Innate Immunity and Inflammation
James A. Thomas
KEY POINTS




INTRODUCTION AND HISTORICAL BACKGROUND
To understand inflammation is, in some respects, to grasp the breadth of human disease. Necrotizing enterocolitis in premature neonates stressed by cold, infection, or formula feeding; bronchiolitis in infants with respiratory syncytial virus infections; and reperfusion injury in children after cardiac arrest or cardiopulmonary bypass are all characterized by intense local responses in affected tissues and sometimes activation of a highly catabolic systemic cascade that affects the entire host. Different injuries provoke distinct manifestations of the inflammatory response. A complex host can generate many more reactions (foreign body, granulomatous, suppurative, hypersensitivity, immune complex, and toxic or septic shock) than one with a simpler genome. While a comprehensive categorization of different inflammatory processes exceeds the scope of this chapter, the following sections outline some elements of an ancient system that has evolved to protect the host from insults to its integrity, innate immunity.
The survival of a species depends on its ability to perpetuate itself. To accomplish this, members must succeed at three fundamental activities. First, they must generate adequate nutrition to reach reproductive age. In the process of growing and maturing, they must also avoid becoming another organism’s food source. Finally, organisms must ensure passage of the species’ genetic information to the next generation.
The struggle to achieve these ends is fraught with danger. During the course of activities to survive and reproduce, organisms encounter agents that compromise their physical integrity and threaten their self-propagation. Gravity can hurl bodies from trees during food foraging, and bacteria can gain access to the warm, humid, and nutrient-rich subepithelium via abrasions or puncture wounds. Most major injuries or infections, such as those treated in modern ICUs, would be nonsurvivable without antibiotics, antiseptic surgery, and modern nursing care. To maintain a competitive advantage and survive minor to moderate threats, however, most species have evolved a repertoire of behavioral and physiologic responses to help them avoid or defend themselves against threats to their integrity. These countermeasures include mechanisms to limit secondary injury or microbial dissemination, if an initial attack succeeds and primary (epithelial) defenses are breached. After containing a threat, additional mechanisms modulate the intensity of the reaction and commence repairing the damage occasioned by the insult. Defects that impair any of these responses hamper the host’s survival chances.
The aggregate of early physiologic responses that detect and respond to injury is loosely referred to as innate immunity, while the inflammatory response represents innate immunity at work. This chapter summarizes the history of our evolving understanding of host defense and inflammation. It then defines major concepts associated with innate immunity and the inflammatory response. Subsequent sections review current thinking about the nature of injury signals, the acute cellular and systemic responses to injury, and their regulation and overall impact.
History of Knowledge of Inflammation and Innate Immunity
The concept of innate immunity is more modern than the notion of inflammation (1,2,3,4,5). Early Mesopotamian caregivers were familiar with the signs and symptoms of inflammation, but as an early codifier of medical knowledge, Hippocrates (460-377 bc) receives credit for both documenting the early vocabulary associated with the inflammatory response and recognizing it as a prerequisite for the healing process.
The Roman encyclopedist, Aulus Cornelius Celsus (˜35-50 bc) is the second ancient Westerner whose contribution to the study of inflammation survives. In his treatise, De Medicina, he coined the initial four cardinal signs of
inflammation: calor (heat), dolor (pain), tumor (swelling), and rubor (redness or erythema). The fifth sign functio laesa (dysfunction of inflamed organs) was attributed to the Greek physician Galen of Pergamon (129-200 ad), whose writings dominated Western medical thought until the European Renaissance. According to some scholars, Galen’s influence over the practice of medicine in Europe stifled observation and empiricism, and that medieval Muslim physicians in Spain, North Africa, and the Middle East contributed more to the understanding and treatment of inflammation than their European counterparts during this period.
inflammation: calor (heat), dolor (pain), tumor (swelling), and rubor (redness or erythema). The fifth sign functio laesa (dysfunction of inflamed organs) was attributed to the Greek physician Galen of Pergamon (129-200 ad), whose writings dominated Western medical thought until the European Renaissance. According to some scholars, Galen’s influence over the practice of medicine in Europe stifled observation and empiricism, and that medieval Muslim physicians in Spain, North Africa, and the Middle East contributed more to the understanding and treatment of inflammation than their European counterparts during this period.
