Chapter 19 Infusion equipment and intravenous anaesthesia
Evolution of infusion systems
This was superseded first by electronic drip counters, which were subsequently made to control the adjustable clamp on a gravity-fed giving set (infusion controllers) and, ultimately, by microprocessor-controlled infusion pumps (Fig. 19.1), able to generate flow irrespective of the effects of gravity, and incorporating many features such as sensing infusion line pressure and the facility for being programmed in a variety of units and even languages to give stepped infusions based on patient weight. The first devices to not rely on a drip counter used a syringe type cassette to reliably control the volumes delivered and were hence known as ‘volumetric pumps’ to differentiate them (Fig. 19.2). This design is less common now, and most devices rely on a peristaltic mechanism to push fluid along. The term should now probably be made obsolete to be replaced by ‘infusion pump’.
Microprocessor controlled/software driven
• simple ml/h request giving rise to the simplest infusion pump, say for ward use
• unit of drug per unit patient weight per unit time with variable units used for each; for example mg/kg/h or µg/kg/min. Clearly when dosing drug in this manner, the drug concentration in use and the patient weight must be input to the pump to allow automatic calculation of the flow rate. Such pumps may be used in the intensive care unit setting, but are more often termed ‘anaesthesia pumps’ as liberal discretion for choice of units is now usually only entrusted to anaesthetists
• simple infusion rate with an additional bolus volume when commanded by a separately attached control handle, thus constituting a PCA pump
• the in-built processor (or an additional piggybacked processor) can contain algorithms for the pharmacokinetics of particular drugs, allowing automated drug delivery based on achieving any desired theoretical patient plasma or effect site concentration of that drug. Such a configuration is termed a ‘target-controlled infusion (TCI) pump’. These systems are currently only commercially available for use in anaesthesia and are principally limited to four drugs: propofol, remifentanil, sufentanil and alfentanil. Patient variables such as age, sex, weight and height may need to be inputted to complete the algorithm. The algorithm, using this information together with the known drug concentration in the syringe, thereafter allows the anaesthetist to simply select a target patient concentration of drug. The pump automatically alters the infusion rate to most rapidly attain and maintain the calculated drug concentration in the patient at the set target. Such systems are very simple to use and obviate the need for complex calculations and detailed knowledge of pharmacokinetics by the user. They are largely responsible for a revolution in the administration of anaesthesia in a trend away from volatile agent maintenance towards total intravenous anaesthesia (TIVA).
Simple infusion systems
• simple fluid administration, no filter, droplet size approximately 15 drops/ml (0.07 ml)
• blood and fluid administration with clot filter at about 200 μm mesh size. These giving sets use a larger-bore tubing and a double drip chamber containing a float: by squeezing the bottom chamber, the float jams the inlet and acts as a one-way ball valve allowing fluid to be pumped. Droplet size is usually approximately 15 drops/ml
• burette, 100–150 ml in volume, droplet size either 15 drops/ml or, for paediatric usage, 60 drops/ml. A flap or ball valve at the bottom of the burette prevents air entering the drip chamber when the burette is empty
• platelet giving sets, designed to reduce the risk of aggregation in the giving set as would occur with conventional blood giving sets.
Some giving sets are now also designed so as to also be compatible with volumetric infusion pumps. This necessitates a narrower-bore tube made from softer plastic to function with the peristaltic pumps (see below). Flow rates are, therefore, lower and the tubing is less kink-resistant. These sets usually have a 15 μm filter at the base of the drip chamber and are not suitable for infusing blood or for use in adult resuscitation.
The rate of infusion in a simple gravity fed system depends on:
• the height of the fluid container above the infusion site
• the resistance to flow caused by the infusion set
• occlusion of the tubing from a rate controlling device
• the physical properties (i.e. viscosity) of the fluid to be administered
• the bore of the intravenous cannula
Rapid infusion
When rapid infusion of blood or other fluids is required, the rate of administration may be increased by the use of an inflatable pressure bag (Fig. 19.3), which may even be contained in a rigid box to increase the speed at which pressure may be applied.
When fluids are administered at a rapid rate, provision should be made for warming them to body temperature, otherwise significant cooling of the body may ensue (see Chapter 30).
