Pain is indeed an unpleasant experience; the perception of pain feels like damage or injury. Whether such damage is actual or potential, pain is usually described with the idioms of injury, including inflammation. The word itself conjures flames, fire, burning, redness, swelling…ouch! As students of the body, we know that inflammation refers to a complex biochemical process and is not a colloquialism to be used loosely. How often do patients describe their pain as swelling or fire, or ask “is it inflammation?” or “will this injection make the disc swelling go down?” How often are treatments variations of anti-inflammatories? Is inflammation truly there? This chapter serves as a review of evidence that there is a process of inflammation involved in some of the major conditions seen in the pain clinic: musculoskeletal pain and neuropathic pain. The general principles applicable are illustrated in detail by the specific examples of intervertebral disc disorders and complex regional pain syndrome.
Celsus’ ancient description of the cardinal signs of inflammation includes rubor, calor, tumor, and dolor. These are, respectively, redness, heat, swelling, and pain. Others, including Virchow, later added loss of function as part of the cardinal descriptors of inflammation. Two thousand years after Celsus, we are beginning to understand the biochemical substrates underlying the cardinal properties of inflammation. Increasingly, inflammatory biochemical profiles are being discovered in association with all afflictions, from Alzheimer’s to zoster. While disorders such as rheumatoid arthritis or gout have long been managed as inflammatory conditions, new knowledge of the inflammatory mechanisms underlying “noninflammatory” disorders gives hope that new treatments may be on the horizon.
Inflammation types include acute and chronic. The inflammation response may result from a variety of types of damage to body tissue; inflammation itself may perpetuate damage. Vascular response is a critical component of inflammation and includes vasodilation, increased small blood vessel flow adjacent to tissue damage, and increased permeability of blood vessels. These vascular changes are caused by chemical mediators histamine and prostaglandin, and the kinin and complement enzyme systems. Polymorphonuclear lymphocytes migrate first, then macrophages, through permeable blood vessels, and into the damaged tissue. Plasma proteins flow through permeable vessels into tissue, resulting in increased osmotic pressure there, and tissue edema by a process of exudation. Fibrinogen is converted into fibrin, which forms acute inflammatory exudate (and aids in clotting).
Chemical mediators are numerous in inflammation. Histamine causes the immediate dilation of vessels. Histamine is stored in mast cells, basophils, eosinophils, and platelets. Histamine release is activated by complement C3a and C5a and lysosomal protein from neutrophils. Complement proteins are glycoproteins that may form a membrane attack complex, and may amplify immune response. Serotonin is also a vasoactive mediator released by mast cells and basophils. Phospholipase, contained in cell membranes, produces arachadonic acid during inflammation. Arachadonic acid is rapidly formed into (1) prostaglandins, prostacyclins, thromboxanes, via the cyclooxygenase pathway, and (2) and leukotrienes and lipoxins, via the lipoxygenase pathway. Cytokines are a diverse group of locally acting glycoproteins with short half-lives that act on specific receptors to regulate cell function in inflammation. Cytokine categories include interleukins, interferons, tumor necrosis factors, growth factors, and chemokines.1
Enzyme systems involved in inflammation are alluded to earlier. The four major enzyme systems are complement proteins, kinins, coagulation factors, and the fibrinolytic system. The complement system may be involved in tissue necrosis, may be activated by antigen–antibody complexes in the classic pathway (or endotoxin is in alternate pathway), and are involved in the opsonization of bacteria. The kinins, such as bradykinin, are activated by coagulation factor XII and are mediators of vascular permeability and pain.
