div class=”ChapterContextInformation”>
6. Permissive Hypotension
Keywords
TraumaPermissive hypotensionDamage control resuscitationRemote damage control resuscitationCrystalloidHaemorrhagic shockBlood failureCoagulopathyRestricted resuscitationDelayed resuscitationHypotensive resuscitationHybrid resuscitationBlood-based resuscitationIntroduction
Trauma patients are a complex population, with each individual requiring a unique management strategy tailored to his/her needs – the acceptance of a single global blood pressure target would appear to be the antithesis of this principle. – M. Wiles [1]
Permissive hypotension (PH) in the context of life-threatening haemorrhage (LTH) is a resuscitation strategy which is used within damage control resuscitation (DCR), in which a lower than normotensive blood pressure is targeted until the source of haemorrhage is controlled [2]. The perceived benefit of PH is that it limits bleeding and prevents dislodging of any clot formed at the injury site by avoiding excessively high blood pressures. This must be balanced against the dangers of an under-perfused bleeding patient who will suffer from increasing physiological burden of reduced oxygen delivery resulting in endotheliopathy, coagulopathy, acidosis and hypothermia which can ultimately lead to multi-organ failure to include ‘blood failure’ [3, 4].
The ultimate goal of DCR is the delivery of a casualty to higher echelons of care (i.e. a hospital with suitable surgical facilities available) with sufficient physiological reserve to survive definitive surgical haemostasis and further aggressive resuscitation. The paradigm of DCR has evolved over many iterations ultimately leading to the current practice of combined use of blood-based resuscitation and limiting systolic blood pressure (SBP) which is believed to be effective in promoting haemostasis and reversing shock.
This chapter will examine the evolution of PH and its part in DCR, the benefits and risks of PH and how future iterations of PH have started to evolve.
Evolution of the Management of Haemorrhagic Shock
Post-injury haemorrhagic shock remains the major cause of morbidity and mortality in patients suffering major trauma [5, 6]. Shock is a defined state of oxygen delivery that is inadequate to meet vital organ metabolic demands. Once perfusion falls and hence oxygen delivery of an organ falls below a sufficient level, anaerobic metabolism dominates, leading to an oxygen deficit at the cellular level. The product of the severity of shock and time spent in a shocked state is known as oxygen debt, which must be repaid. Failure to resolve this debt results in worsening organ dysfunction/failure, including blood failure, making ongoing resuscitation increasingly difficult and eventually impossible [7].
Emphasis on restoration of circulating volume to maintain perfusion remained the mainstay of clinical practice until the start of the twenty-first century. The basis of this was founded on canine models initially proposed by Wiggers [8, 9], who found that survival rates were poor following periods of prolonged severe haemorrhagic shock even after re-administration of blood that had been lost.
This was further developed by Reynolds [10] and Shires [11], who found that administration of whole blood with large amounts of crystalloid solution relative to the amount of blood loss (2 ml per 1 ml of blood loss) could result in the reversal of the state of shock and dramatically improve survival rates. This is the basis of the ‘3:1 rule’ where 2–3 times of estimated blood loss would be administered in the form of crystalloid solution, early in the treatment of haemorrhagic shock. This was adopted by Advanced Trauma Life Support and taught until 1997 [12].
Concurrently, in the latter half of the twentieth century, work looking at management of patient with burns found that successful management resuscitation of burns patients required very large volumes of crystalloid fluid [13], forming the basis of the Parkland formula which is still widely used today [14]. There was a belief that the ‘burns model’ would serve as an excellent model for trauma resuscitation as both injury patterns appeared to lead to extracellular deficits.
This reinforced the strategy of large-volume resuscitation in the context of traumatic haemorrhage, and widespread liberal fluid resuscitation strategies were used. During the Vietnam War, aggressive crystalloid administration strategies, whilst believed to have saved many lives and reduced the incidence of renal failure [15], were also thought to have been the cause of ‘Da Nang lung’, now known as acute respiratory distress syndrome (ARDS) along with other recognised syndromes associated with excess crystalloid use such as abdominal compartment syndrome [16].
