressure effects of propofol may be exaggerated in hypovolemic patients, elderly patients, and patients with compromised left ventricular function. Adequate hydration before rapid IV administration of propofol is recommended to minimize the blood pressure reduction.

Addition of nitrous oxide does not alter the cardiovascular effects of propofol. The pressor response to ephedrine is augmented by propofol (Fig. 5-5).53

Despite decreases in systemic blood pressure, heart rate typically remains unchanged. Baroreceptor reflex control of heart rate may be depressed by propofol.54 However, bradycardia and asystole have been observed after induction of anesthesia with propofol, resulting in the occasional recommendation that anticholinergic drugs be administered when vagal stimulation is likely to occur in association with administration of propofol (see the section “Bradycardia-Related Death”). Propofol may decrease sympathetic nervous system activity to a greater extent than parasympathetic nervous system activity, resulting in a predominance of parasympathetic activity.1 Propofol does not alter sinoatrial or atrioventricular node function in normal patients or in patients with Wolff-Parkinson-White syndrome, thus making it an acceptable drug to administer during ablative procedures.55,56 Nevertheless, there is a case report of a patient with Wolff-Parkinson-White syndrome in whom δ waves on the electrocardiogram disappeared during infusion of propofol.57 Unlike sevoflurane, propofol does not prolong the QTc interval on the electrocardiogram.58
Bradycardia-Related Death
Profound bradycardia and asystole after administration of propofol have been described in healthy adult patients, despite prophylactic anticholinergics.59–62 The risk of bradycardia-related death during propofol anesthesia has been estimated to be 1.4 in 100,000. Propofol anesthesia, compared with other anesthetics, increases the incidence of the oculocardiac reflex in pediatric strabismus surgery, despite prior administration of anticholinergics.63
Heart rate responses to IV administration of atropine are attenuated in patients receiving propofol compared with awake patients (Fig. 5-6).64 This decreased responsiveness to atropine cannot be effectively overcome by larger doses of atropine suggesting that propofol may induce suppression of sympathetic nervous system activity. Treatment of propofol-induced bradycardia may require treatment with a direct β agonist such as isoproterenol.

Lungs
Propofol produces dose-dependent depression of ventilation, with apnea occurring in 25% to 35% of patients after induction of anesthesia with propofol.65 Opioids administered with the preoperative medication enhances ventilatory depressant. Painful surgical stimulation is likely to counteract the ventilatory depressant effects of propofol. A maintenance infusion of propofol decreases tidal volume and frequency of breathing. The ventilatory response to arterial hypoxemia are also decreased by propofol due to an effect at the central chemoreceptors.66 Likewise, propofol at sedative doses significantly decreases the slope and causes a downward shift of the ventilatory response curve to hypoxia.67 Hypoxic pulmonary vasoconstriction seems to remain intact in patients receiving propofol.
Hepatic and Renal Function
Propofol does not normally affect hepatic or renal function as reflected by measurements of liver transaminase enzymes or creatinine concentrations. Prolonged infusions of propofol have been associated with hepatocellular injury accompanied by lactic acidosis, bradydysrhythmias, and rhabdomyolysis as part of the propofol infusion syndrome described in the following texts. In rare instances, presumed propofol-induced hepatocellular injury following uneventful anesthesia and surgery has been described.68 Prolonged infusions of propofol may also result in excretion of green urine, reflecting the presence of phenols in the urine. This discoloration does not alter renal function. Urinary uric acid excretion is increased after administration of propofol and may manifest as cloudy urine when the uric acid crystallizes in the urine under conditions of low pH and temperature.20 This cloudy urine is not considered to be detrimental or indicative of adverse renal effects of propofol.
Intraocular Pressure
Laparoscopic surgery is associated with increased intraocular pressure and some consider laparoscopic surgery with the head down position a risk in the presence of preexisting ocular hypertension. In this regard, propofol is associated with significant decreases in intraocular pressure that occur immediately after induction of anesthesia and are sustained during tracheal intubation.1 Total IV anesthesia with propofol for laparoscopic surgery was associated with lower intraocular pressures than in patients undergoing similar surgery with isoflurane anesthesia (Fig. 5-7).69

