Fig. 22.1
Angiogram of an arteriovenous malformation (AVM)
Treatment options for AVMs include expectant monitoring versus complete obliteration using microneurosurgery, endovascular techniques, or radiosurgery. Embolization can be employed presurgically or preradiosurgically for progressive flow reduction and decrease in nidus size, to occlude deep/inaccessible arterial vessels and to eliminate related aneurysms. It can also be used palliatively for worsening neurological deficits in large AVMs not suitable for surgical management. Radiosurgery is usually employed to destroy small AVMs, especially if they are poorly accessible or if they are in an eloquent brain location [3]. In patients who undergo radiosurgery that then fails, surgery is usually facilitated, since deep and fragile arteriolar feeders tend to become fibrotic and easier to manage [7]. Surgically, AVMs are usually excised with routine microsurgical technique by ligating arterial feeders and then excising the nidus and resecting the draining vein(s) [1, 3] (Fig. 22.2).
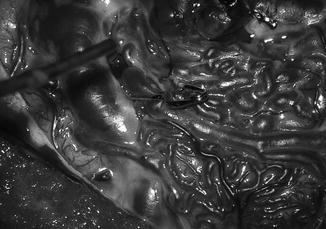
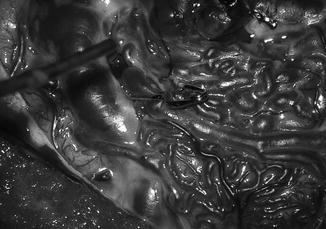
Fig. 22.2
Microsurgical excision of an arteriovenous malformation with a temporary clip in place
Prior to management, it is sometimes necessary to perform Wada testing with intracarotid injection or superselective Wada testing in the neurointerventional suite with specific arterial injection of usually the short-acting barbiturate amobarbital to inhibit gray matter structures and the local anesthetic lidocaine to inhibit white matter structures to detect eloquent brain tissue perfused en passage by AVM feeding arteries to better preserve function [8]. For embolization , materials used include Gelfoam (from purified skin gelatin), polyvinyl alcohol particles, N-butyl cyanoacrylate, Onyx (a non-adhesive liquid polymer), and a variety of coils [9]. Although the patient is awake for Wada testing, general anesthesia is preferred at many centers for AVM embolization to facilitate imaging and prevent patient movement. Depending on the agent deployed, some specific anesthetic maneuvers may be undertaken. For example, transient profound-induced hypotension may be necessary to aid in correct embolic glue setting, and temporary apnea is sometimes requested to improve visualization [10] (see Chaps. 20 and 21 for discussion of potential physiological effects on neuromonitoring as well as later in this chapter).
Routine neuroanesthetic management for intracranial lesions applies to AVM surgical resections. Because AVM resections are generally elective, the patient should be medically optimized prior to surgery. There is the potential for rapid and massive blood loss, so adequate intravascular access and blood availability is mandatory [3, 11]. Risk of AVM rupture during induction is low, but it should be remembered that the incidence of a coexisting intracranial aneurysm is at least about 10 %, so blood pressure control in the range of the patient’s normal pressures is warranted [1, 3, 11]. The selected anesthetic regimen must maintain hemodynamic stability, provide amnesia, prevent patient movement to noxious stimulation, facilitate neuromonitoring, and allow rapid emergence, but no specific regimens have demonstrated superior safety in intracranial surgery [12, 13]. Anesthetics that decrease cerebral metabolic rate and vasoactive agents that do not promote cerebral vasodilation are usually selected. Maintenance of normothermia or a mild decrease in body temperature resulting from general anesthesia is generally accepted. Patients should be rewarmed in preparation for emergence [3, 11, 14].
Case Presentation
A 35-year-old woman with no significant past medical history presents for craniotomy for AVM resection after the AVM was incidentally discovered on an MRI performed for the patient’s complaint of headaches. The AVM is located in the middle cerebral artery territory, and it has a maximal diameter of 4 cm.
What management could be considered to potentially decrease blood loss prior to surgical resection of the AVM?