Beutler identifies two threads in what we would now call the history of inflammation research. The first focuses on characterizing what is sensed or what triggers the inflammatory response. Before Koch promulgated his postulates, investigators attempted to purify toxins from putrid meat to better understand why the same process in a patient’s injured leg triggered gangrene, shock, and death. These studies ultimately gave way to the isolation and characterization of microbial toxins, such as lipopolysaccharide, peptidoglycan, and lipoteichoic acid.
The second line of inquiry concentrated on describing the response to infection and invasion. The Scottish surgeon, John Hunter (1728-1793) first recognized leukocytes at the site of inflammation and was one of the first to describe the inflammatory response as a form of host defense and not a disease process. Moreover, as light microscopy improved in the 18th and 19th centuries, investigators such as Dutrochet, Virchow, Waller, and Addison described inflammatory leukocyte morphology, loose association with the vessel wall, firm adherence to the vessel wall, and extravasation of leukocytes beyond the vessel wall. Cohnheim detailed the stepwise events of leukocyte rolling, adhesion, and diapedesis and speculated that changes in vascular wall properties promoted this process. Schultze’s descriptions of different leukocyte morphologies preceded Elie Metchnikov’s observations of phagocytosis in macrophages and granulocytes that led to the formulation of his cellular theory of immunity in 1884. Paul Erlich’s identification and analysis of antibodies and complement gave rise to the concept of humoral immunity and opened the era of functional studies that have characterized subsequent studies of immunity and inflammation.
Since the early 20th century, efforts to unravel the adaptive immune response eclipsed the study of innate immunity. Description of the first genetic defect underlying chronic granulomatous disease in the 1960s; isolation and characterization of cytokines, receptors, and signaling molecules critical to inflammation; recognition that adaptive immunity depends on innate immunity to function; and the information windfall from the Human Genome Project, have all helped fuel resurgent interest in understanding the depth and complexity of innate immunity.
Recognition of the relationship between inflammation and the neuroendocrine system has coalesced more recently than our comprehension of the roles of leukocytes, soluble mediators, and the vascular system. Investigation into the stress response has also highlighted the importance and interdependence of the central nervous system (CNS) and the hypothalamic-pituitary axis (HPA) in preparing complex hosts to defend themselves against different threats.
DEFINITIONS AND GENERAL CONCEPTS
Innate Immunity
As Beutler (6) observes, “it is sometimes difficult to decide
where the innate immune system ends and the rest of the host begins.” The statement reflects the difficulty inherent in trying to distinguish one physiologic system from another in an organism that functions as an integrated whole. Although the host’s innate response to injury or infection employs specialized cells and macromolecules to defend itself, any body tissue may become infected, traumatized, or deprived of blood flow. Tissues considered “nonimmune” must be able to detect and respond to an insult and have evolved different mechanisms (e.g., nearuniversal Toll-like receptor [TLR] expression, or interleukin-6 [IL-6] trans-signaling) to meet this challenge (7,8).

Defining the limits of the innate immune system may be futile, but characterizing core features and functions of innate immunity is possible. First, it is old, much older than adaptive
(lymphocyte-based) immunity. In fact, it most likely developed in parallel with early metazoans >600 million years ago (9). Moreover, elements of immunity shared by plants, invertebrates, and vertebrates alike, such as intracellular kinases (critical to disease resistance and pathogen recognition), are older than components found only in vertebrates. Second, like the nervous system with which it is tightly linked, innate immunity has both afferent and efferent limbs, suggesting the two systems may have evolved from a common ancestral system to sense threats and respond (6). Third, the cellular receptors for sensing invasion or injury are encoded in the germ line of the host, signifying that (a) they have evolved to recognize something essential and therefore invariant in either microbes or host, and (b) they can be mobilized quickly.

Three essential functions of innate immunity deserve mention, but only two will be discussed further. Pathogen or injury recognition is the first and most important of these. Without this ability, the host is immunologically blind to the threats surrounding it. The second function of innate immunity is to respond to the threat. Doing so effectively requires mechanisms to assess the magnitude, localization, and type of insult, and to match, coordinate, and regulate the response to that threat. Finally, and as an integral component of its response to microbial invasion, innate immunity instructs and focuses the adaptive immune response to the identity of the invader, and provides additional information about the preferred adaptive effector response (B cell- vs. T cell-mediated) (10). The adaptive immune response is discussed in subsequent chapters.