Principles of infusion devices
The stepper motor
The driving force in the majority of infusion pumps and electronic syringe drivers is provided by an electronic stepper motor, which is directly controlled from a digital microprocessor system. The speed of a conventional electric motor driven from either an AC supply or a DC supply may vary with mechanical load, the voltage or the frequency of the supply. It is, therefore, difficult, without electronic feedback, to control such a motor accurately. The stepper motor (Fig. 19.4) is designed so that a series of pulses applied to the stator windings of the motor cause the shaft to rotate by a fixed amount for each pulse, typically 1.8°, 2.5°, 3.75°or 7.5°, irrespective (within certain limits) of the load. Infusion systems are designed so that a pulse generator, whose output frequency is varied by the microprocessor, can produce accurate control of an infusion by varying the speed of the stepper motor.
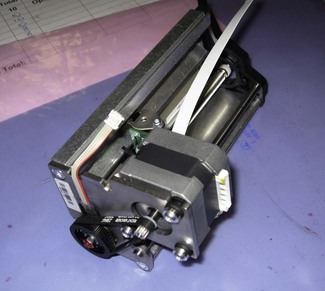
Figure 19.4 The stepper motor and lead screw assembly of an Alaris PK syringe pump during manufacture.
Infusion pumps
Cassette type
Originally, the most accurate (and expensive) infusion pumps used syringe type cassettes (see Fig. 19.2) and were referred to as volumetric infusion pumps. This, like the newer peristaltic pumps, is driven by a stepper motor controlled directly by a microprocessor. The volume of the cassette is typically about 5 ml, with the dedicated disposable ‘syringe cassette’ for each manufacturer’s pump being supplied separately as a sterile product. A valve operating in harmony with the piston directs flow from the infusate bag to the reservoir or from there to the patient. Fluid is drawn rapidly from the reservoir bag into the cassette in less than 1 s. The valve is then actuated such that on the piston upstroke the contents are expelled at the required rate into the patient, and the cycle is repeated. Although effectively this produces an intermittent flow, it also gives overall extremely accurate infusion rates, with only infrequent 1 s interruptions.
Peristaltic pumps
The principle of the peristaltic infusion pump is shown in Fig. 19.5. The tubing of a giving set is compressed by a series of rotating rollers or by a wave of mechanical ‘fingers’ or cam followers. The section of tubing in the peristaltic mechanism must be hard wearing, of known and consistent internal volume, and have no memory after compression so that it easily fills on being released. Depending on the manufacturer, specific proprietary tubing with dedicated fittings may be needed to allow it to be loaded into the pumping mechanism. Precision silicone tubing is often used in this section of the giving set.
Linear peristaltic mechanisms allow much easier loading of the infusion tubing and are now by far the commonest design of infusion pump. The driving force is again a stepper motor. The rotary motion from the motor is translated into a linear peristalsis by the use of cams and cam followers as shown in Fig. 19.6.
Because such infusion pumps have the theoretical capacity to inject limitless quantities of air into a patient should air ingress occur upstream of the pump (for example due to an empty infusion bottle), these devices incorporate sophisticated ultrasonics (Fig. 19.7) or optics-based ‘air in line’ detection systems capable of sensing air bubbles as small as 0.1 ml volume. These are usually placed downstream of the pump mechanism. Further protection is conferred by setting target delivery volumes smaller than the volume in the bag of infusate.
To detect obstructed or extravasated catheters, electrically powered infusion devices must have some measure of the pressure generated in the infusion line beyond the device. Peristaltic intravenous pumps, therefore, use a sensing piston pressing on the infusion line immediately downstream of the pumping chamber. This is calibrated to indirectly measure line pressure and can be programmed to alarm for occlusion at different pre-set levels.
Syringe drivers
There continues to be a range of small simple battery-operated syringe drivers (Fig. 19.8). The driving mechanism is a miniature DC motor that is switched on and off intermittently and drives a screw-threaded rod (lead screw), which is linked to the syringe plunger, causing its advancement. They may have a variable rate that is altered by adjusting a recessed control using a small screwdriver. These pumps are small and light enough to be worn in a holster by an ambulant patient and are now used chiefly for narcotic infusions for the relief of cancer pain. Great care must be taken in calculating drug dilutions and to ascertain that the correct units are used for setting the infusion rates, as the pumps are available in different models with rates set either as mm per 24 h or mm per h of plunger movement (Fig. 19.9).
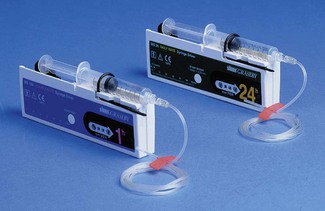
Figure 19.9 Graseby battery operated syringe drivers calibrated in mm per h and mm per 24 h.