Development of Permissive Hypotension
Despite this, it was not until a growing body of evidence in the 1990s and early 2000s started to challenge the dogma of large-volume crystalloid resuscitation. Three strategies began to emerge to deliver hypotensive resuscitation summarised in Table 6.1.
Injection of a fluid that will increase blood pressure has dangers in itself. If the pressure is raised before the surgeon is ready to check any bleeding that might take place, blood that is sorely needed may be lost. – W. Cannon [17]
Summary of fluid resuscitation strategies
Type of resuscitation strategy | Intervention to patient | Major clinical trials focusing on concepts |
---|---|---|
Restricted resuscitation | Limit the volume of fluid administered | Schreiber et al. [23] |
Delayed resuscitation | Restrict fluid resuscitation until admission to hospital | Bickel et al. [25] |
Hypotensive resuscitation | Titrate and control BP to less than normal range |
Restricted Resuscitation
Animal studies report that in simulated cases of uncontrolled haemorrhage, administration of large volume of crystalloids, whilst increased blood pressure, worsened bleeding at the injury site and mortality [18–20]. Two retrospective studies found that those who received a restrictive fluid regime during the resuscitation phase had better chance of survival [21, 22]. These findings should be interpreted with caution as there may be confounding factors between morality and the amount of fluid administered.
Recently, a multicentre prospective randomised pilot trial was conducted to compare outcomes of controlled resuscitation (250 ml of 0.9% saline) vs. standard resuscitation (2000 ml of 0.9% saline) in response to hypotension in trauma (<70 mmHg or absent radial pulse). They examined 192 patients and reported 24-h mortality to be higher in the standard resuscitation group (18% vs. 3%) in those who suffered from blunt trauma [23].
Delayed Resuscitation
A study looking at pre-hospital management of LTH found that the administration of fluid prior to surgical intervention did not have a significant influence on mortality rate [24]. Bickell et al.’s landmark study randomised 598 shocked patients with penetrating torso trauma into 2 groups: those who received early fluid administration (pre-hospital) and those who received delayed fluid administration (in-hospital).
The group with delayed fluid resuscitation had a better survival rate (70 vs. 62% (p = 0.04)) and suggested that it may also reduce intraoperative bleeding [25]. Within this group, in blood samples taken on arrival to hospital, there were also significantly higher haemoglobin and platelet concentrations with a shorter prothrombin, and partial thromboplastin times were longer. This may suggest that large volumes of crystalloid may not just attenuate secondary bleeding but also worsen coagulopathy.
Hypotensive Resuscitation
When targeting a lower than normal blood pressure , in animal models, both survival outcomes and control of haemorrhage improved [26, 27]. The models of haemorrhage often used in animal studies may not be entirely representative of a traumatic haemorrhage in humans. The animal models used are the most sensitive to re-bleeding (i.e. a lesion to a major artery with large volumes of crystalloid fluids given immediately) and are conducted under anaesthesia resulting in differences in vascular resistances and regional blood flow due to anaesthetic-induced vasodilatation. Despite this, collectively these studies do suggest that very aggressive and early resuscitation with clear fluids is detrimental and provides a useful starting point for clinical studies.
Clinical human studies, however, found it difficult to demonstrate benefits of hypotensive resuscitation. Dutton et al. [28] performed a prospective randomised controlled trial (RCT); 110 hypotensive trauma patients with presumed haemorrhagic shock were randomised to receive fluid resuscitation to a targeted SBP (hypotensive (70 mmHg) vs. normotensive (>100 mmHg)). There were no differences in ‘in-hospital mortality’ between these two groups; however, mean SBP in these two groups were higher than intended (100 mmHg vs. 114 mmHg).

An example of blood pressure oscillation and eventual equilibration in a patient from the low-pressure arm of the study. (From Dutton et al. [28], Fig. 3, with permission of Wolters Kluwer Health, Inc.)