Staged reduction in blood supply to the malformation via intra-arterial embolization is often recommended to decrease the size of the arteriovenous shunt [3, 6]. (For information on neuromonitoring for interventional neuroradiology procedures, see Chap. 42, “Interventional Neuroradiology”.) To avoid development of collateral blood flow, surgical resection of the AVM should occur within several days after embolization of the final feeding artery [3].
Which monitors should you consider for the surgical resection ?
Monitoring for cerebrovascular surgery is aimed at all the physiological influences on cerebral blood flow [15]. American Society of Anesthesiologists’ standard monitors including end-tidal carbon dioxide and temperature monitoring should be used. Because manipulation of systemic pressure with vasoactive agents is often required, intra-arterial pressure monitoring also is indicated. Central venous pressure monitoring may be desired for assistance in determining volume status. Osmotic diuretics are often used, mandating urine output monitoring. If the operative site is above heart level, a precordial Doppler probe to monitor for air entrainment should be employed (and placement of a multiorifice catheter for air aspiration should also be considered). Finally, especially if deliberate temporary arterial occlusion is planned, multimodal neurophysiological monitoring—electroencephalography (EEG), somatosensory evoked potential (SSEP) , and motor-evoked potential (MEP) monitoring—should be considered to help identify cerebral ischemia (and therefore identify feeder vessels to eloquent areas) [16]. (See Chaps. 10 [“EEG Monitoring”], 1 [“Somatosensory Evoked Potentials], and 2 [“Transcranial Motor Evoked Potentials”] for the technical aspects of performing these neuromonitoring techniques, respectively.)
What are potential benefits of combining SSEP and MEP monitoring?
There is a dearth of prospective and randomized trials of neurophysiological monitoring in intracranial surgery. However, there is evidence, from both adult and pediatric patient series, that neuromonitoring intraoperatively is useful to help prevent morbidity in surgical and endovascular management of cerebral AVMs [17–20]. Because SSEPs and MEPs provide complementary information about the neurological system, combining these modalities may give a better reflection of the patient’s actual neurological status [21]. In fact, in up to 25 % of cases in which a new postoperative deficit manifests in intracranial neurovascular surgery (especially intracranial aneurysm surgery), intraoperative SSEP recordings were unchanged. This is not surprising since anatomically distinct arteries not supplying sensory pathways supply parts of the motor pathway (e.g., subcortical portions) [22]. MEPs also can give earlier warning of potential ischemic injury compared to SSEPs [23]. Finally, if there is difficulty in obtaining signals from one of the modalities, combining SSEPs and MEPs can increase the number of patients who can be successfully monitored [21]. Of course, it is especially important to ensure the anatomic area most at risk from the particular surgery is monitored, as discussed in Chap. 21 (“Intracranial Aneurysm Clipping”).
Although the majority of AVMS are suptratentorial in location, what additional neuromonitoring would be helpful if the AVM involved the posterior fossa/vertebrobasilar circulation ?
Posterior circulation and brainstem ischemia can be identified by using a combination of SSEPs, MEPs, and auditory brainstem-evoked potentials (ABRs) [17, 24] (see Chap. 3, “Auditory Evoked Potentials” for additional information). Electromyography for cranial nerve monitoring of any cranial nerve with a motor component at anatomic risk may also be added to the neuromonitoring regimen (see Chap. 7, “Electromyography”, for additional information).
When should neuromonitoring be performed during the case?
Recordings should be obtained after anesthesia induction and once a steady state of anesthesia is achieved (but before incision) as a baseline. Obtaining a baseline before incision allows time for troubleshooting if technical difficulties are encountered, and, if evoked potential changes occur, it could help identify (and allow correction of) patient malpositioning while a surgical cause is a nonfactor. Recordings should be obtained at regular intervals during the procedure, even when there is minimal risk of neural injury. Anesthetic agents and levels, physiological parameters (mean arterial pressure and temperature), and surgical events should be noted with each reading. If burst suppression is initiated, a new “baseline” should be obtained before critical surgical maneuvers are undertaken, since high doses of most intravenous anesthetics can affect evoked potentials [25]. During critical surgical manipulation, neuromonitoring should be performed continually.