Photo courtesy M Stewart, Graseby Medical Ltd.
Virtually all other syringe drivers for hospital use and particularly those used in intensive care and anaesthesia use microprocessor controlled stepper motors, again connected to the syringe plunger by a carriage on a lead screw (Fig. 19.10). Thus, each pulse applied to the stepper motor causes the advancement of the syringe plunger by a known amount. The pulse generator may be calibrated from 0.1 ml h−1 to 1200 or occasionally 1800 ml h−1, the higher rates being used only for delivering a bolus (often of predetermined volume) or for purging the infusion line.
Syringe pumps (the term is synonymous with syringe drivers) are now designed to automatically recognize a variety of syringes by virtue of the calibre of the barrel using some form of spring-loaded arm; some manufacturers’ models nonetheless require manual confirmation of the detector. Infusion line pressure (and empty syringe detection) is calculated indirectly from the force acting on the syringe plunger by sensors, which may be incorporated into the carriage or lead screw assembly (Fig. 19.11). This is a more popular option than the use of specialized infusion sets with in-built diaphragm and corresponding transducer housing on the syringe pump which remains largely confined to pumps used on neonatal ICUs. The facility in some devices to also alarm for low infusion line pressures is intended to allow recognition of disconnection of an infusion line (with the aim of, for example, preventing awareness in intravenous anaesthesia).
Rechargeable batteries
Although mains-driven, electrical infusion devices must have battery back-up both to cover mains failure and for patient transfer and emergency situations. The performance of the in-built rechargeable batteries is an important consideration when purchasing such equipment, but it must be remembered that this is also influenced by the battery maintenance procedures. Poor battery life can render otherwise excellent devices unreliable and unusable. Pumps should be kept connected to the mains when not in use and batteries should be replaced appropriately. Microprocessor-driven infusion devices are susceptible to bizarre error conditions when rechargeable batteries begin to fail.
Safety
Microprocessor-driven infusion devices are used for the administration of many potent drugs with narrow therapeutic windows where maladministration can have lethal consequences. The drugs are used in a variety of dilutions and dosed in units that can vary by several orders of magnitude (ηg/ml, µg/ml, µg/kg/min, mg/kg/h). The devices themselves are highly versatile and capable of being instructed to perform in many different manners. Given these factors it is evident that there is significant potential for user error with disastrous consequences.
The machines have built-in alarms for occlusion, low battery, mains failure, disengagement of drive mechanism, failure to load infusion set and other common fault conditions. In spite of this, they remain high-risk devices capable ultimately of delivering drugs dangerously: they have a recognized associated morbidity and mortality. In at least 27% of the 1495 incidents involving infusion pumps reported to the Medical Devices Agency in the UK between 1990 and 2000, the cause was found to be user error (including failure to maintain the device appropriately).1 Only in 20%, were problems device-related with issues such as performance, degradation, quality assurance and design and labelling. In the 53% of cases where no cause was established it is likely that a very large number represent user error. The MHRA report for the year 2009 again reports that infusion and feeding pumps ‘continues to be one of our busiest areas’ with 375 adverse incident reports.2 The user must, therefore, be ever vigilant and particularly aware of the following problems:
• Unitary programming errors. The simplest and most common error is a mistake or slip in selecting the correct dosing unit or drug concentration, e.g. mg/kg/min instead of µg/kg/min or µg/ml instead of mg/ml. These issues are addressed to a large extent by the use of drug libraries (v.i.).
• Wrong drug errors. Where more than one infusion pump is used particularly when they are controlled through a common interface it is relatively easy to confuse the drugs. Propofol available as identical looking 2% and 1% solutions is also easy to confuse especially as one manufacturer of open label TCI pumps allows a change in concentration during a TCI (Fresenius) and one does not (Alaris), and hence does not prompt for concentration when new syringes are loaded. The Diprifusor system automatically senses the drug in the prefilled syringe and hence does not have this problem but this may now predispose doctors to errors when using other TCI systems.
• Failure to restart infusion. This is a very common error after refilling syringes during intravenous anaesthesia. Software changes across generations of the same device may require additional key presses for the same function (Diprifusor) and can contribute further to this error.
