MAJOR FACTORS AFFECTING UPTAKE
Solubility
• Partition coefficients express relative solubility of anesthetic gas at equilibrium
• Lower partition coefficients imply ↓ solubility, faster equilibration of partial pressure (alveolus ↔ blood ↔ brain), rapid induction (e.g., desflurane)
• Higher partition coefficients imply ↑ solubility, slower equilibration as more molecules are dissolved in blood, prolonged induction (e.g., halothane)
• Tissue: Blood partition coefficient = time for equilibrium of tissue with arterial blood
Cardiac Output
• Increased cardiac output results in faster uptake but ↓ alveolar concentration (Fa) and therefore slower induction (more blood passing through lungs = anesthetic is carried away faster).
• Effect is less pronounced for insoluble agents
• Note: Slower induction with R → L cardiac shunt due to no uptake of agent in shunted blood → dilution of arterial concentration despite faster ↑ in alveolar concentration (Fa); least soluble agents are affected most
Alveolar-Venous Concentration Gradient
• Depends on uptake by desired (brain) and undesired (fat, muscle) tissues
• Tissue uptake is determined by partition coefficients and regional blood flow
• Less tissue uptake means blood returns to alveolus with higher partial pressure, thus alveolar concentration (Fa) can ↑ faster
OTHER FACTORS INFLUENCING UPTAKE
Concentration Effect: Increasing the inspired concentration of a gas results in a disproportionate ↑ in the alveolar concentration (Fa); most clinically significant with N2O, as can be used at ↑↑ inspired concentrations than volatile anesthetics
Second Gas Effect: Large-volume uptake of the first gas (classically N2O) causes ↓ total gas volume in alveolus, thereby ↑ alveolar concentration/accelerating uptake of second gas (volatile agent)
Factors that Speed Rate of Induction (↑ Fa/Fi)
• Use of agents with ↓ solubility (low partition coefficients)
• Low cardiac output with minimal R → L shunt and preserved cerebral blood flow
• Increased alveolar minute ventilation, ↑ inspired concentration of agent, ↑ fresh gas flow rate (replaces anesthetic taken up in bloodstream)
• Pediatric pts → faster induction due to ↑ alveolar ventilation, ↓ FRC, ↑ % of blood flow to brain
ELIMINATION/RECOVERY
• Reduction of anesthetic in brain tissue is via exhalation >> biotransformation > transcutaneous loss
• Biotransformation via P-450 enzymes more important for halothane (20%) than sevoflurane (5%), isoflurane (0.2%), or desflurane (<0.1%)
• Recovery expedited by high fresh gas flows, elimination of rebreathing, low absorption by the circuit, decreased solubility, high cerebral blood flow and ↑ minute ventilation
• Context-sensitive elimination time: Longer duration of anesthetic is associated with longer time to recovery; over longer time more anesthetic is deposited in undesired tissues and must be “washed out”; effect more pronounced with ↑ solubility of agent (see Fig. 2A-2)
Figure 2A-2. Solubility and duration of use affect rate of recovery from inhaled anesthetics.
DIFFUSION HYPOXIA
• High concentrations of relatively insoluble gasses (N2O) diffuse out of the blood and enter the alveolus, displacing and reducing alveolar concentration of O2 and CO2