Morrison et al. looked at intraoperative control of blood pressure, comparing targeting mean arterial pressure (MAP) of 50 mmHg vs. 65 mmHg. In the preliminary findings [29], they found that within the hypotensive group, early post-operative mortality had improved and that there were less to develop coagulopathies. As with Dutton’s team, trying to maintain separation of MAP proved challenging, and in fact the mean MAP values in both groups were not statistically different. This study was terminated early as it was unable to demonstrate differences in 30-day mortality with the proposed sample size [30].
What should be noted is that many of these studies had established trauma networks with short pre-hospital times (<60 min) and access to surgery was readily available. This means that any period of hypotension is short and potentially the adverse effects of shock may not have as significant of an effect. However, this is not the case in many parts of the world, even within the economically developed nations , particularly in isolated rural regions, or within a military context.
Of the three strategies for hypotensive resuscitation mentioned previously, there is no strong evidence that any can be applied universally to bleeding trauma patients, and no human data exist to guide the duration of a hypotensive strategy. To add more confusion, it is also unclear whether the restrictive resuscitation/controlled resuscitation approach might be beneficial as a consequence of less crystalloid use or due to hypotension itself. The risks associated with hypotensive resuscitation, in particular hypoperfusion and end-organ damage, have been documented in the literature, although to counter this, certain animal studies dispute the significance [31].
A recent Cochrane Review demonstrated that no large-scale RCT has shown any benefit from hypotensive resuscitation in trauma [32]. This highlights the uncertainty behind the postulated mortality benefit. Despite the lack of evidence, the concept of hypotensive resuscitation for LTH has been adopted in many national trauma guidelines and has entered widespread practice as a result [33, 34].
Defining an Appropriate Target
Analogously, the question concerning what blood pressure to aim for has also been left unanswered. When evaluating PH, one cannot avoid trying to define blood pressure targets and as such consider a suitable range, i.e. the lower and upper limits acceptable in PH. A consensus group in 2002 suggested that the presence/absence of a palpable peripheral pulse (i.e. radial pulse) could be used as this end point [35].
As with most areas pertaining to PH, the evidence for defining these limits is scarce, especially when taking into consideration non-anaesthetised patients . What does seem to be clear is that patients suffering trauma (except for those with severe traumatic brain injury) do not necessarily seem to be disadvantaged from short periods of hypotension. [36–39] There is some experimental evidence that tissue autoregulation limits may be lower than originally suspected [40].
These patients failed to respond to adequate shock treatment, although in neither case could this failure be attributed to lack of adequate transfusion therapy or to the presence of infection. The sequence of events suggests that failure of shock therapy in these cases is related to irreversible changes in the cardiovascular system resulting from prolonged tissue anoxia.
This observation would suggest that 85 mmHg might be too low to be the lower limit for a prolonged period. In the absence of any evidence, the currently accepted level of 80–90 mmHg should be the absolute lowest. Simultaneously, it could be argued that a higher goal of 100 mmHg may be more appropriate in order to ensure that 90 mmHg is never infringed.