Transcranial MEP stimulation can cause movement of the patient. What can be done to minimize this movement?
Naturally occurring field movement can be limited with transcranial MEP stimulation by minimizing stimulus intensity to the lowest amount that results in reliable MEPs [26]. To avoid movement of the microsurgical field during critical surgical work, brief surgical pauses for monitoring should be coordinated with MEP testing. Often, MEP stimulation can be facilitated by the anesthesiologist noting a pause in surgery and initiating communication between the technologist and surgeon to efficiently acquire MEPs. Most MEP acquisitions can be timed to coincide with surgical instrument exchange. In addition, some stimulation locations used for MEPs may be associated with less movement. (To not sacrifice SSEP and/or MEP head electrode position when craniotomy site interferes with ideal electrode positioning, sterile electrodes can be placed by the surgeon.) Video monitoring can also be used to assist the neurophysiology team in timing stimulation with surgical pauses.
Spontaneous movement of the patient under anesthesia without muscle relaxation, which could occur with MEP stimulation, can be largely avoided by keeping the patient adequately anesthetized. We routinely use a maintenance anesthetic technique of moderate opioid dosing (≥0.1 μg kg−1 min−1 remifentanil, 0.5 minimum alveolar concentration (MAC) of volatile anesthetic, and 0–150 μg kg−1 min−1 propofol). We pay close attention to volume status and phenylephrine (10–50 μg min−1) is added, if needed, to maintain adequate hemodynamics. Since much of the anesthetic regimen is via intravenous infusion, diligence is required to ensure that drug delivery problems, such as extravasation or mechanical obstruction, do not occur (which could result in the patient being “light” and spontaneously moving).
After initial baseline MEP recordings are obtained, you note detectable signals from bilateral upper and lower extremities. However, you notice that both contralateral and ipsilateral waveforms are obtained with stimulation of one hemisphere. What is happening and what can be done to correct this?
The stimulation intensity should be decreased. Otherwise, during surgery, cerebral ischemia could be missed, resulting in false-negative findings . This is because too high of a transcranial stimulus intensity can activate deep subcortical motor pathways and bypass ischemic higher cortical levels, resulting in myogenic MEPs from the contralateral limbs being generated despite cerebral ischemia [17, 27]. Deep subcortical motor pathway ischemia could also be missed if activation of the corticospinal tract occurred even more caudally [26, 28]. In other words, as the intensity of stimulation is increased, the activation site of corticospinal fibers is shifted deeper into the brain. There appears to be a limit to how deeply the site of activation can be displaced by increasing the transcranial MEP stimulation intensity, and this limit appears to be similar to the site activated by stimulation at the level of the brainstem (likely at the pyramidal decussation) [29]. Higher stimulation intensity is required to overcome muscle relaxation, so avoiding muscle relaxant post-intubation can help minimize stimulus intensity [30, 31]. In summary, transcranial MEPs are made more accurate by decreasing the stimulation intensity [27].
The stimulation intensity is decreased to the point where only contralateral extremity waveforms are obtained. However, now there is no signal from one foot. Surgery has not yet begun. What can be done to improve the signal knowing that increasing the intensity results in bilateral limb stimulation?
The monitoring team can increase the number of stimulus pulses in the stimulation train. Often, lower extremity MEP responses require more train pulses than upper extremity MEPs [28]. Since the threshold intensity of MEPs depends on the number of pulses, the duration of each pulse, and the locations of the stimulating and recording electrodes, the amplitude of muscle responses generally can be increased by increasing the number of pulses, the duration of each pulse, and/or the stimulation intensity and by optimizing electrode locations [32]. (However, as noted above, increasing the stimulation intensity can have the undesirable effect of incorrect localization.) In this patient, with the increase in stimulus train count, detectable signals in all four extremities return with bilateral stimulation.
What nonradiographic intraoperative method could be used to help delineate the vasculature while the surgeons are dissecting around the AVM?