What, then, is innate immunity? The term refers to the cells, subcellular elements, and molecules that act to eliminate invading pathogens and limit the spread of tissue damage. The cells involved include leukocytes, particularly polymorphonuclear (PMN) cells (neutrophils, basophils, and eosinophils), mast cells, and mononuclear phagocytes (monocytes, tissue macrophages, and dendritic cells), but also lymphocytes, natural killer (NK) cells, neurons, and neuroendocrine cells. Platelets are the major subcellular elements and are essential to coagulation. Molecular mediators of innate immunity range from major antimicrobial proteins (complement, defensins, cathelicidins, lysozyme, and lactoferrin) to pro- and anti-inflammatory cytokines and chemokines (tumor necrosis factor TNF-α, IFN-α, IFN-β, IL-1β, IL-8, IL-6, IFN-γ, and IL-10) and to neurotransmitters involved in neurogenic inflammation (substance P, calcitonin gene-related peptide, epinephrine, and serotonin). The remainder of the chapter outlines how some of these components cooperate to protect the host following injury.
Adaptive Immunity
In contrast to the notion of a preset responsiveness inherent in innate immunity, adaptive immunity denotes the ability to vary an immune response depending on the received stimulus. The existence of this adaptability implies two additional qualities: the capacity to distinguish among different stimuli and a preexisting pool of effectors capable of responding to almost
any molecular motif. The nearly unlimited repertoire of lymphocytes, generated by somatic recombination and hypermutation of the genes that code for their antigen receptors, arises from the interaction between innate immune cells (antigenpresenting cells) and lymphocytes (T helper cells). Although adaptive immunity is a more recent and restricted system of host defense than innate immunity, it remains dependent on its evolutionary predecessor for proper function (10).
any molecular motif. The nearly unlimited repertoire of lymphocytes, generated by somatic recombination and hypermutation of the genes that code for their antigen receptors, arises from the interaction between innate immune cells (antigenpresenting cells) and lymphocytes (T helper cells). Although adaptive immunity is a more recent and restricted system of host defense than innate immunity, it remains dependent on its evolutionary predecessor for proper function (10).
Inflammation
Defining inflammation is complex because it comes in many guises. A peritonsillar abscess and necrotizing bacterial pneumonia, lupus nephritis and acute tubular necrosis leading to renal failure, and the cerebral responses to trauma and viral
encephalitis are all inflammatory diseases. These reactions share elements in common, but also have unique aspects to them that are determined by the injury itself, the tissue(s) involved, and the overall state of the host at the time of insult. The inflammatory response, broadly defined, is:

A localized reaction, that can be elicited by microbial invasion or sterile tissue injury, which serves to destroy, dilute, or sequester both the injurious agent and injured tissue and to initiate the healing process.
An intact inflammatory response is essential for host survival, performing at least three critical functions. First it prevents disease extension. Through a variety of strategies, including extravasation of serum proteins, migration of leukocyte effectors, and local thrombosis, innate immunity contains invading microbes and delineates boundaries between viable and nonviable tissue. Second, the response is critical for the development of adaptive immunity against infectious organisms. Its “adjuvant effect” on antigen presentation to T cells results in their response to the antigen as an invader. Finally, control and resolution of the inflammatory response promotes eventual healing, with a combination of regeneration, remodeling, and scarring.
Several general concepts about inflammation deserve mention. First, when it functions appropriately, the inflammatory response is a localized phenomenon. The reaction may either occur within a microscopic domain, such as a Ghon complex within a lymph node, or occupy an entire body cavity, as occurs during peritonitis. Though the extent of the response is much broader in the second example, the host has evolved measures to contain inflammatory mediators within the focus, and prevent their systemic spillover (11). A second, related idea is that generalized activation of even a single component of the inflammatory response can be lethal to the host. A ruptured appendix, for example, may trigger pulmonary endothelial activation, neutrophil influx into remote alveoli and the development of acute respiratory distress syndrome (ARDS), a nonsurvivable condition without intensive care. The third notion is that the acute inflammatory response is invariant and stereotyped. While there is considerable variability in extent and intensity of the reaction depending on the degree of insult, in general the host’s innate immunity will use the same mechanisms and kinetics to sense and respond to repeated injuries.