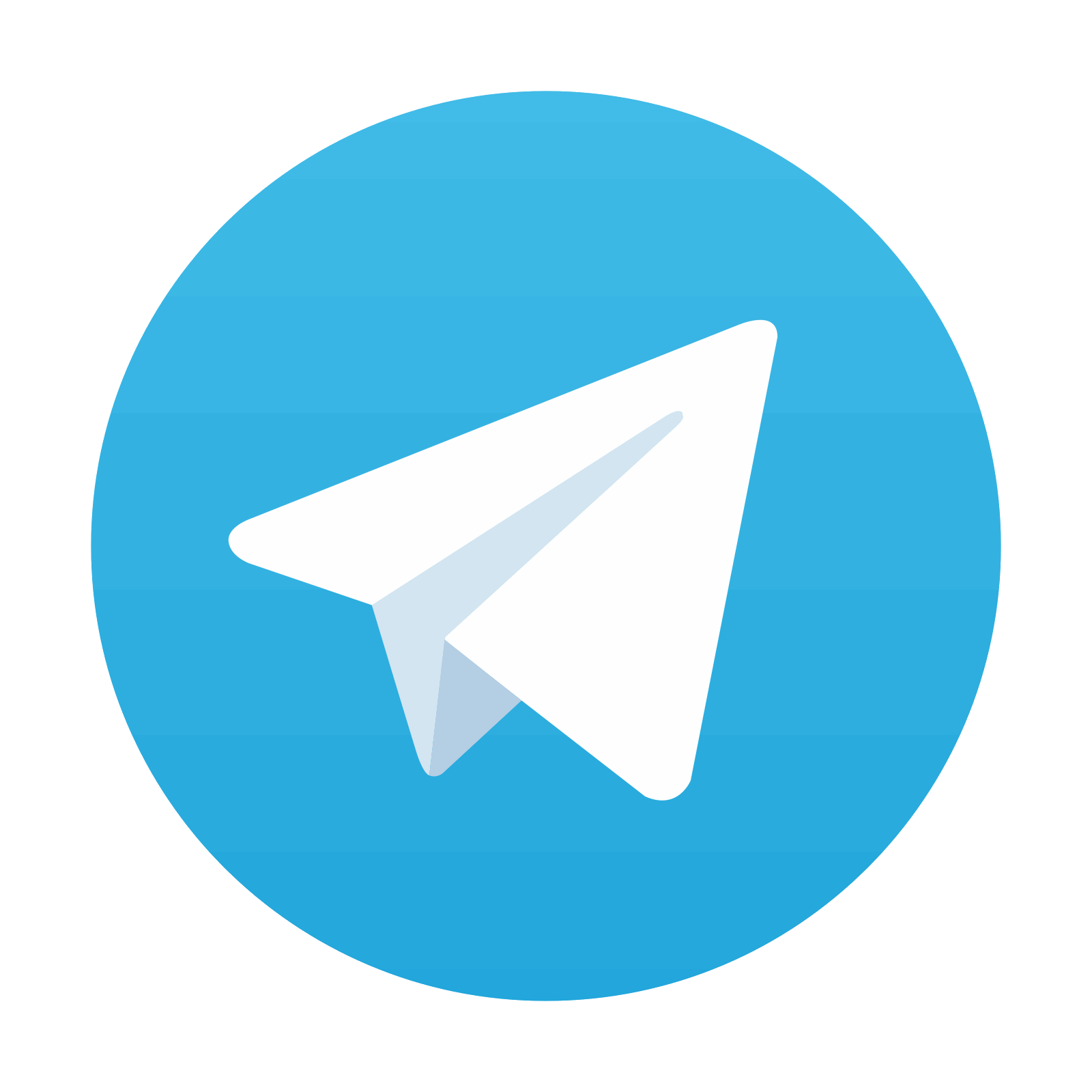
Stay updated, free articles. Join our Telegram channel

Full access? Get Clinical Tree
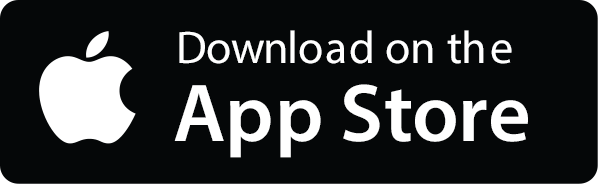
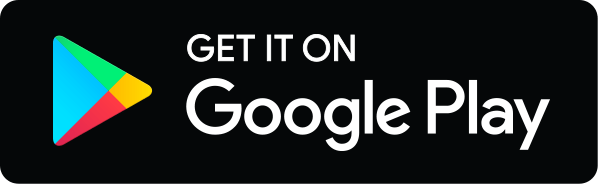