Coagulation
Propofol does not alter tests of coagulation or platelet function. This is reassuring because the emulsion in which propofol is dispensed resembles intralipid, which has been associated with alterations in blood coagulation. However, propofol inhibits platelet aggregation that is induced by proinflammatory lipid mediators including thromboxane A2 and platelet-activating factor.70
Other Side Effects
Side effects of propofol may reflect the parent drug or actions attributed to the oil-in-water emulsion formulation. For example, some of the side effects of propofol (bradycardia, risk of infection, pain on injection, hypertriglyceridemia with prolonged administration, potential for pulmonary embolism) are believed to be due in large part to the lipid emulsion formulation.7,8
Allergic Reactions
Allergenic components of propofol include the phenyl nucleus and diisopropyl side chain.71 Patients who develop evidence of anaphylaxis on first exposure to propofol may have been previously sensitized to the diisopropyl radical, which is present in many dermatologic preparations. Likewise, the phenol nucleus is common to many drugs. Indeed, anaphylaxis to propofol during the first exposure to this drug has been observed, especially in patients with a history of other drug allergies, often to neuromuscular blocking drugs.72 Propofol-induced bronchoconstriction has been described in patients with allergy histories.38 The formulation of propofol administered to these patients was Diprivan containing soybean oil, glycerin, yolk lecithin, and sodium edetate.
Lactic Acidosis
Lactic acidosis (“propofol infusion syndrome”) has been described in pediatric and adult patients receiving prolonged high-dose infusions of propofol (>75 µg/kg/ minute) for longer than 24 hours.73,74 Severe, refractory, and fatal bradycardia in children in the ICU has been observed with long-term propofol sedation.75,76 Even short-term infusions of propofol (Diprivan) for surgical anesthesia have been associated with development of metabolic acidosis.77,78 Unexpected tachycardia occurring during propofol anesthesia should prompt laboratory evaluation for possible metabolic (lactic) acidosis. Measurement of arterial blood gases and serum lactate concentrations is recommended. Documentation of an increased ion gap is useful but will take time and delay treatment, which includes prompt discontinuation of propofol administration.79 Metabolic acidosis in its early stages is reversible with discontinuation of propofol administration although cardiogenic shock requiring assistance with extracorporeal membrane oxygenation has been described in a patient receiving a prolonged propofol infusion (Diprivan) for a craniotomy.80
The mechanism for sporadic propofol-induced metabolic acidosis is unclear but may reflect poisoning (cytopathic hypoxia) of the electron transport chain and impaired oxidation of long chain fatty acids by propofol or a propofol metabolite in uniquely susceptible patients.81 Indeed, this propofol infusion syndrome mimics the mitochondrial myopathies, in which there are specific defects in the mitochondrial respiratory chain associated with specific mitochondrial DNA abnormalities, resulting in abnormal lipid metabolism in cardiac and skeletal muscles. These individuals, who are probably genetically susceptible, remain asymptomatic until a triggering event (sepsis, malnutrition) intervenes.
The differential diagnosis when propofol-induced lactic acidosis is suspected includes hyperchloremic metabolic acidosis associated with large volume infusions of 0.9% saline and metabolic acidosis associated with excessive generation of organic acids, such as lactate and ketones (diabetic acidosis, release of a tourniquet). Measurement of the anion gap and individual measurements of anions and organic acids will differentiate hyperchloremic metabolic acidosis from lactic acidosis.
Proconvulsant Activity
The majority of reported propofol-induced “seizures” during induction of anesthesia or emergence from anesthesia reflect spontaneous excitatory movements of subcortical origin.28 These responses are not thought to be due to cortical epileptic activity. Prolonged myoclonus associated with meningismus has been associated with propofol administration.82 The incidence of excitatory movements and associated ECG changes are low after the administration of propofol.83 Propofol resembles thiopental in that it does not produce seizure activity on the EEG when administered to patients with epilepsy, including those undergoing cortical resection.44 There appears to be no reason to avoid propofol for sedation, induction, and maintenance of anesthesia in patients with known seizures.10
Abuse Potential
Intense dreaming activity, amorous behavior, and hallucinations have been reported during recovery from low-dose infusions of propofol.28 Addiction to virtually all opioids and hypnotics, including propofol, has been described.84,85 The death of music pop star Michael Jackson in 2009 from an overdose of propofol he was receiving as a sleep aid has recently brought the dangers of propofol misuse to public attention.86
Bacterial Growth
Propofol strongly supports the growth of Escherichia coli and Pseudomonas aeruginosa, whereas the solvent (Intralipid) appears to be bactericidal for these same organisms and bacteriostatic for Candida albicans.87 Clusters of postoperative surgical infections manifesting as temperature elevations have been attributed to extrinsic contamination of propofol.88,89 For this reason, it is recommended that (a) an aseptic technique be used in handling propofol as reflected by disinfecting the ampule neck surface or vial rubber stopper with 70% isopropyl alcohol; (b) the contents of the ampule containing propofol should be withdrawn into a sterile syringe immediately after opening and administered promptly; and (c) the contents of an opened ampule must be discarded if they are not used within 6 hours. In the ICU, the tubing and any unused portion of propofol must be discarded after 12 hours. Despite these concerns, there is evidence that when propofol is aseptically drawn into an uncapped syringe, it will remain sterile at room temperature for several days.90 Given the cost of propofol, some have questioned the logic of discarding unused drug at the end of an anesthetic or 6 hours, whichever occurs sooner.3
Antioxidant Properties
Propofol has potent antioxidant properties that resemble those of the endogenous antioxidant vitamin E.91,92 Like vitamin E, propofol contains a phenolic hydroxyl group that scavenges free radicals and inhibits lipid peroxidation. A neuroprotective effect of propofol may be at least partially related to the antioxidant potential of propofol’s phenol ring structure. For example, propofol reacts with lipid peroxyl radicals and thus inhibits lipid peroxidation by forming relatively stable propofol phenoxyl radicals. In addition, propofol also scavenges peroxynitrite, which is one of the most potent reactive metabolites for the initiation of lipid peroxidation. Because peroxynitrite is a potent bactericidal agent, it is likely that the peroxynitrite-scavenging activity of propofol contributes to this anesthetic’s known ability to suppress phagocytosis.93 Conversely, propofol might be beneficial in disease states, such as acute lung injury, in which peroxynitrite formation is thought to play an important role.94
Reintroduction of molecular oxygen into previously ischemic tissues (removal of an aortic cross-clamp) can further damage partially injured cells (reperfusion injury). Oxygen leads to the formation of free oxygen radicals, which react with polyunsaturated fatty acids of cell membranes resulting in disruption of cell membranes. Myocardial cell injury can cause postischemic dysfunction, myocardial stunning, and reperfusion cardiac dysrhythmias. Propofol strongly attenuates lipid peroxidation during coronary artery bypass graft surgery.95
Pain on Injection
Pain on injection is the most commonly reported adverse event associated with propofol administration to awake patients. This unpleasant side effect of propofol occurs in less than 10% of patients when the drug is injected into a large vein rather than a dorsum vein on the hand. Preceding the propofol with (using the same injection site as for propofol) 1% lidocaine or by prior administration of a potent short-acting opioid decreases the incidence of discomfort experienced by the patient. The incidence of thrombosis or phlebitis is usually less than 1%. Changing the composition of the carrier fat emulsion for propofol to long and medium chain triglycerides decreases the incidence of pain on injection.96
Accidental intraarterial injection of propofol has been described as producing severe pain but no vascular compromise.97 In an animal model, propofol-exposed arteries showed no changes in the vascular smooth muscle, and the endothelium was not damaged.98
Airway Protection
Inhaled and injected anesthetic drugs alter pharyngeal function with the associated risk of impaired upper airway protection and pulmonary aspiration. Subhypnotic concentrations of propofol, isoflurane, and sevoflurane decrease pharyngeal contraction force.99
Miscellaneous Effects
Propofol does not trigger malignant hyperthermia and has been administered to patients with hereditary coproporphyria without incident.100–102 Secretion of cortisol is not influenced by propofol, even when administered for prolonged periods in the ICU. Temporary abolition of tremors in patients with Parkinson’s disease may occur after the administration of propofol.103 For this reason, propofol may not be ideally suited for patients undergoing stereotactic neurosurgery during which the symptom is required to identify the correct anatomic location.
Etomidate
Etomidate is a carboxylated imidazole–containing compound that is chemically unrelated to any other drug used for the IV induction of anesthesia.104 The imidazole nucleus renders etomidate, like midazolam, water soluble at an acidic pH and lipid soluble at physiologic pH.
Commercial Preparation
The original formulation of etomidate included 35% propylene glycol (pH 6.9) contributing to a high incidence of pain during IV injection and occasional venous irritation. This has been changed to a fat emulsion, which has virtually abolished pain on injection and venous irritation, whereas the incidence of myoclonus remains unchanged. An oral formulation of etomidate for transmucosal delivery has been shown to produce dose-dependent sedation.105 Administration through the oral mucosa results in direct systemic absorption while bypassing hepatic metabolism. As a result, higher blood concentrations are achieved more rapidly compared with drug that is administered by mouth.
Mechanism of Action
Etomidate is unique among injected and inhaled anesthetics in being administered as a single isomer.104 The anesthetic effect of etomidate resides predominantly in the R(+) isomer, which is approximately five times as potent as the S(−) isomer. In contrast to barbiturates, etomidate appears to be relatively selective as a modulator of GABAA receptors. Stereoselectivity of etomidate supports the concept that GABAA receptors are the site of action of etomidate. Etomidate exerts its effects on GABAA receptors by binding directly to a specific site or sites on the protein and enhancing the affinity of the inhibitory neurotransmitter (GABA) for these receptors.106 Antagonism of steroid-induced psychosis by etomidate is consistent with enhancement of GABA receptor function by this anesthetic drug.107 Etomidate is not known to modulate other ligand-gated ion channels in the brain at clinically relevant concentrations.
Pharmacokinetics
The volume of distribution (Vd) of etomidate is large, suggesting considerable tissue uptake (see Table 5-1). Distribution of etomidate throughout body water is favored by its moderate lipid solubility and existence as a weak base (pK 4.2, pH 8.2, 99% unionized at physiologic pH). Etomidate penetrates the brain rapidly, reaching peak levels within 1 minute after IV injection. About 76% of etomidate is bound to albumin independently of the plasma concentration of the drug. Decreases in plasma albumin concentrations, however, result in dramatic increases in the unbound pharmacologically active fraction of etomidate in the plasma. Prompt awakening after a single dose of etomidate principally reflects the redistribution of the drug from brain to inactive tissue sites. Rapid metabolism is also likely to contribute to prompt recovery.
Metabolism
Etomidate is rapidly metabolized by hydrolysis of the ethyl ester side chain to its carboxylic acid ester, resulting in a water-soluble, pharmacologically inactive compound. Hepatic microsomal enzymes and plasma esterases are responsible for this hydrolysis. Hydrolysis is nearly complete, as evidenced by recovery of less than 3% of an administered dose of etomidate as unchanged drug in urine. About 85% of a single IV dose of etomidate can be accounted for as the carboxylic acid ester metabolite in urine, whereas another 10% to 13% is present as this metabolite in the bile. Overall, the clearance of etomidate is about five times that for thiopental; this is reflected as a shorter elimination half-time of 2 to 5 hours. Likewise, the context-sensitive half-time of etomidate is less likely to be increased by continuous infusion as compared with thiopental.
Cardiopulmonary Bypass
Institution of hypothermic cardiopulmonary bypass causes an initial decrease of about 34% in the plasma etomidate concentration that then returns to within 11% of the prebypass value only to be followed by a further decrease with rewarming.22 The return of the plasma concentration toward prebypass levels is attributed to decreased metabolism, and the subsequent decrease on rewarming is attributed to increased metabolism. In addition, hepatic blood flow changes during cardiopulmonary bypass may alter metabolism, as etomidate is a high–hepatic extraction drug.
Clinical Uses
Etomidate may be viewed as an alternative to propofol or barbiturates for the IV induction of anesthesia, especially in the presence of an unstable cardiovascular system. After a standard induction dose of 0.2 to 0.4 mg/kg IV, the onset of unconsciousness occurs within one arm-to-brain circulation time. Involuntary myoclonic movements are common during the induction period as a result of alteration in the balance of inhibitory and excitatory influences on the thalamocortical tract. The frequency of this myoclonic-like activity can be attenuated by prior administration of an opioid. Awakening after a single IV dose of etomidate is more rapid than after barbiturates, and there is little or no evidence of a hangover or cumulative drug effect. Recovery of psychomotor function after administration of etomidate is intermediate between that of methohexital and thiopental. The duration of action is prolonged by increasing the dose of etomidate or administering the drug as a continuous infusion. As with barbiturates, analgesia is not produced by etomidate. For this reason, administration of an opioid before induction of anesthesia with etomidate may be useful to blunt the hemodynamic responses evoked by direct laryngoscopy and tracheal intubation. Etomidate, 0.15 to 0.3 mg/kg IV, has minimal effects on the duration of electrically induced seizures and thus may serve as an alternative to drugs that decrease the duration of seizures (propofol, thiopental) in patients undergoing electroconvulsive therapy.34
The principal limiting factor in the clinical use of etomidate for induction of anesthesia is the ability of this drug to transiently depress adrenocortical function (see the section “Adrenocortical Suppression”). It is widely viewed that postoperative nausea and vomiting is increased in patients receiving etomidate for induction of anesthesia.108 Nevertheless, comparison of etomidate with propofol did not document an increased incidence of nausea and vomiting in the first 24 hours after surgery in patients receiving etomidate.109
Side Effects
Central Nervous System
Etomidate is a potent direct cerebral vasoconstrictor that decreases cerebral blood flow and CMRO2 35% to 45%.110 As a result, previously increased ICP is lowered by etomidate. These effects of etomidate are similar to those changes produced by comparable doses of thiopental. Suppression of adrenocortical function limits the clinical usefulness for long-term treatment of intracranial hypertension (see the section “Adrenocortical Suppression”).
Etomidate produces a pattern on the EEG that is similar to thiopental. However, the frequency of excitatory spikes on the EEG is greater with etomidate than with thiopental and methohexital, suggesting caution in administration of etomidate to patients with a history of seizures.83 Like methohexital, etomidate may activate seizure foci, manifesting as fast activity on the EEG.111 For this reason, etomidate should also be used with caution in patients with focal epilepsy. Conversely, this characteristic has been observed to facilitate localization of seizure foci in patients undergoing cortical resection of epileptogenic tissue. Etomidate also possesses anticonvulsant properties and has been used to terminate status epilepticus. Etomidate has been observed to augment the amplitude of somatosensory evoked potentials, making monitoring of these responses more reliable.112
Cardiovascular System
Cardiovascular stability is characteristic of induction of anesthesia with 0.3 mg/kg IV of etomidate. After this dose of etomidate, there are minimal changes in heart rate, stroke volume, or cardiac output, whereas mean arterial blood pressure may decrease up to 15% because of decreases in systemic vascular resistance. The decrease in systemic blood pressure in parallel with changes in systemic vascular resistance suggests that administration of etomidate to acutely hypovolemic patients could result in sudden hypotension. When an induction dose of etomidate is 0.45 mg/kg IV, significant decreases in systemic blood pressure and cardiac output may occur.113 The cardiovascular effects of etomidate and thiopental are similar when continuously infused in patients with severe valvular heart disease.114
Effects of etomidate on myocardial contractility are important to consider, as this drug has been proposed for induction of anesthesia in patients with little or no cardiac reserve. It is difficult to document anesthetic-induced negative inotropic effects in vivo because of concurrent changes in preload, afterload, sympathetic nervous system activity, and baroreceptor reflex activity. Therefore, direct effects of anesthetics on intrinsic myocardial contractility may be more accurately assessed in vitro. In this regard, etomidate causes dose-dependent decreases in developed tension in isolated cardiac muscle obtained from patients undergoing coronary artery bypass graft operations or cardiac transplantation (Fig. 5-8).115 This depression was reversible with β-adrenergic stimulation. Nevertheless, concentrations required to produce these negative inotropic effects are in excess of those achieved with clinical use. In this regard, etomidate may differ from most other IV anesthetics in that depressive effects on myocardial contractility are minimal at concentrations needed for the production of anesthesia. Hepatic and renal functions tests are not altered by etomidate. Intraocular pressure is decreased by etomidate to a similar degree as by thiopental. Etomidate does not result in detrimental effects when accidentally injected into an artery.