Inflammation is also an integrated biological response, the overall control of which is still poorly understood. Distinctions between soluble or humoral components, cellular mediators, and the neuroendocrine systems represent attempts to tease out components of an interdependent reaction pattern, closely related to the stress response that has evolved over billions of years. Most attempts at comprehensive descriptions of the host response to infection and injury remain phenomenological, and only recently have investigators applied a more system-oriented approach to try understanding of aspects of innate immunity (e.g., the monocyte-phagocyte system or neutrophil biology) (12,13,14,15).
Finally, different schemata have evolved to categorize inflammation. We distinguish between acute, subacute, and chronic inflammation to group inflammatory responses according to onset and duration. Effector mechanisms based on pathological description of an inflammatory lesion (neutrophilic infiltrative, caseating, necrotizing, histiocytic) provide another basis for distinction. We also describe inflammatory responses based on the inciting injury mechanisms, such as infectious, immune complex, trauma, hypoxic-ischemic, though these descriptors provide little insight into the type of specific inflammatory response they trigger.
Essential Elements of a Host Defense System
A host response to insult must accomplish a minimum of five functions. It must first detect a threat and determine whether tissue injury or microbial invasion has occurred. Second, an effective system must be able to alert the appropriate effectors, both local and distant from the lesion. These sensing and alerting functions constitute the afferent limb of the response. Third, in the efferent arm, an effective system must contain the injury or infection, minimizing the chances that microbes, the contents of necrotic cells, or the toxic endogenous mediators elaborated at the site of inflammation spill into the blood and lymph or spread throughout natural cavities or along tissue planes. Fourth, the response should eliminate the invading organism and/or damaged or dead tissue. Fifth, it must be able to cycle off and initiate the healing process. Vertebrates have also evolved the ability to prevent productive reinfection via lymphocyte-mediated immunological memory, a sixth function absent in most other multicellular organisms. Innate immunity is critical to each of these functions, but it collaborates with adaptive immunity in eliminating some pathogens and in the generation of a memory response.
The necessary components of a host defense system must subserve the five functions outlined above, possessing, at minimum, three capabilities. The first is an injury-sensing apparatus. Without the ability to sense damage to its integrity, the host cannot engage in responses to prevent further injury and repair the primary injury. Second, such a system must be able to initiate local protective responses and recruit distant support to reinforce the local response. Finally, it must incorporate controls that modulate the type, intensity, and timing of responses. Following consideration of the nature of injury signals, the remainder of the chapter examines how the mammalian host protects itself against infections and other injuries.
INJURY SIGNALS
The term “injury signal” carries inherent ambiguity. It can signify either a stimulus causing injury or a marker of tissue injury or invasion. The following discussion refers to the second
meaning, the indicator of possible damage. It is also convenient to distinguish between primary and secondary injury signals. Primary injury signals are generated or liberated by an insult that threatens the host. They can be molecules or forces (such as bacterial endotoxin, products of necrotic cell death, or thermal injury) that in turn generate endogenous, secondary injury signals (e.g., cytokines or neurotransmitters) that extend, amplify, propagate or terminate primary injury signals. This section focuses on the nature and identity of primary injury signals, while the subsequent two sections examine secondary injury signals.

Background
In Lives of the Cell, Lewis Thomas commented on the symbolic importance of injury sensing, hinting at the existence of an injury language.
It is the information carried by the bacteria that we cannot abide. The gram-negative [sic] bacteria are the best examples of this. They display lipopolysaccharide endotoxin (LPS) in their walls, and these macromolecules are read by our tissues as the very worst of bad news. When we sense lipopolysaccharide, we are likely to turn on every defense at our disposal.. There is nothing intrinsically poisonous about endotoxin, but it must look awful, or feel awful, when sensed by cells. (16)
Early attempts to understand illness recognized the association between rotting or putrefaction and its toxic effects on different hosts (5). By the mid-19th century, investigators had begun to isolate activities from dead bacteria that could sicken animals immunized against infection with them, suggesting that an inanimate property of a formerly live organism encoded a threat. Subsequent work on lymphocyte recognition of pathogens led Burnet, Lederburg, and others to propose a dichotomous antigen-sensing system in which lymphocytes that sensed self-antigens were suppressed, whereas those that recognized nonself persisted (17,18). Limitation of this “either-or” code and the acknowledgement that effective vaccines required inflammation-causing adjuvants to ensure specific immunity prompted Janeway to propose that innate immunity recognized invariant attributes specific to classes of pathogens (“pathogen-associated molecular patterns,” PAMPs) via a germ line-encoded sensory system (“pattern recognition receptors,” PRRs) (19). This “stranger theory” failed to account for certain phenomena, such as autoimmunity and the temporary tolerance of the pregnant female to the foreign fetus within her body. Matzinger (20) later articulated the “danger theory,” which proposed that the threat or presence of injury determined responsiveness more than the foreignness of the stimulus. Though this theory fails to account for certain realities of the innate immune response, it does highlight the role of tissue damage in initiating the inflammatory response (21).