Many cell types are involved in inflammation. B lymphocytes secrete the antibodies that bind antigens to form a complex that activates complement. T lymphocytes secrete the cytokines and chemokines. Neutrophils, basophils, and eosinophils are known as granulocytes. Neutrophils are polymorphonuclear leukocytes (PML) and are the main cell of inflammation response, being the first cells drawn to the site of inflammation. They have granules containing cytotoxic substances as well as tissue remodeling substances. Neutrophils are next to respond, and both synthesize and are regulated by cytokines. Arachadonic acid and derivatives such as leukotrienes and prostaglandins are abundant in neutrophils. Macrophages derive from mononuclear phagocytes, known as monocytes. Macrophages may be primed, with enhanced major histocompatibility complex (MHC) class II expression. Such activated macrophages are important producers of inflammatory mediators such as arachadonic acid and derivatives. Macrophages play a role in neovascularization when activated by low oxygen conditions, such as in wounds, or cytokines. Mast cells occur in connective tissue typically (versus circulating in the bloodstream). The process of degranulation is the release of inflammatory mediators, contained in cytoplasmic granules, from mast cells and basophils. Mast cells and basophils are known for roles in allergic reactions. Eosinophils are leukocytes recruited to tissue sites of immune reaction; these are also known for allergic states. Chronic inflammation may differ from acute inflammation, although both may be present simultaneously; chronic inflammation may involve a predominance of monocytes and lymphocytes, rather than granulocytes.2
One of the most common examples of musculoskeletal pain is the painful intervertebral disc. Disc degeneration is actually a normal, expected part of the human experience. Disc degeneration is not always painful, but there may be increased likelihood of pain in those with magnetic resonance imaging (MRI) evidence of disc degeneration.3 Clinical, animal, and tissue studies have implicated a number of inflammatory substances in painful disc disorders. In association with inflammatory processes, herniated nucleus pulposus alters nerve conduction and discharge, causes nerve fiber degeneration, alters intraneural capillary permeability and blood flow, and induces an inflammatory cascade.4
The normal structure of the intervertebral disc follows from its components, resulting in an orderly functional arrangement. The normal disc morphology allows the performance of its physiological functions, including movement and load bearing. In early life, the disc is arranged as a very ordered structure: It is comprised of a central gelatinous nucleus pulposus contained within a series concentric bundles of collagen fibers, which make up the annulus fibrosus.5 With age and variations of stress through load bearing, the collagen bands (lamellae) develop increased complexity, including variations in the number and size. Thus, throughout life, as more stress is placed on the disc, there is a perpetuating cycle of disruption to disc morphology and cell organization resulting in degeneration.5
The lumbar intervertebral disc has a molecular and cellular makeup similar to articular cartilage. The nucleus pulposus’ matrix is a combination of type II collagen, proteoglycans, and noncollagenous proteins synthesized from chondrocyte-like cells. The annulus fibrosus is primarily type I (and includes type II) collagen produced by fibroblast-like cells.6 The proteoglycans consist of a central protein from which there are branching chains of glycosaminoglycans. Multiple proteoglycans are joined to a hyaluronic acid chain to form aggregates, held together by type II and IX collagen.7 The proteoglycan matrix has hygroscopic properties which allow it to hold water molecules giving the nucleus hydrostatic properties, and thus, the nucleus can bear compressive forces. The matrix components fluctuate and are continually degraded by enzymes called the matrix metalloproteinases (MMPs).8–10 Intervertebral disc herniation is likely preceded by physiologic degeneration of the disc caused by MMPs.11