Ventilation
The depressant effects of etomidate on ventilation seem to be less than those of barbiturates, although apnea may occasionally accompany a rapid IV injection of the drug.116 In the majority of patients, etomidate-induced decreases in tidal volume are offset by compensatory increases in the frequency of breathing. These effects on ventilation are transient, lasting only 3 to 5 minutes. Etomidate may stimulate ventilation independently of the medullar centers that normally respond to carbon dioxide. For this reason, etomidate may be useful when maintenance of spontaneous ventilation is desirable. Depression of ventilation may be exaggerated when etomidate is combined with inhaled anesthetics or opioids during continuous infusion techniques.
Pain on Injection
Pain on injection and venous irritation has been virtually eliminated with use of etomidate in a lipid emulsion vehicle rather than propylene glycol.
Myoclonus
Commonly administered IV anesthetics can cause excitatory effects that may manifest as spontaneous movements, such as myoclonus, dystonia, and tremor. These spontaneous movements, particularly myoclonus, occur in 50% to 80% of patients receiving etomidate in the absence of premedication.83 In one report, 87% of patients receiving etomidate developed excitatory effects, of which 69% were myoclonic. Multiple spikes appeared on the EEG of 22% of these patients.83 In this same report, the frequency of excitatory effects was 17% after thiopental, 13% after methohexital, and 6% after propofol, and none of the patients treated with other drugs developed myoclonus with spike activity on the EEG.83 Inclusion of atropine in the preoperative medication may suppress spike activity on the EEG associated with the administration of etomidate. Prior administration of an opioid (fentanyl, 1 to 2 µg/kg IV) or a benzodiazepine may decrease the incidence of myoclonus associated with administration of etomidate. Furthermore, the incidence and intensity of myoclonus following the administration of etomidate is dose-related and suppressed by pretreatment with small doses of etomidate (0.03 to 0.075 mg/kg IV) before administration of the induction dose.117
The mechanism of etomidate-induced myoclonus appears to be disinhibition of subcortical structures that normally suppress extrapyramidal motor activity. In many patients, excitatory movements are coincident with the early slow phase of the EEG, which corresponds to the beginning of deep anesthesia.83 It is possible that myoclonus could occur on awakening if the extrapyramidal system emerged more quickly than the cortex that inhibits it.118 The fact that etomidate-induced myoclonic activity may be associated with seizure activity on the EEG suggests caution in the use of this drug for the induction of anesthesia in patients with a history of seizure activity.83 Conversely, others have not documented seizure-like activity on the EEG in association with etomidate-induced myoclonus.117
Adrenocortical Suppression
Etomidate causes adrenocortical suppression by producing a dose-dependent inhibition of the conversion of cholesterol to cortisol (Fig. 5-9).119,120 The specific enzyme inhibited by etomidate appears to be 11-β-hydroxylase as evidenced by the accumulation of 11-deoxycorticosterone.121 This enzyme inhibition lasts 4 to 8 hours after an induction dose of etomidate. Conceivably, patients experiencing sepsis or hemorrhage and who might require an intact cortisol response would be at a disadvantage should etomidate be administered.122 Conversely, suppression of adrenocortical function could be considered desirable from the standpoint of “stress-free” anesthesia. Nevertheless, in at least one report, it was not possible to demonstrate a difference in the plasma concentrations of cortisol, corticosterone, or adrenocorticotrophic hormone in patients receiving a single dose of etomidate or thiopental.123 In a retrospective study of more than 3,000 cardiac surgical patients who received etomidate for induction of anesthesia, there was no evidence to suggest that etomidate exposure was associated with severe hypotension, longer mechanical ventilation hours, longer length of hospital stay, or in-hospital mortality.124 In stark contrast, another large scale retrospective study demonstrated that anesthetic induction with etomidate, rather than propofol, was associated with increased 30-day mortality and cardiovascular morbidity after noncardiac surgery.125 The clinical benefit of minimizing cardiac suppression should be carefully weighed against the potential for worsened long-term outcomes when using propofol in high-risk patients.