Current Knowledge
Despite knowledge about the major injury mechanisms and their consequences, information about the molecular character of the injury signals they generate has emerged more slowly. The consequences of physical/mechanical (burn/scald, temperature extreme, electrical, radiation, atmospheric pressure), toxic (metabolic poison, free radical), ischemia-reperfusion, autoimmune, or infectious injuries have been extensively documented in clinical and experimental literatures. Many of these injuries can cause extensive tissue destruction, which in itself can pose a threat to survival through secondary injury such as exsanguinating hemorrhage, unconsciousness, airway obstruction, or critical organ dysfunction (e.g., status epilepticus, myocardial depression, renal failure). With the exception of microbial invasion and infection, however, the molecular identities of primary signals produced by these injuries have been more difficult to characterize, though considerable progress has occurred in the last two decades.
So what, precisely, is known about primary injury signals, what is suspected and what remains, at this time speculative? First, many injury signals from bacteria, fungi, and viruses have been isolated and chemically characterized. Although Janeway referred to them as “patterns,” they are, above all, molecules or domains within molecules (6), and they belong to most major macromolecular classes, including carbohydrates, lipids, nucleic acids, proteins, as well as compound macromolecules such as glycolipids, lipopeptides, and glycoproteins. LPS, for example, is a complex, phosphorylated glycolipid that composes ˜75% of the outer leaflet of the outer membrane of Gram-negative bacteria. Although different species have varying patterns of LPS acylation and glycosylation, the lipid A moiety activates the innate immune system (22,23).
Second, the presence of these molecules alone does not by itself represent a threat to the host. Facultative anaerobic Gramnegative commensal species in the human GI and respiratory tracts synthesize similar hexacylated, lipid A-containing lipidcontaining poly- and oligosaccharides, putative “mucosal” LPSs that are best recognized by the CD-14/TLR4/MD2 receptor apparatus. Their presence in the oropharynx or intestine does not trigger an ongoing inflammatory response, and in fact some evidence exists to suggest that their presence actually protects the host (R.S. Munford, personal communication, 2006). Moreover, as will be discussed briefly, appearance of one or a few of these microbial products outside of the their usual domains does not trigger a full-blown injury response, but rather indicates a possible threat and initiates a preparative or priming response that can be escalated should the host encounter additional evidence of microbial injury or tissue destruction (14).
Third, as discussed in the following section, the mammalian injury-sensing system depends on both redundancy and complexity to generate a repertoire of effector responses. For example, different signals from very different organisms or injuries activate overlapping, sometimes identical, signaling pathways. Molecules from viruses, Gram-negative and Grampositive bacteria, and fungi activate the TLR/IL-1 signaling pathway and NF-κB transcription factor and lead to similar cellular responses, such as cytokine and chemokine production, and cell surface marker changes. Moreover, the same injury molecule can elicit different responses, depending on how it is presented. Flagellin (bacterial filament protein) in the extracellular space activates TLR5 and NF-κB and TNF-α secretion, while its intracellular injection into a host macrophage by a microbial Type III secretion system triggers the ICE proteaseactivating factor (IPAF) inflammasome and massive IL-1β and IL-18 release (14).
The existence of primary injury signals of endogenous origin has been postulated, but their exact identity has been more difficult to document, in part because of the difficulty in conducting experiments free of contaminating endotoxin (24). A few molecules of host origin, however, appear to qualify as such. The most convincing demonstration to date of an endogenous primary injury signal has been the biochemical purification of a low-molecular-weight activity from dying cells that augments an adaptive immune response. Investigators identified uric acid as the signal and demonstrated its biological activity as an injury signal occurred when its concentration approached crystal formation, suggesting that a phase transition from soluble to insoluble (as monosodium urate crystals in the extracellular space) leads to cellular uptake, activation of caspase-1, and IL-1β release (25,26).