• Dilution of alveolar O2 can lead to hypoxia, dilution of CO2 can ↓ ventilatory drive and worsen hypoxia
• Administer high-flow 100% O2 for 5 to 10 min after discontinuation of N2O
MINIMUM ALVEOLAR CONCENTRATION (MAC)
• Unitless value comparing potency of inhaled anesthetic agents
• Reference point (1 MAC) = alveolar concentration at which 50% of patients will not move in response to a standardized surgical stimulus; analogous to ED50
• MAC values are roughly additive (i.e., 0.5 MAC of N2O plus 0.5 MAC of sevoflurane ≈ 1.0 MAC)
• MAC is greatest at 1 yr of age and reduced by 6% per decade of life
• At MAC 1.3, 95% of patients will not move in response to surgical stimulus
• MAC-BAR (1.5–2.0 MAC): Concentration which Blocks Adrenergic Response to nociceptive stimuli
• MAC-Aware (estimated 0.4–0.5 MAC): Concentration at which 50% of patients will not be forming long-term memory
• MAC-Awake (0.15–0.5 MAC): Concentration at which 50% of patients open eyes on command
CLINICAL CONSIDERATIONS OF INHALED ANESTHETICS
• Volatile agents may trigger malignant hyperthermia (MH) (see Appendix C)
• Agents in current use are nonflammable at clinical concentrations
• All potentiate neuromuscular blockade, degree varies with combinations of drugs/agents; effect of volatiles > N2O
• Carbon monoxide formed in reaction of volatile agents with desiccated CO2 absorbent, (desflurane > isoflurane >> halothane, sevoflurane); CO production ↑ with Baralyme, dry granules (classic example is Monday AM after O2 flows left on), ↑ temperature, ↑ concentration of agent
• Exothermic degradation reaction of sevoflurane in the presence of desiccated Baralyme linked to rare absorbent canister fires
SYSTEMIC EFFECTS OF INHALED AGENTS
• Cardiovascular:
• All volatile agents are dose-dependent CV depressants, though mechanism of ↓ BP differs (see Table, Differential Physiologic Effects of Inhaled Anesthetics)
• Heart rate effects vary with MAC and inspired concentration rate of change
• Pulmonary:
• All agents cause ↑ RR with ↓ TV, overall volatile agents cause ↓ in minute ventilation and ↑ resting PaCO2
• All blunt ventilatory response to hypoxemia (even at 0.1 MAC), volatile agents ↓ response to hypercarbia
• Volatile agents are potent bronchodilators
• Minimal inhibition of hypoxic pulmonary vasoconstriction (HPV)
• Neurologic:
• All agents ↑ cerebral blood flow causing ↑ ICP (especially halothane) and impair autoregulation of vascular tone (least with sevoflurane at <1 MAC)
• Volatile agents ↓ cerebral metabolic rate, N2O may ↑
• Desflurane and isoflurane at <1 MAC can suppress status epilepticus while ↑ sevoflurane concentrations associated with epileptiform EEG ∆
• All agents ↓ SSEP/MEP signals
• Hepatic: Halothane causes both hepatic artery vasoconstriction and ↓ portal vein flow (potential for hypoxic hepatic injury, ↑ LFTs), others preserve vascular supply better with ↑ in hepatic artery flow compensating for ↓ portal vein flow
• Renal: All cause ↓ renal blood flow, ↓ GFR, ↓ urine output without lasting dysfunction; untreated hypotension can cause acute kidney injury
INHALATIONAL ANESTHETICS, SPECIFIC COMMENTS
Nitrous Oxide (N2O)