Allergic Reactions
The incidence of allergic reactions following administration of etomidate is very low.126 When reactions have occurred, it is difficult to separate the role of etomidate from other concomitantly administered drugs (neuromuscular blocking drugs) that are more likely to evoke histamine release than etomidate.
Benzodiazepines
Benzodiazepines are drugs that exert, in slightly varying degrees, five principal pharmacologic effects: anxiolysis, sedation, anticonvulsant actions, spinal cord–mediated skeletal muscle relaxation, and anterograde amnesia (acquisition or encoding of new information).127 The amnestic potency of benzodiazepines is greater than their sedative effects resulting in a longer duration of amnesia than sedation. Stored information (retrograde amnesia) is not altered by benzodiazepines.128 Benzodiazepines do not produce adequate skeletal muscle relaxation for surgical procedures nor does their use influence the required dose of neuromuscular blocking drugs. The frequency of anxiety and insomnia in clinical practice combined with the efficacy of benzodiazepines has led to widespread use of these drugs. For example, it is estimated that 4% of the population uses “sleeping pills” sometime during a given year, and 0.4% of the population uses hypnotics for more than a year.129 Although benzodiazepines are effective for the treatment of acute insomnia, their use for management of chronic insomnia is decreasing. Compared with barbiturates, benzodiazepines have fewer tendencies to produce tolerance, less potential for abuse, a greater margin of safety, and elicit fewer and less serious drug interactions. Unlike barbiturates, benzodiazepines do not induce hepatic microsomal enzymes. Benzodiazepines are intrinsically far less addicting than opioids, cocaine, amphetamines, or barbiturates.
Midazolam is the most commonly used benzodiazepine in the perioperative period. Furthermore, the context-sensitive half-times for diazepam and lorazepam are prolonged; therefore, only midazolam is likely to be used for prolonged administration when prompt recovery is desired. However, the longer context-sensitive half-time of lorazepam makes this drug an attractive choice to facilitate sedation of patients in critical care environments. Unlike other drugs administered IV to produce CNS effects, benzodiazepines, as a class of drugs, are unique in the availability of a specific pharmacologic antagonist, flumazenil.
Structurally, benzodiazepines are similar and share many active metabolites.
Mechanism of Action
Benzodiazepines appear to produce all their pharmacologic effects by facilitating the actions of GABA.130 Benzodiazepines do not activate the GABAA receptors but rather enhance the affinity of the receptors for GABA (Fig. 5-10).131 As a result of increased affinity for GABA, there is more frequent channel openings, resulting in increased chloride conductance and hyperpolarization of the postsynaptic cell membrane. The postsynaptic neurons are thus rendered more resistant to excitation. This resistance to excitation is presumed to be the mechanism by which benzodiazepines produce anxiolysis, sedation, anterograde amnesia, alcohol potentiation, and anticonvulsant and skeletal muscle relaxant effects.