Extracellular matrix (ECM) degradation products may also function as primary injury signals. Separate groups identified the immunostimulatory potential of soluble hyaluronic acid (HA) fragments, the breakdown products of solid-phase polymeric HA (27,28), later demonstrating TLR4- and TLR2-dependent signal transduction (29). Thus, alterations to the ECM, normally immunologically silent, precipitated by injury may convert this and other macromolecules, such as biglycan and heparin sulfate, into injury signals (24).
Additional candidate primary injury signals include the chromatin-associated protein HMGB1 (high-mobility group box 1 protein) and extracellular ATP. The former is an ancient DNA-binding protein that stabilizes nucleosomes and
functions as an activating transcription factor. More recent studies demonstrate that its secretion from activated macrophages and dendritic cells, interaction with the RAGE receptor (receptor for advanced glycation end products), and localized inflammation confers a cytokine function on it. However, demonstration of its release from necrotic and pyroptotic cells and TLR2- and TLR4-dependent initiation of an innate immune response also suggests a role as primary injury signal (30,31).
functions as an activating transcription factor. More recent studies demonstrate that its secretion from activated macrophages and dendritic cells, interaction with the RAGE receptor (receptor for advanced glycation end products), and localized inflammation confers a cytokine function on it. However, demonstration of its release from necrotic and pyroptotic cells and TLR2- and TLR4-dependent initiation of an innate immune response also suggests a role as primary injury signal (30,31).
ATP released from necrotic and pyroptotic cells accumulates in the extracellular space following tissue injury and is a potent marker of tissue injury. Signaling through the lowaffinity P2X7 extracellular receptor and pannexin leads to potassium efflux and triggers assembly of one or more inflammasomes (see below) in innate immune cells. It is a key factor in critical caspase-1-dependent responses, including pyroptosis and the processing and release of mature IL-1β and IL-18. Macrophages stimulated by LPS, for example, accumulate large amounts of intracellular pro-IL-1β, and pro-IL-18, but a second signal in the form of ATP acting on the P2X7 receptor is required to activate caspase-1, which cleaves the procytokines and leads to their massive release of mature cytokine (32).
Summary
Although present knowledge of microbial molecules that trigger innate immune responses exceeds current understanding of endogenous injury signals, much remains to be learned about both. For example, in 18 short years, we understand the essential outline of how ligands activating the TLRs trigger a massive reprogramming of cellular gene expression through the NF-κB transcription factor, and have mapped many of those ligand-receptor interactions. Signal concentration matters as well, though less is known about how the host has evolved different thresholds for sensing different signals, and what those thresholds imply for subsequent responses. Picogram quantities of LPS trigger TNF-α production, while milligram amounts of uric acid, at least locally, are required for its crystallization to an activating form in physiologic solutions. Finally, the identity, characterization, and classification of novel injury signals may uncover unexpected strategies used by the host to distinguish normal homeostasis from a threat, some of which may represent minute deviations from the range of normal concentration or activity. For example, the mammalian equivalent of the plant “guard hypothesis,” in which intracellular sentinels targeted by viral proteins are under the surveillance of proteins that can detect viral modifications and mount a resistance response when these changes are detected, has yet to be described in mammalian innate immunity.
CELLULAR RECOGNITION AND RESPONSE TO INJURY SIGNALS
Characteristics of an Injury-Sensing Apparatus

A third characteristic, that injury sensors be promiscuous (capable of detecting more than a single signal), is neither required nor universal, but would maximize genetic economy. TLR2, for example, senses lipoteichoic acid, porins, and lipoarabinomannan in bacteria, zymosan and phospholipomannan in fungi, tGPI-mutin in trypanosomes, and measles virus hemagglutinin protein (33). This ability to recognize more than one activator, particularly when coupled with the ability to associate with different family members (e.g., when TLR2 and TLR6 combine to detect lipoteichoic acid), provides the host with an extended recognition spectrum from a limited number of genes.