• Key features: MAC of 104% precludes use as solo agent for surgical anesthesia; used at 30–70% concentration as adjuvant to IV or potent inhaled anesthetics. Low solubility = rapid onset/offset of action. Nonpungent, has analgesic properties
• Disadvantages: Rapidly diffuses into and expands air-containing cavities → avoid in air embolism, pneumothorax (75% N2O doubles size in 10 min), bowel obstruction, pneumocephalus, middle ear and retinal procedures; monitor ETT cuffs and PAC balloons for expansion
• Prolonged exposure → inhibits B12-dependent enzymes responsible for myelin and nucleic acid synthesis; megaloblastic bone marrow ∆ possible with >12–24 hrs use; neurotoxicity with repeated exposures (abuse)
• Increased homocystine levels possibly related to ↑ postoperative MI (ENIGMA trial; Anesth Analg. 2011 Feb;112(2):387–393)
• Teratogenic in animal models, no evidence in humans at clinical doses
• Not flammable, although does support combustion
• May ↑ PONV risk
• CV effects: Sympathomimetic, though direct myocardial depressant effect may prevail in hypovolemia, cardiac dx; ↑ PVR especially in patients with pre-existing pulmonary HTN
Isoflurane
• Key features: Inexpensive; slower onset/offset of action, pungent. Versatile use
• Disadvantages: Coronary vasodilator, potential for coronary “steal” effect (flow diverted away from vessels with fixed lesions) of uncertain clinical significance
Desflurane
• Key features: Most rapid onset/offset of action among volatiles; very pungent
• Disadvantages: High vapor pressure requires an electrically heated vaporizer (eliminates variation in delivery owing to ∆ in ambient temperature). Pungency may be irritant in patients prone to bronchospasm. Rapid increase or high MAC (>1.25) may cause transient but significant sympathetic stimulation
Sevoflurane
• Key features: Least pungent (best choice for inhalational induction); fast onset/offset of action; causes ↓ tachycardia than desflurane or isoflurane; does not sensitize myocardium to catecholamines
• Disadvantages: Controversial potential for nephrotoxicity due to metabolic production of fluoride ion and degradation to Compound A (nephrotoxic in animals). Compound A production ↑ with low flows, high concentrations of sevoflurane, desiccated barium lime absorbent; minimizing exposure recommended although studies have not shown nephrotoxicity in humans (if using flow rate of 1–2 L limit exposure to <2 MAC hrs; use >2 L for longer cases)
Halothane
• Key features: Low pungency (ideal for gas induction), inexpensive, ↑ cerebral blood flow > other volatiles, especially potent bronchodilator
• Disadvantages: Use ↓↓ due to rare but fulminant postoperative auto-immune hepatitis, CV depression and myocardial sensitization to catecholamines (↑ ventricular dysrhythmias)
Heliox (Helium–Oxygen Combination)
• Non-anesthetic gas mixture, commonly 70–79% helium + 21–30% O2
• Lower density of gasses (up to 2/3 ↓ than air + O2) promotes laminar flow, reduces turbulence in upper airway obstruction, asthma, COPD
• Helps ↓ pressures needed to ventilate pts with small-diameter ETTs; ↓ work of spontaneous breathing
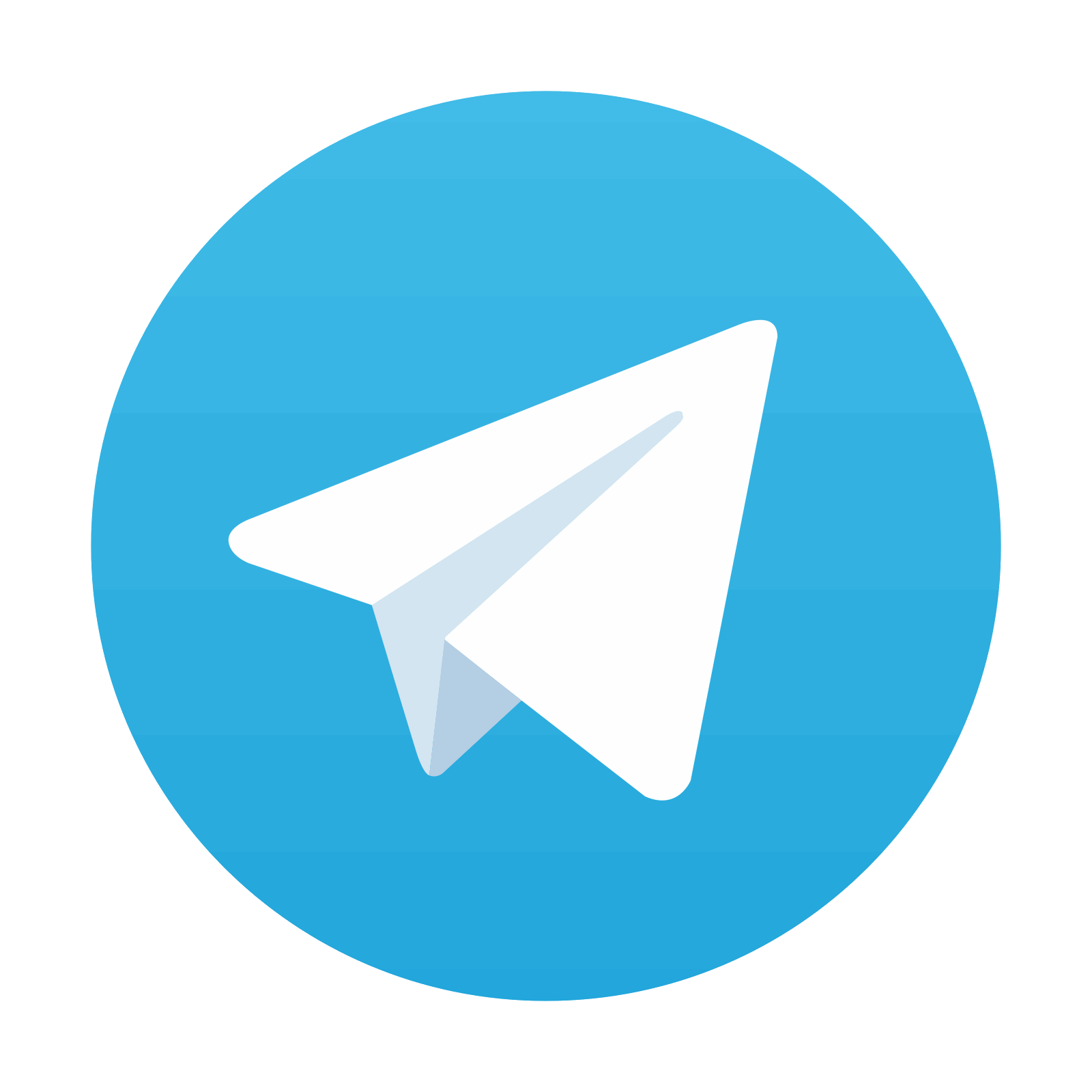
Stay updated, free articles. Join our Telegram channel

Full access? Get Clinical Tree
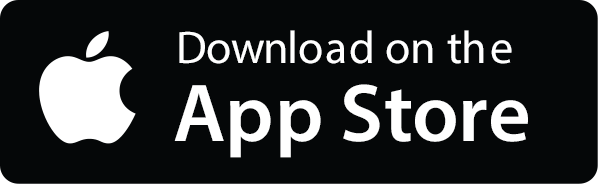
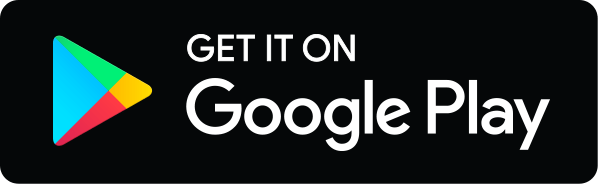