Disc degeneration is a biochemical process involving altered rate of matrix breakdown and synthesis, change from type II to type I collagen, decreased aggrecan, and chondrocyte death.12 Matrix metalloproteinases (MMPs), aggrecanase, and interleukin 1 (IL-1) have been strongly implicated in the degeneration, inflammation, and tissue destruction of the intervertebral disc.13 IL-1 is the stimulator of chondrocytes to produce the matrix-degrading MMPs.8,14,15 MMPs degrade matrix and produce matrix fragments, which in turn propagate further tissue destruction by activating cell-signaling pathways.16 Cytokines, such as IL-1, interferon (IFN), and tumor necrosis factor-α (TNF-α) are produced by macrophages that invade the disc in response to injury.17 The ability to invade the disc is due to the increased vascularity that develops as the normally avascular disc in the healthy adult becomes increasingly vascularized with degeneration. This leads to an increased supply of molecules such as cytokines.5,18 Cytokines cause secretion of growth factors by disc cells, leading not only to this neovascularization, but also to an ingrowth of nerve fibers, altered sensitivity of nerve fibers, and granulation tissue formation. Macrophages secrete superoxide (O2−), which causes degradation of the proteoglycans, forcing them to deaggregate. That is, glycosaminoglycans of the extracellular matrix, especially aggrecan, are broken down by enzymes such as aggrecanase. Furthermore, superoxide will inhibit chondrocyte proliferation and synthesis. TNF-α and IL-1 stimulate the production of produce nitric oxide, which affects the matrix components directly via promotion of matrix degradation and inhibition of matrix synthesis.19 Degeneration includes the production of abnormal components of the matrix and an increase in the mediators of matrix degradation (IL-1, TNF-α, superoxide, nitric oxide, MMPs).20,21
Herniated intervertebral disc tissue has been shown to produce a number of proinflammatory mediators and cytokines, although studies are not without controversy. Specifically, herniated intervertebral disc specimens have been shown to produce increased amounts of nitric oxide, interleukins-6 and 8 (IL-6 and IL-8), prostaglandin E2 (PGE2), and matrix metalloproteinases.19,22 Burke and colleagues demonstrated a statistically significant difference between levels of production of IL-6 and IL-8 in the herniated discs of sciatica and low back pain groups (p < 0.006 and p < 0.003, respectively) compared to nonherniated discs.22 These inflammatory markers found in disc tissue suggest that production of proinflammatory mediators within the nucleus pulposus may be a cause of the painful intervertebral disc. However, a study of cerebrospinal fluid in patients with lumbosacral radicular pain demonstrated no difference in IL-6 or TNF-α, and only 12 of 39 patients had an increase in IL-8, and, of those, no correlation was found with pain intensity.23 Another study of cerebrospinal fluid concentrations of IL-1β, IL-6, and TNF-α found only increased concentration of IL-6 in patients with cervical myelopathy and lumbar radiculopathy but no clinical correlation involving the concentration of IL-6.24
Some studies do suggest a quantifiable relation of proinflammatory mediators with degree of disc abnormality. Using magnetic resonance imaging, Kanemoto and colleagues determined that MMPs were significantly correlated with the grade of intervertebral disc degeneration, and levels of MMPs observed in prolapsed lumbar intervertebral discs were significantly higher than that in nonprolapsed discs. Such a relation has also been demonstrated in cervical intervertebral discs, in that MMPs have been correlated with the size of osteophyte formation.21,25
Herniated disc tissues demonstrate high activity—for example, a 20- to 100,000-fold increase—of inflammatory phospholipase A2, revealing the occurrence of inflammation and an immune reaction in disc herniations.26 Prostaglandins and leukotrienes are strong mediators of inflammation and are produced when phospholipase A2 releases arachidonic acid from cell membranes, causing inflammation, in this case at the site of disc herniations.27 Cytokines induce phospholipase A2 activation: Nygaard showed there is a significantly higher concentration of leukotriene B4 and thromboxane B2 in noncontained versus contained disc herniation.28 Therefore, varying inflammatory mechanisms are involved in different types of disc herniations. There are data suggesting that cumulative damage and elevated cytokine levels may be connected to incidence of low back pain: Ulrich demonstrated that recurrent disc injury results in persistent inflammation and enhanced disc degeneration.29