Benzodiazepines interact with a site located between the α and γ subunits of the GABAA receptor. The γ subunit is required for benzodiazepine binding. The α1– and α5-containing GABAA receptors are important for sedation, whereas anxiolytic activity is due to interaction with α2 and α5 subunit–containing receptors.132,133 The α1-containing GABAA receptors are the most abundant receptor subtypes accounting for approximately 60% of GABAA receptors in the brain. α2 Subunits have more restricted expression, principally in the hippocampus and amygdala. The α5-containing GABAA receptors are principally extrasynaptic and are responsible for modulation of the resting membrane potential. This anatomic distribution of receptors is consistent with the minimal effects of these drugs outside the CNS (minimal circulatory effects). In the future, it may be possible to design benzodiazepines that selectively activate specific GABAA receptor types to produce anxiolysis without sedation.
The GABAA receptor is a large macromolecule that contains physically separate binding sites (principally α, β, and γ subunits) not only for GABA and the benzodiazepines but also barbiturates, etomidate, propofol, neurosteroids, and alcohol. Acting on a single receptor at different binding sites, the benzodiazepines, barbiturates, and alcohol can produce synergistic effects to increase GABAA receptor–mediated inhibition in the CNS. This property explains the pharmacologic synergy of these substances and, likewise, the risks of combined overdose, which can produce life-threatening CNS depression. This synergy is also the basis for pharmacologic cross-tolerance between these different classes of drugs and is consistent with the clinical use of benzodiazepines as the first-choice drugs for detoxication from alcohol. Conversely, benzodiazepines have a built-in ceiling effect that prevents them from exceeding the physiologic maximum of GABA inhibition. The low toxicity of the benzodiazepines and their corresponding clinical safety is attributed to this limitation of their effect on GABAergic neurotransmission.
Differences in the onset and duration of action among commonly administered benzodiazepines reflect differences in potency (receptor binding affinity), lipid solubility (ability to cross the blood–brain barrier and redistribute to peripheral tissues), and pharmacokinetics (uptake, distribution, metabolism, and elimination). All benzodiazepines are highly lipid soluble and are highly bound to plasma proteins, especially albumin. Hypoalbuminemia owing to hepatic cirrhosis or chronic renal failure may increase the unbound fraction of benzodiazepines, resulting in enhanced clinical effects produced by these drugs. Following oral administration, benzodiazepines are highly absorbed from the gastrointestinal tract and after IV injection, they rapidly enter the CNS and other highly perfused organs.
Nucleoside Transporter Systems
Benzodiazepines decrease adenosine degradation by inhibiting the nucleoside transporter, which is the principal mechanism whereby the effect of adenosine is terminated through reuptake into cells.134 Adenosine is an important regulator of cardiac function (reduces cardiac oxygen demand by slowing heart rate and increases oxygen delivery by causing coronary vasodilation) and its physiologic effects convey cardioprotection during myocardial ischemia.
Electroencephalogram
The effects of benzodiazepines on the EEG resemble those of barbiturates in that α activity is decreased and low-voltage rapid β activity is increased. This shift from α to β activity occurs more in the frontal and rolandic areas with benzodiazepines, which, unlike the barbiturates, do not cause posterior spread. In common with barbiturates, however, tolerance to the effects of benzodiazepines on the EEG does not occur. Midazolam, in contrast to barbiturates and propofol, is unable to produce an isoelectric EEG.
Side Effects
Fatigue and drowsiness are the most common side effects in patients treated chronically with benzodiazepines. Sedation that could impair performance usually subsides within 2 weeks in patients chronically treated with benzodiazepines. Patients should be instructed to ingest benzodiazepines before meals and in the absence of antacids because meals and antacids may decrease absorption from the gastrointestinal tract. Chronic administration of benzodiazepines does not adversely affect systemic blood pressure, heart rate, or cardiac rhythm. Although effects on ventilation seem to be absent, it may be prudent to avoid these drugs in patients with chronic lung disease characterized by hypoventilation and/or decreased arterial oxygenation as they may interact with other medications to have adverse effects. Decreased motor coordination and impairment of cognitive function may occur, especially when benzodiazepines are used in combination with other CNS depressant drugs. Acute administration of benzodiazepines may produce transient anterograde amnesia, especially if there is concomitant ingestion of alcohol. For example, there have been reports of profound amnesia in travelers who have ingested triazolam combined with alcohol to facilitate sleep on airline flights across several time zones.135
Drug Interactions
Benzodiazepines exert synergistic sedative effects with other CNS depressants including alcohol, inhaled and injected anesthetics, opioids, and α2 agonists. Anesthetic requirements for inhaled and injected anesthetics are decreased by benzodiazepines. Although benzodiazepines, especially midazolam, potentiate the ventilatory depressant effects of opioids, the analgesic actions of opioids are reduced by benzodiazepines.136,137 Indeed, antagonism of benzodiazepine effects with flumazenil results in enhanced analgesic effects of opioids.
Hypothalamic-Pituitary-Adrenal Axis
Benzodiazepine-induced suppression of the hypothalamic-pituitary-adrenal axis is supported by evidence of suppression of cortisol levels in treated patients.138 In animals, alprazolam produces dose-dependent inhibition of adrenocorticotrophic hormone and cortisol secretion.139 This suppression is enhanced compared with other benzodiazepines and may contribute to the unique efficacy of alprazolam in the treatment of major depression.
Dependence
Even therapeutic doses of benzodiazepines may produce dependence as evidenced by the onset of physical or psychologic symptoms after the dosage is decreased or the drug is discontinued. Symptoms of dependence may occur after more than 6 months’ use of commonly prescribed low-potency benzodiazepines. It is misleading to consider dependence as evidence of addiction in the absence of inappropriate drug-seeking behaviors. Withdrawal symptoms (irritability, insomnia, tremulousness) have a time of onset that reflects the elimination half-time of the drug being discontinued. Typically, symptoms of withdrawal appear within 1 to 2 days for short-acting benzodiazepines and within 2 to 5 days for longer acting drugs.
Aging
Aging and liver disease affect glucuronidation less than oxidative metabolic pathways. Lorazepam, oxazepam, and temazepam are metabolized only by glucuronidation and have no active metabolites. For this reason, these benzodiazepines may be preferentially selected in elderly patients over benzodiazepines, such as diazepam, and that is metabolized by hepatic microsomal enzymes to form active metabolites. Elderly patients may also be intrinsically sensitive to benzodiazepines, suggesting that the enhanced response to these drugs that occurs with aging has pharmacodynamic as well as pharmacokinetic components. Long-term benzodiazepine administration may accelerate cognitive decline in elderly patients. Benzodiazepine withdrawal symptoms in the elderly include confusion. Postoperative confusion is more common in elderly long-term benzodiazepine users (daily use for >1 year) than in short-term users or nonusers of benzodiazepines.140
Platelet Aggregation
Benzodiazepines may inhibit platelet-activating factor–induced aggregation resulting in drug-induced inhibition of platelet aggregation. Midazolam-induced inhibition of platelet aggregation may reflect conformational changes in platelet membranes.141 Although benzodiazepines significantly inhibit platelet aggregation in vitro, they do not appear to affect the risk of hemorrhagic complications in patients with severe, chemotherapy-induced thrombocytopenia142; the clinical significance of benzodiazepine-induced inhibition of platelet aggregation in the surgical arena is unclear.
Midazolam
Midazolam is a water-soluble benzodiazepine with an imidazole ring in its structure that accounts for stability in aqueous solutions and rapid metabolism.143 This benzodiazepine has replaced diazepam for use in preoperative medication and conscious sedation. As with other benzodiazepines, the amnestic effects of midazolam are more potent than its sedative effects. Thus, patients may be awake following administration of midazolam but remain amnestic for events and conversations (postoperative instructions) for several hours.
Commercial Preparation
The pK of midazolam is 6.15, which permits the preparation of salts that are water soluble. The parenteral solution of midazolam used clinically is buffered to an acidic pH of 3.5. This is important because midazolam is characterized by a pH-dependent ring-opening phenomenon in which the ring remains open at pH values of less than 4, thus maintaining water solubility of the drug (Fig. 5-11). The ring closes at pH values of greater than 4, as when the drug is exposed to physiologic pH, thus converting midazolam to a highly lipid-soluble drug (see Fig. 5-11). The water solubility of midazolam obviates the need for a solubilizing preparation, such as propylene glycol required for other benzodiazepines that can produce venoirritation or interfere with absorption after intramuscular (IM) injection. Indeed, midazolam causes minimal to no discomfort during or after IV or IM injection. Midazolam is compatible with lactated Ringer solution and can be mixed with the acidic salts of other drugs, including opioids and anticholinergics.