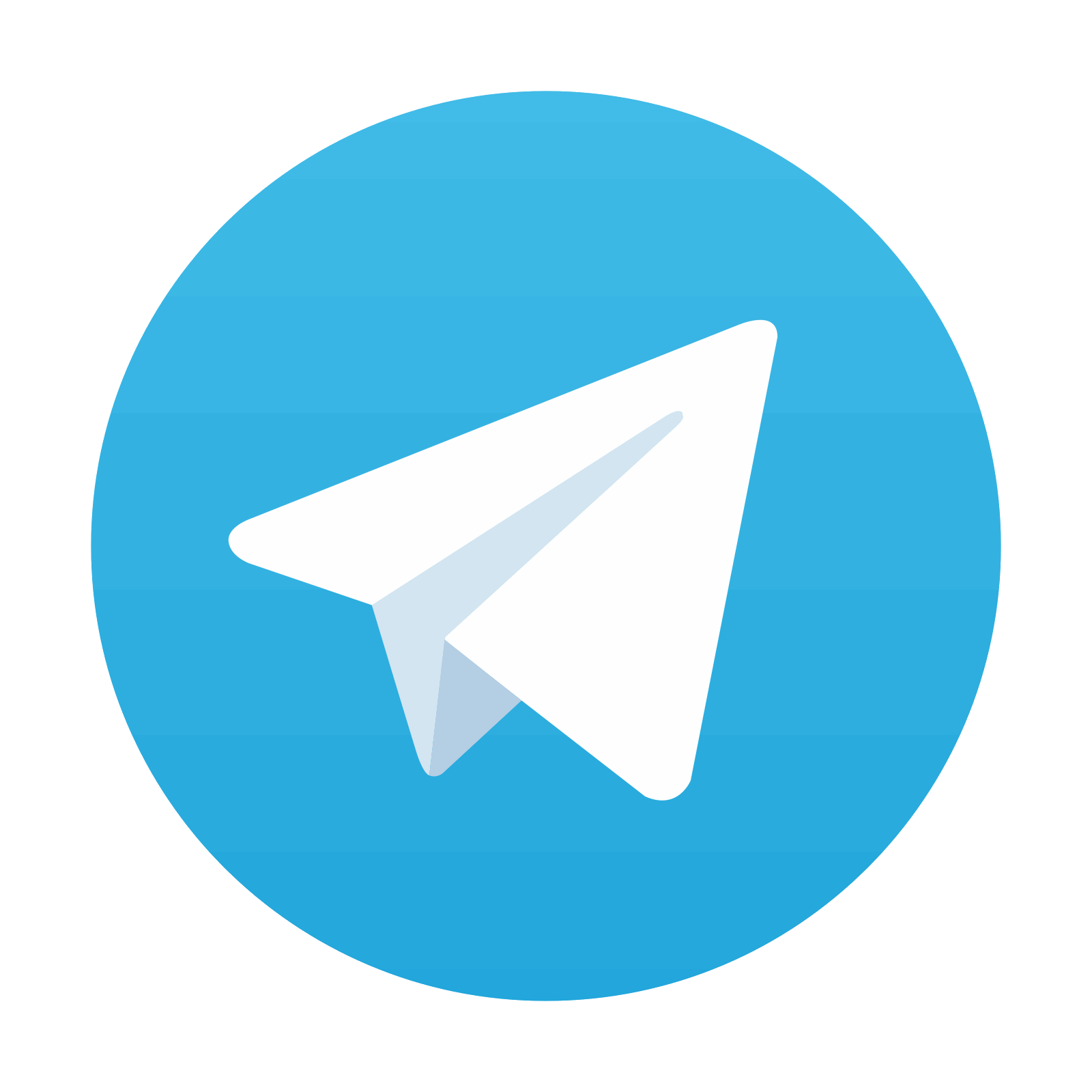
Stay updated, free articles. Join our Telegram channel

Full access? Get Clinical Tree
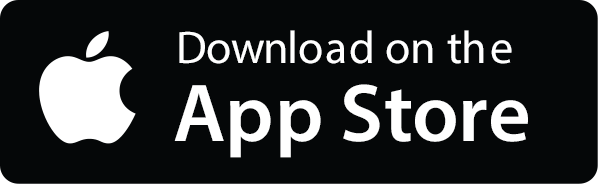
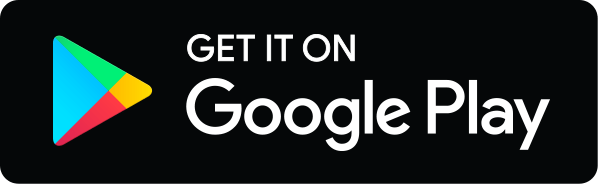