Inflammation of disc tissue may be a major factor in radicular pain. When the disc extrudes into the epidural space, it becomes like a foreign material and an immune response occurs, leading to an inflammatory cascade. The extruded discs express anti-interleukin-1, intracellular adhesion molecule-1, lymphocyte function-associated antigen, and basic fibroblast growth factor. These extruded discs express inflammatory mediators that could induce neovascularization and persistence of inflammation.30 Woertgen analyzed the discs of patients who had undergone discectomy and found that those who did better after having the herniated disc material removed had a statistically significant correlation between the histologically proven inflammation and the outcome, as shown by the pain grading scale.31 That is, when an inflamed disc was removed, the patient had a better outcome because it was likely the etiology of pain. Sequestered discs have been shown to be associated with a higher level of prostaglandin E2 content than extruded discs, while, in turn, extruded discs have been associated with higher prostaglandin E2 content than disc protrusions. Clinically, a positive straight leg raising test has been associated with a higher prostaglandin E2.32
Inflammatory markers have also been demonstrated in lumbosacral spinal stenosis. Cerebrospinal fluid concentration of IL-6 is increased in lumbosacral spinal stenosis, but studies are not consistent in correlating this with pain severity for this function.33
Animal model evidence supports the notion of inflammation in disc herniation and radiculopathy. In animal models, TNF-α causes neuropathologic injury in nerve roots and neuropathic pain, as when exogenous TNF-α is applied in vivo to rat nerve roots, resulting in behavior deficits that mimic experimental studies with herniated nucleus pulposus applied to nerve roots.34 Also, TNF-α inhibitors prevent the injury-associated reduction of nerve conduction velocity and intraneural edema formation.35 In a pig study of radiculopathy, wherein study animals had spinal nerve roots exposed to exogenous nucleus pulposus, application of aminoguanidine, a nitric oxide synthase inhibitor, significantly reduced the injurious effect of nucleus pulposus on nerve-conduction velocity in spinal nerve roots. Nucleus pulposus exposure (disc herniation) increases nitric oxide synthase activity in spinal nerve roots, and by inhibiting nitric oxide synthase, nucleus pulposus–induced edema and abnormalities in nerve-conduction velocity are countered. Such activity strongly suggests that nitric oxide, an inflammatory marker, is involved in the pathophysiology of the nucleus pulposus in disc herniation.36 Other inflammatory markers are also implicated. For example, Kawakami demonstrated that thromboxane A2 and leukotriene B4 result in the hyperalgesia induced by application of nucleus pulposus to the lumbar nerve root in the rat. He applied thromboxane A2 synthetase inhibitor and leukotriene B4 receptor antagonist by injection into the epidural space and demonstrated decreased mechanical hyperalgesia after such epidural injection.37
Regulation of gene expression via signaling pathways may be involved in a variety of inflammatory conditions, including intervertebral disc disorders. For example, NF-κB and MAP kinases are known to be involved in disc degeneration. Expression of the proinflammatory cytokines such as TNF and interleukins are all upregulated by NF-κB. MAP kinases may be involved in maintenance of the extracellular matrix of the disc by regulation of anabolic and catabolic gene products. Targeting NF-κB and MAP kinases may be promising for future therapies for disc disorders.38
The preceding is a selection of human and animal evidence demonstrating that inflammation is involved in disc-related pain and degeneration. There is evidence of inflammation in disc disorders, in spite of the fact that the disc is a relatively avascular structure. In fact, it may be the inflammatory component of disc herniation or disruption, and not the physical or compressive component, that is most important in pathology of disc disorders. However, the existing evidence is not entirely consistent. It is rational to target the inflammation involved when treating pain related to discs not only with current modalities, but with future research. For example, studies have evaluated biomarkers as predictors in response to epidural steroid injection, and therapies involving gene, stem cell, and antibodies have been proposed.39,40 TNF-α inhibition has been studied as a therapy for disc disorders, in vitro and in vivo, with mixed results.39,41 There is also promising work under way on new MRI techniques that highlight inflammatory signals, which may distinguish painful discs versus nonpainful degenerated discs.42 In fact, many of the MRI features of degeneration can already be understood as markers of inflammation. The breakdown of the hydrophilic extracellular matrix leads to loss of water content, resulting in the “dark disc” and decreased disc height seen on MRI. Cytokines migrate through the end plates into adjacent vertebral bone, leading to inflammatory response there, including marrow edema, seen as Modic changes on MRI.