Pharmacokinetics
Midazolam undergoes rapid absorption from the gastrointestinal tract and prompt passage across the blood–brain barrier. Despite this prompt passage into the brain, midazolam is considered to have a slow effect-site equilibration time (0.9 to 5.6 minutes) compared with other drugs such as propofol and thiopental. In this regard, IV doses of midazolam should be sufficiently spaced to permit the peak clinical effect to be appreciated before a repeat dose is considered. Only about 50% of an orally administered dose of midazolam reaches the systemic circulation, reflecting a substantial first-pass hepatic effect. As for most benzodiazepines, midazolam is extensively bound to plasma proteins; this binding is independent of the plasma concentration of midazolam (Table 5-2).143,144 The short duration of action of a single dose of midazolam is due to its lipid solubility, leading to rapid redistribution from the brain to inactive tissue sites as well as rapid hepatic clearance. The context-sensitive half-times for diazepam and lorazepam are prolonged compared with midazolam.

The elimination half-time of midazolam is 1 to 4 hours, which is much shorter than that of diazepam (see Table 5-2).143 The elimination half-time may be doubled in elderly patients, reflecting age-related decreases in hepatic blood flow and possibly enzyme activity. The volume of distribution (Vd) of midazolam and diazepam are similar, probably reflecting their similar lipid solubility and high degree of protein binding. Elderly and morbidly obese patients have an increased Vd of midazolam resulting from enhanced distribution of the drug into peripheral adipose tissues. The clearance of midazolam is more rapid than that of diazepam, as reflected by the context-sensitive half-time. As a result of these differences, the CNS effects of midazolam would be expected to be shorter than those of diazepam. Indeed, tests of mental function return to normal within 4 hours after the administration of midazolam in healthy young patients.
The institution of cardiopulmonary bypass is associated with a decrease in the plasma concentration of midazolam and an increase on termination of cardiopulmonary bypass.22 These changes are attributed to redistribution of priming fluid into body tissues. In addition, benzodiazepines are extensively bound to protein, and changes in protein concentrations and pH that accompany institution and termination of cardiopulmonary bypass may have significant effects on the unbound and pharmacologically active fractions of these drugs. The elimination half-time of midazolam is prolonged after cardiopulmonary bypass.
Metabolism
Midazolam is rapidly metabolized by hepatic and small intestine cytochrome P450 (CYP3A4) enzymes to active and inactive metabolites (Fig. 5-12).143 The principal metabolite of midazolam, 1-hydroxymidazolam, has approximately half the activity of the parent compound.145 This active metabolite is rapidly conjugated to 1-hydroxymidazolam glucuronide and is subsequently cleared by the kidneys. This glucuronide metabolite has substantial pharmacologic activity when present in high concentrations, as may occur in critically ill patients with renal insufficiency who are receiving continuous IV infusions of midazolam over prolonged periods of time. In these patients, the glucuronide metabolite may have synergistic sedative effects with the parent compound.146 The other pharmacologically active metabolite of midazolam, 4-hydroxymidazolam, is not present in detectable concentrations in the plasma following IV administration of midazolam.

Metabolism of midazolam is slowed in the presence of drugs (cimetidine, erythromycin, calcium channel blockers, antifungal drugs) that inhibit cytochrome P450 enzymes resulting in unexpected CNS depression.147 Cytochrome P450 3A enzymes also influence the metabolism of fentanyl. In this regard, the hepatic clearance of midazolam is inhibited by fentanyl as administered during general anesthesia.148 Overall, the hepatic clearance rate of midazolam is 5 times greater than that of lorazepam and 10 times greater than that of diazepam.
Renal Clearance
The elimination half-time, Vd, and clearance of midazolam are not altered by renal failure.149 This is consistent with the extensive hepatic metabolism of midazolam.
Effects on Organ Systems
Central Nervous System
Midazolam, like other benzodiazepines, produces decreases in CMRO2 and cerebral blood flow analogous to barbiturates and propofol. Midazolam causes dose-related changes in regional cerebral blood flow in brain regions associated with the normal functioning of arousal, attention, and memory.150 Cerebral vasomotor responsiveness to carbon dioxide is preserved during midazolam anesthesia.43 Patients with decreased intracranial compliance show little or no change in ICP when given midazolam doses of 0.15 to 0.27 mg/kg IV. Thus, midazolam is an acceptable alternative to barbiturates for induction of anesthesia in patients with intracranial pathology. There is some evidence, however, that patients with severe head trauma but ICP of less than 18 mm Hg may experience an undesirable increase in ICP when midazolam (0.15 mg/kg IV) is administered rapidly (Fig. 5-13).151 Similar to thiopental, induction of anesthesia with midazolam does not prevent increases in ICP associated with direct laryngoscopy for tracheal intubation.152 Although midazolam may improve neurologic outcome after incomplete ischemia, benzodiazepines have not been shown to possess neuroprotective activity in humans. Midazolam is a potent anticonvulsant effective in the treatment of status epilepticus. Prolonged sedation of infants in critical care units (4 to 11 days) with midazolam and fentanyl has been associated with encephalopathy on withdrawal of the benzodiazepine.153 Paradoxical excitement occurs in less than 1% of all patients receiving midazolam and is effectively treated with a specific benzodiazepine antagonist, flumazenil.154