A final, required feature of the host sensory apparatus is the sequestration of sensors from signals in the uninjured state. Nature accomplishes this separation through different strategies. Epithelial barriers, for example, constitute the most effective means of partitioning microbial invaders and their sensors, but plasma membranes accomplish the same purpose at a cellular level, particularly if intracellular molecules signal cellular injury when released. Breakdown products of ECM, prevalent in some rheumatic diseases may also be recognized as injury, while the polymeric forms are immunologically inert (21,28
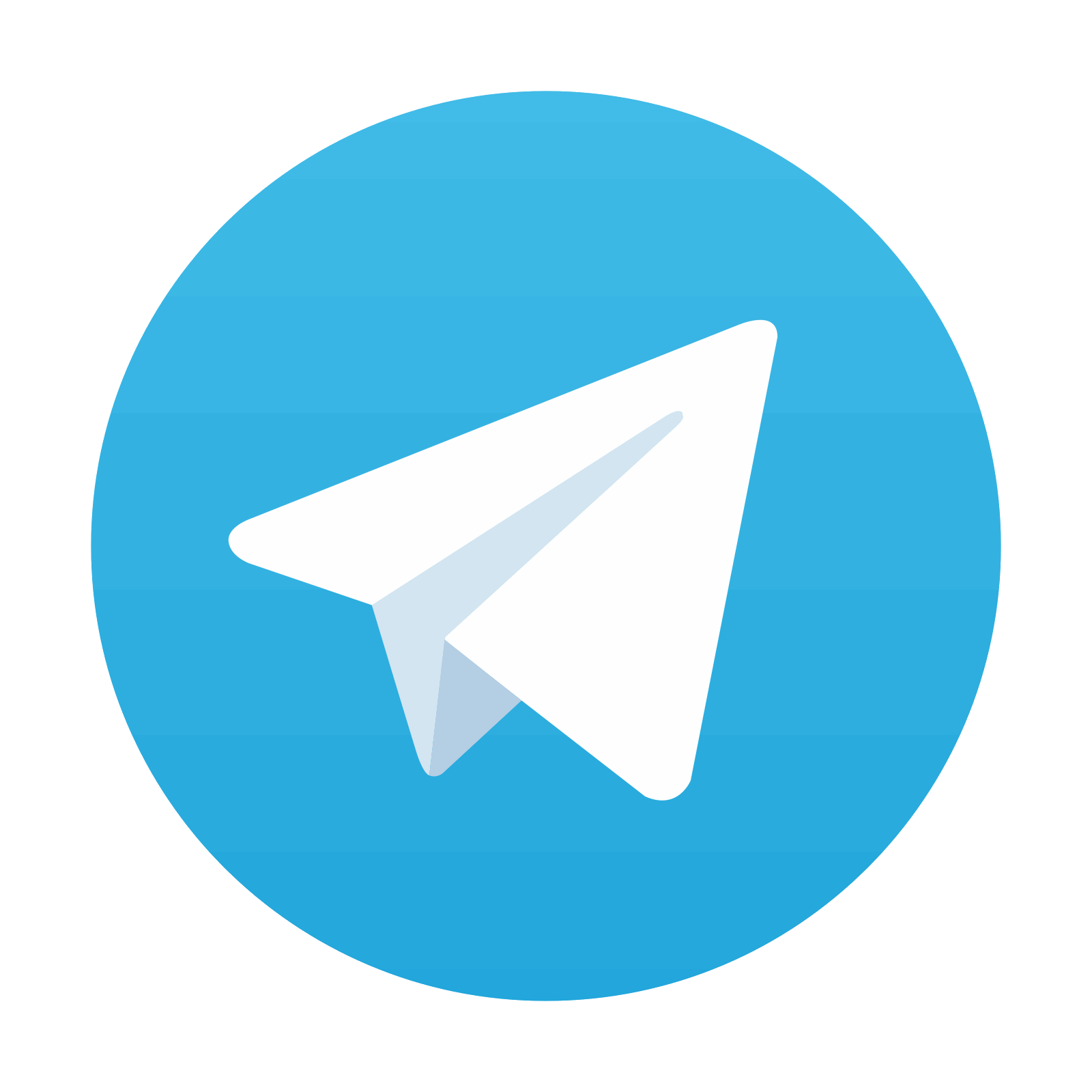
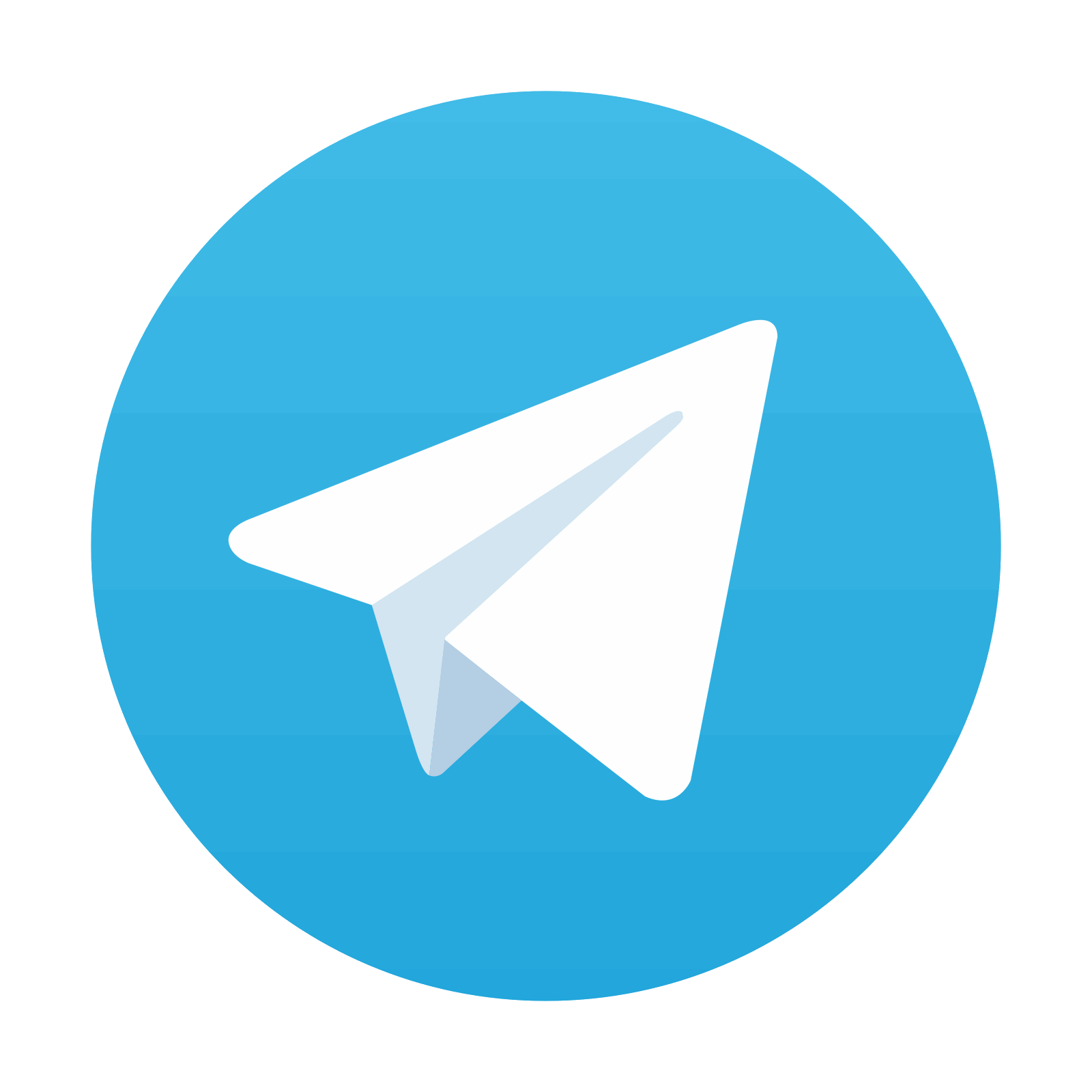
Stay updated, free articles. Join our Telegram channel

Full access? Get Clinical Tree
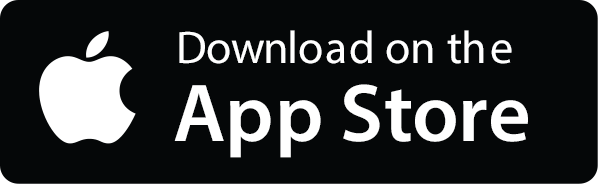
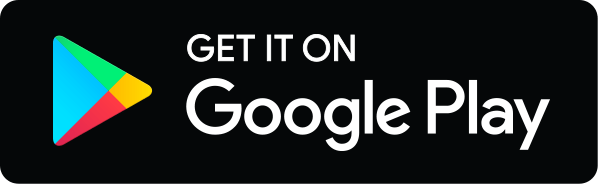