Although digital subtraction angiography is the “gold standard” for evaluating vascular flow in AVM surgery, distinguishing AVM vasculature also can be facilitated by several nonradiographic methods, including Doppler ultrasound imaging and fluorescent angiography [33, 34]. Indocyanine green (ICG) video angiography is one of the fluorescent techniques, and it is based on the different timing of vessel fluorescence. After intravenous injection, ICG leads to arteries fluorescing first (within 3–12 s), then arterialized veins, and then nonarterialized cortical veins. Images are viewed using a near-infrared video integrated microscope. The light source includes the ICG excitation wavelength, and the images are viewed through an optical filter that allows only fluorescence in the ICG emission wavelength. The recommended dose of ICG for angiography is 0.2–0.5 mg kg−1. ICG is cleared by the liver, and it has a mean half-life of 3–4 min. Of note, viewing ICG fluorescence is hindered by blood, so, if a hematoma is present, it should be removed as much as possible before ICG injection [34]. ICG video angiography can be used not only to distinguish AVM vasculature, but also serial imaging during dissection can be useful in identifying feeder vessels and in assessing nidus perfusion, as well as confirming complete removal after lesion resection [35]. At our institution, we routinely use a lower dose of ICG (approximately 0.1–0.2 mg kg−1) with good success, allowing for faster “washout” to facilitate repeat imaging quickly if needed. See a video of intraoperative use of ICG in aneurysm surgery in Chap. 21, “Intracranial Aneurysm Clipping.”
The electrophysiologist reports to the surgery and anesthesia teams that the amplitude of the MEP response from one lower extremity has decreased significantly. The surgeons state that they are not working in the territory of the anterior cerebral artery, so they do not expect to see changes in the MEPs from the lower extremities. The surgeons also recently had noted that the brain was “tight”, so the patient currently is being more aggressively hyperventilated. What should you do to determine the etiology of the MEP change and possibly mitigate any neurological problem?
Since the change is unilateral, a technical, positional, or surgical etiology is suspected (instead of a physiologic or pharmacologic etiology which should cause a bilateral change). The electrophysiologist should repeat the MEP stimulus to ensure the problem is reproducible. Once reproducibility is confirmed, the electrophysiologist should check for possible technical problems by checking electrode impedances and stimulation parameters. As this technical check is occurring, the anesthesia team should check the patient’s position and padding while paying special attention to the affected extremity and any monitors or intravenous lines in that extremity. In this case, if no technical or positional problems are detected, a surgical problem could remain; especially since the patient is being hyperventilated because of increased brain bulk, some local cortical injury from retractor pressure could be occurring [36]. Brain retraction injury can be caused by focal pressure from the retractor blade on brain tissue. The deformation of brain tissue from the retractor causes a reduction in blood flow to the area, and this can lead to ischemia. Local direct tissue trauma to the neurons, their processes, and/or glia is also possible. Resulting injury is likely dependent on the number and shape of retractors and on the pressure and duration of retraction [37]. Upon questioning, the surgeons state they do have a retractor that they can reposition, and they do so. To lessen potential ischemia to the area, raising the systemic blood pressure and less aggressive hyperventilation (since hyperventilation is decreasing cerebral blood flow) could be undertaken by the anesthesia team [36, 37]. Approximately 15 min after these maneuvers, the signals return to baseline. Of note, although prolonged hyperventilation has been shown to be detrimental in instances such as traumatic brain injury, intraoperative hyperventilation has been shown to improve operating conditions and moderate hyperventilation to facilitate surgery seems reasonable and is still routinely employed [13, 38].