Ventilation
Midazolam produces dose-dependent decreases in ventilation with 0.15 mg/kg IV producing effects similar to diazepam, 0.3 mg/kg IV.155 Patients with chronic obstructive pulmonary disease experience even greater midazolam-induced depression of ventilation.156 Transient apnea may occur after rapid injection of large doses of midazolam (>0.15 mg/kg IV), especially in the presence of preoperative medication that includes an opioid.157 In healthy volunteers, midazolam alone produced no ventilatory depressant effects, whereas the combination of midazolam, 0.05 mg/kg IV, and fentanyl, 2 µg/kg IV, resulted in arterial hypoxemia and/or hypoventilation.158 Midazolam, 0.05 or 0.075 mg/kg IV, was shown to depress resting ventilation in healthy volunteers, whereas spinal anesthesia (mean sensory level T6) stimulated resting ventilation, and the combination had a modest synergistic effect for depressing resting ventilation.159 Benzodiazepines also depress the swallowing reflex and decrease upper airway activity.
Cardiovascular System
Midazolam, 0.2 mg/kg IV, for induction of anesthesia produces a greater decrease in systemic blood pressure and increase in heart rate than does diazepam, 0.5 mg/kg IV.160 Conversely, these midazolam-induced hemodynamic changes are similar to the changes produced by thiopental, 3 to 4 mg/kg IV.161 Cardiac output is not altered by midazolam, suggesting that blood pressure changes are due to decreases in systemic vascular resistance. In this regard, benzodiazepines may be beneficial in improving cardiac output in the presence of congestive heart failure. In the presence of hypovolemia, administration of midazolam results in enhanced blood pressure–lowering effects similar to those produced by other IV induction drugs.162 Midazolam does not prevent blood pressure and heart rate responses evoked by intubation of the trachea. In fact, this mechanical stimulus may offset the blood pressure–lowering effects of large doses of midazolam administered IV. The effects of midazolam on systemic blood pressure are directly related to the plasma concentration of the benzodiazepine. However, a plateau plasma concentration appears to exist (ceiling effect) above which little further change in systemic blood pressure occurs.
Clinical Uses
Preoperative Medication
Midazolam is the most commonly used oral preoperative medication for children. Oral midazolam syrup (2 mg/mL) is effective for producing sedation and anxiolysis at a dose of 0.25 mg/kg with minimal effects on ventilation and oxygen saturation even when administered at doses as large as 1 mg/kg (maximum, 20 mg).163 Midazolam, 0.5 mg/kg administered orally 30 minutes before induction of anesthesia, provides reliable sedation and anxiolysis in children without producing delayed awakening (Fig. 5-14).164 Although it is recommended that oral midazolam be administered at least 20 minutes before surgery, there is evidence that significant anterograde amnesia is present when 0.5 mg/kg orally is administered 10 minutes before surgery.165 Midazolam crosses the placenta but the fetal to maternal ratio is significantly less than that for other benzodiazepines.

Intravenous Sedation
Midazolam in doses of 1.0 to 2.5 mg IV (onset within 30 to 60 seconds, time to peak effect 3 to 5 minutes, duration of sedation 15 to 80 minutes) is effective for sedation during regional anesthesia as well as for brief therapeutic procedures. The effect-site equilibrium time for midazolam must be considered in recognizing the likely time of peak clinical effect and the need for supplemental doses of midazolam.
The most significant side effect of midazolam when used for sedation is depression of ventilation caused by a decrease in the hypoxic drive, particularly in concert with other anesthetic drugs. Midazolam-induced depression of ventilation is exaggerated (synergistic effects) in the presence of opioids and other CNS depressant drugs.137 Patients with chronic obstructive pulmonary disease may also manifest exaggerated depression of ventilation following administration of benzodiazepines to produce sedation. It is important to appreciate that increasing age greatly increases pharmacodynamic variability and is associated with generally increased sensitivity to the hypnotic effects of midazolam.166
Induction of Anesthesia
Although seldom used for this purpose currently, anesthesia can be induced by administration of midazolam, 0.1 to 0.2 mg/kg IV, over 30 to 60 seconds. Nevertheless, thiopental usually produces induction of anesthesia 50% to 100% faster than midazolam (Fig. 5-15).167 Onset of unconsciousness (synergistic interaction) is facilitated when a small dose of opioid (fentanyl, 50 to 100 µg IV or its equivalent) precedes the injection of midazolam by 1 to 3 minutes. The dose of midazolam required for the IV induction of anesthesia is also less when preoperative medication includes a CNS depressant drug. In healthy patients receiving small doses of benzodiazepines, the cardiovascular depression associated with these drugs is minimal. When significant cardiovascular responses occur, it is most likely a reflection of benzodiazepine-induced peripheral vasodilation. As with depression of ventilation, cardiovascular changes produced by benzodiazepines may be exaggerated in the presence of other CNS depressant drugs such as propofol and thiopental.

Maintenance of Anesthesia
Midazolam may be administered to supplement opioids, propofol, and/or inhaled anesthetics during maintenance of anesthesia. The context-sensitive half-time for midazolam increases modestly with an increasing duration of administration of a continuous infusion of this benzodiazepine.15 Anesthetic requirements for volatile anesthetics are decreased in a dose-dependent manner by midazolam. Awakening after general anesthesia that includes induction of anesthesia with midazolam is 1.0 to 2.5 times longer than that observed when thiopental is used for the IV induction of anesthesia.168 Gradual awakening in patients who receive midazolam is rarely associated with nausea, vomiting, or emergence excitement.
Postoperative Sedation
Long-term IV administration of midazolam (loading dose 0.5 to 4 mg IV and maintenance dose 1 to 7 mg per hour IV) to produce sedation in intubated patients results in relative saturation of peripheral tissues with midazolam and clearance from the systemic circulation becomes less dependent on redistribution into peripheral tissues and more dependent on hepatic metabolism.169 In addition, pharmacologically active metabolites may accumulate with prolonged IV administration of the parent drug. Under these conditions, plasma concentrations of midazolam decrease more slowly (emergence delayed) after discontinuation of the IV infusion compared with single IV injections. Emergence time is also a function of the plasma concentrations of midazolam at the time the IV infusion is discontinued. Patients maintained at higher plasma concentrations of midazolam take longer to awaken than patients maintained at lower plasma concentrations for comparable periods of time. The concomitant administration of analgesic doses of opioids greatly decreases the needed dose of midazolam and results in a more rapid recovery from sedation following discontinuation of the IV infusion of midazolam.169 Emergence time from midazolam infusion is increased in elderly patients, obese patients, and in the presence of severe liver disease.
Paradoxical Vocal Cord Motion
Paradoxical vocal cord motion is a cause of nonorganic upper airway obstruction and stridor that may manifest postoperatively. Midazolam 0.5 to 1 mg IV may be an effective treatment for paradoxical vocal cord motion.170
Diazepam
Diazepam is a highly lipid-soluble benzodiazepine with a more prolonged duration of action compared with midazolam. Because of the beneficial aspects of midazolam pharmacology, parenteral diazepam is seldom used as part of current anesthetic regimens.
Commercial Preparation
Diazepam is dissolved in organic solvents (propylene glycol, sodium benzoate) because it is insoluble in water. The solution is viscid, with a pH of 6.6 to 6.9. Dilution with water or saline causes cloudiness but does not alter the potency of the drug. Injection by either the IM or IV route may be painful. Diazepam is also available in a unique soybean formulation for IV injection. This formulation is associated with a lower incidence of pain on injection and thrombophlebitis.
Pharmacokinetics
Diazepam is rapidly absorbed from the gastrointestinal tract after oral administration, reaching peak concentrations in about 1 hour in adults but as quickly as 15 to 30 minutes in children. There is rapid uptake of diazepam into the brain, followed by redistribution to inactive tissue sites, especially fat, as this benzodiazepine is highly lipid soluble. The Vd of diazepam is large, reflecting extensive tissue uptake of this lipid-soluble drug (see Table 5-2). Women, with a greater body fat content, are likely to have a larger Vd for diazepam than men. Diazepam rapidly crosses the placenta, achieving fetal concentrations equal to and sometimes greater than those present in the maternal circulation.171 The duration of action of benzodiazepines is not linked to receptor events but rather is determined by the rate of metabolism and elimination.
Protein Binding
The protein binding of benzodiazepines parallels their lipid solubility. As such, highly lipid-soluble diazepam is extensively bound, presumably to albumin (see Table 5-2). Cirrhosis of the liver or renal insufficiency, with associated decreases in plasma concentrations of albumin, may manifest as decreased protein binding of diazepam and an increased incidence of drug-related side effects.172 The high degree of protein binding limits the efficacy of hemodialysis in the treatment of diazepam overdose.
Metabolism
Diazepam is principally metabolized by hepatic microsomal enzymes using an oxidative pathway of N-demethylation. The two principal metabolites of diazepam are desmethyldiazepam and oxazepam, with a lesser amount metabolized to temazepam (Fig. 5-16). Desmethyldiazepam is metabolized more slowly than oxazepam and is only slightly less potent than diazepam. Therefore, it is likely that this metabolite contributes to the return of drowsiness that manifests 6 to 8 hours after administration of diazepam, as well as to sustained effects usually attributed to the parent drug. Alternatively, enterohepatic recirculation may contribute to recurrence of sedation.173 The plasma concentration of diazepam at this time is clinically insignificant and probably reflects its rapid removal as a conjugate of glucuronic acid. Ultimately, desmethyldiazepam is excreted in urine in the form of oxidized and glucuronide conjugated metabolites. Unchanged, diazepam is not appreciably excreted in urine. Benzodiazepines do not produce enzyme induction.