Neuropathic pain is a major cause of suffering treated by pain physicians, but it has often been considered as a special class of pain, in contrast with the more commonly understood “normal” or nociceptive pain. Per the revised definition of the Neuropathic Pain Special Interest Group of the International Association for the Study of Pain, neuropathic pain is “pain arising as direct consequence of a lesion or disease affecting the somatosensory system,” and may be graded as “definite,” “probable,” or “possible.”43 Common examples of neuropathic pain include postherpetic neuralgia, peripheral neuropathy, tic douloureux, and complex regional pain. What is often forgotten is that the lesion or disease affecting the somatic sensory system, resulting in neuropathic pain, may involve inflammation.
Immunologic pathways have a role in normal neurophysiology as well as pathologic states. Cells of both the central and peripheral nervous systems release cytokines and chemokines and have receptors for these molecules. For example, in response to injury, neurons produce molecules that activate microglia, which, in turn, produce cytokines and chemokines. The cytokines trigger the inflammatory cascade, while the chemokines induce chemotaxis of inflammatory cells such as monocytes, T cells, neutrophils, and granulocytes. Such immune activation is known to be associated with a wide spectrum of diseases of the nervous system, and a common feature is the previously discussed cytokine and chemokine activation.44
Tissue trauma may lead to both classic and neurogenic inflammation. Classic inflammation involves leukocyte release of cytokines such as IL-β, IL-2, and IL-6. In neurogenic inflammation, cytokines are released from neurons themselves. TNF-α may be elevated in neuropathic pain specifically presenting with allodynia; TNF-α further stimulates production of IL-1β and IL-6.45,46 Neurons also may release neuropeptides such as substance P, calcitonin gene–related peptide (CGRP), bradykinin. These substances also produce the cardinal signs of inflammation.47 Cytokines increase the amount of neuropeptide in primary afferents.48 Neurogenic inflammation follows excitation of these primary afferents, consists of plasma extravasation and vasodilatation, and is mediated by CGRP and substance P. CGRP facilitates vasodilation. Substance P induces protein extravasation.48 Nerve injury also leads to release of NGF, which is proinflammatory.48