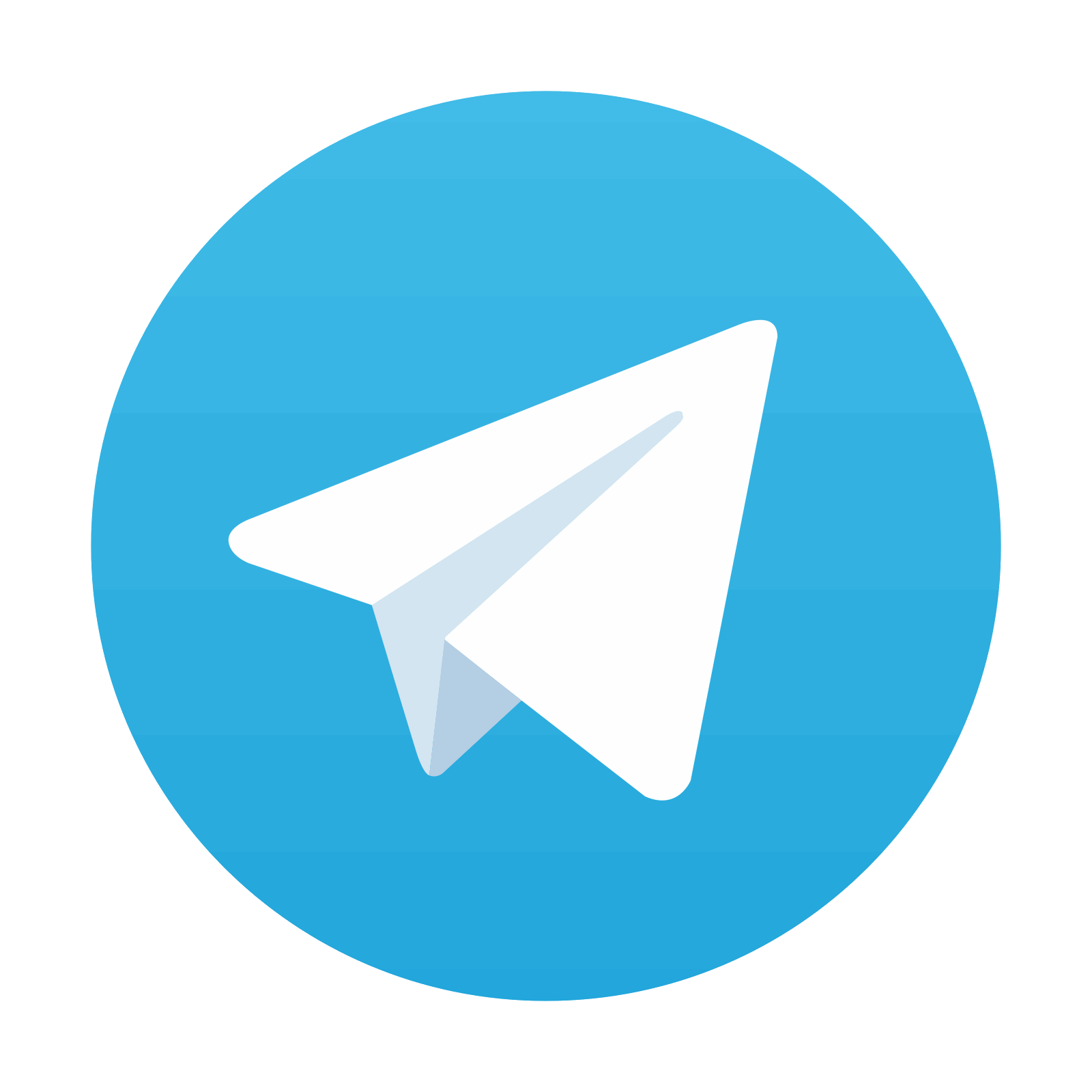
Stay updated, free articles. Join our Telegram channel

Full access? Get Clinical Tree
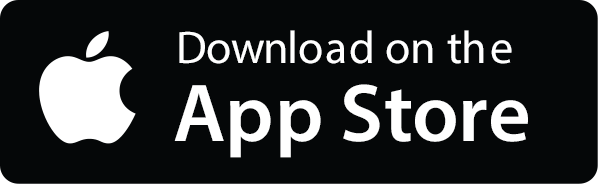
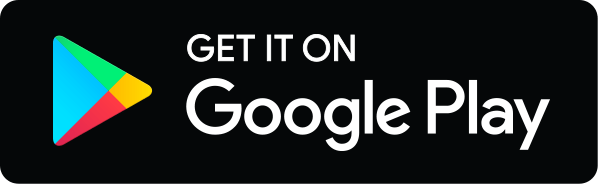