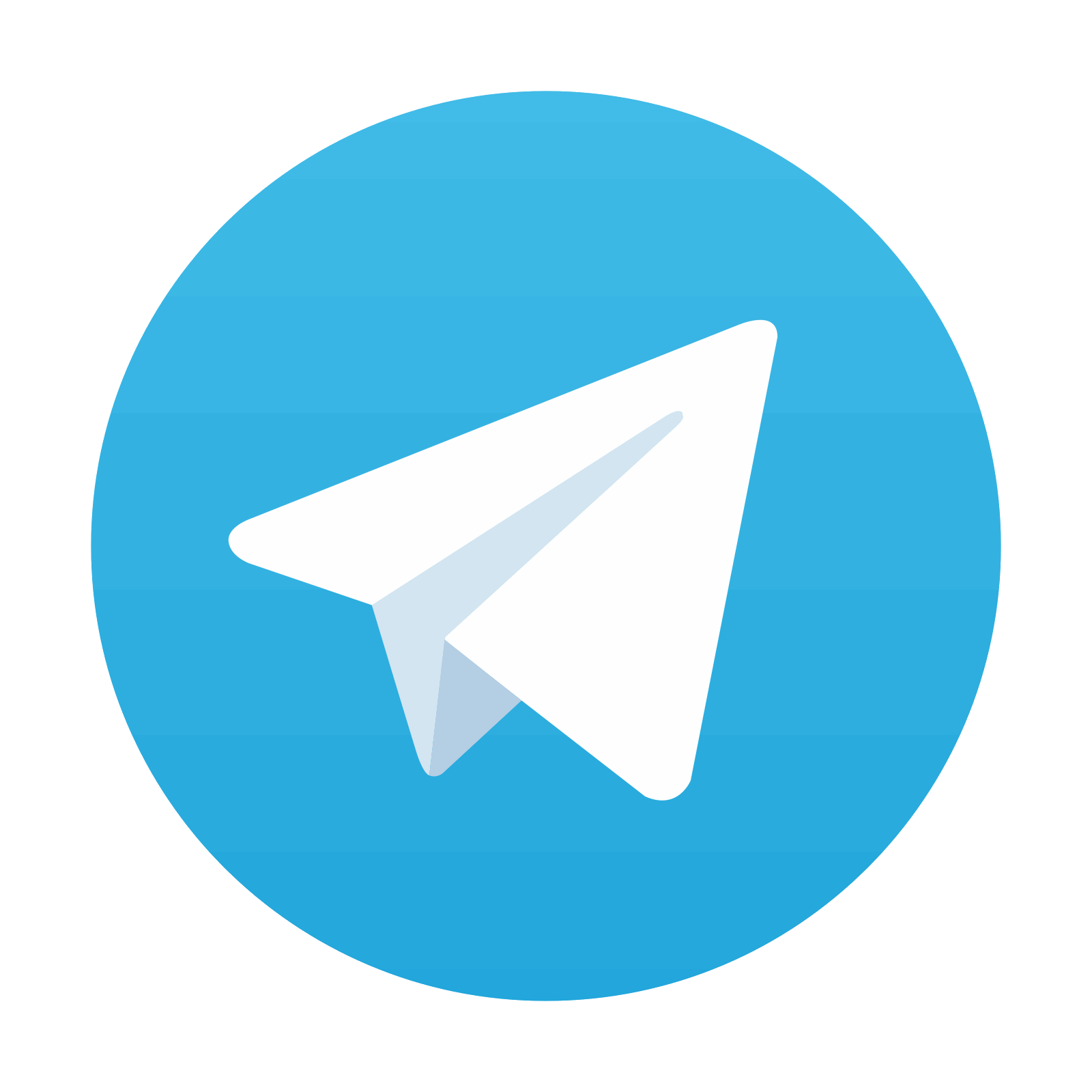
Stay updated, free articles. Join our Telegram channel

Full access? Get Clinical Tree
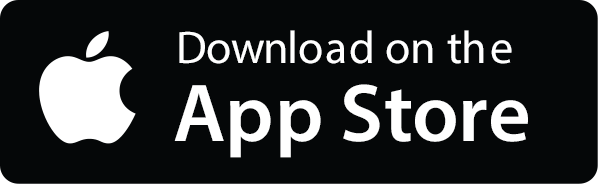
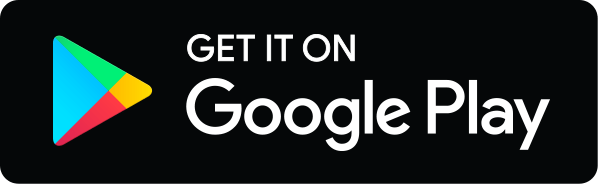