When peripheral tissue is inflamed, sustained nociception induces cortex neuroplastic changes, or central sensitization. When peripheral nerves are injured, activated microglia produce cytokines and chemokines, which recruit neutrophils and macrophages and which themselves alter the blood–nerve barrier and directly damage nerves. For example, peripheral nerve excitability becomes increased directly by cytokines. In peripheral nerve injury states, Schwann cells can induce a T-cell and monocyte inflammatory response via production of chemokine CCL2. There are chemokine receptors in neurons such as primary afferents, dorsal root ganglia, and dorsal horn neurons. For example, in animal models, both peripheral tissue inflammation as well as peripheral nerve injury are associated with macrophage migration into dorsal root ganglia.49–51 In peripheral nerve injury, migration of inflammatory cells may continue into the central nervous system itself, as the blood–brain barrier is transiently compromised (also demonstrated in animal models).52,53 Chemokines such as CCL2 interact directly with receptors on these neurons, while also continuing to recruit inflammatory cells. Chemokine receptors may interact directly with μ-opioid receptors to increase pain. Interleukins accelerate neuropathic pain. For example, IL-1β sensitizes nociceptors in primary afferents, IL-1 induces substance P release in dorsal root ganglia, and IL-6 activates dorsal horn pathways after peripheral nerve injury.44 Thus, peripheral sensitization also results after tissue trauma. Peripheral primary afferents release mediators such as substance P and bradykinin, increasing the irritability of these nerves. Clinically, this results in hyperalgesia or allodynia.47
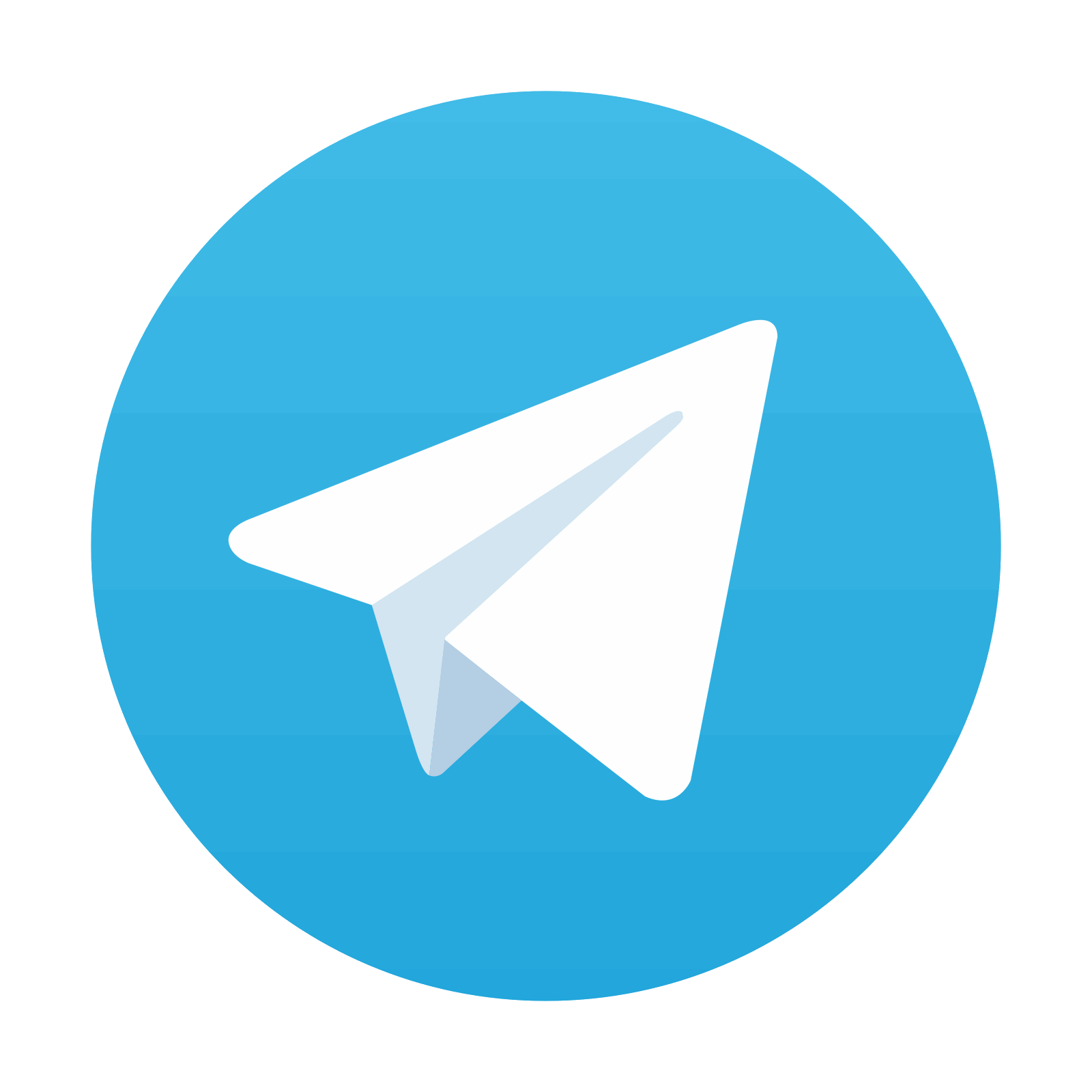
Stay updated, free articles. Join our Telegram channel

Full access? Get Clinical Tree
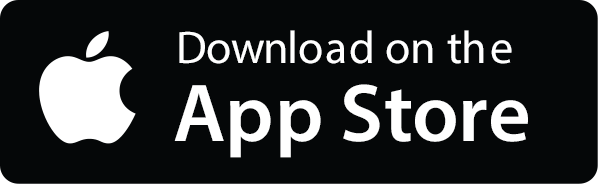
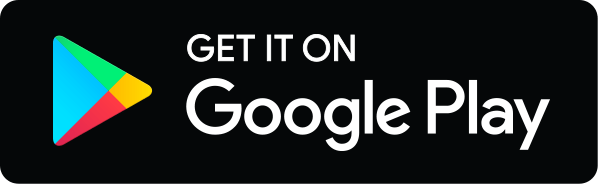