(1)
Division of Pulmonary and Critical Care Medicine, Eastern Virginia Medical School, Norfolk, VA, USA
Keywords
Hospital acquired infections (HAI)Nosocomial infectionCentral line associated blood stream infection (CLABSI)Catheter associated urinary tract infections (CAUTI)Ventilator associated pneumonia (VAP) Clostridia difficile infectionNosocomial rhinosinusitisHand washingSelective decontaminationPreventive measuresChlorhexidine“We can’t solve problems by using the same kind of thinking we used when we created them.”
Albert Einstein, Theoretical Physicist (1879–1955)
This chapter will review Central Line Associated Blood Stream Infection (CLABSI), Catheter Associated Urinary Tract Infections (CAUTI), Ventilator associated pneumonia (VAP), Clostridia difficile enterocolitis and Nosocomial Rhinosinusitis (NS) as well as measures to prevent these infections.
Hospital acquired infections (HAIs) are infections developing in the hospital setting and are a worldwide problem occurring both in developed and in developing countries. It was estimated that in 2002 a total of 1.7 million HAIs (4.5 per 100 admissions) occurred in the US and that almost 99,000 deaths resulted from or were associated with a HAIs; similar data have been reported from Europe [1–3]. These facts have been widely publicized in the lay press and attracted the attention of hospital administrators and governmental agencies, whose stated goals are to eliminate HAIs. It is however likely that these estimates are inflated. More recent data suggest that there are approximately 440,000–640,00 HAIs annually in the US [4, 5]. In a recent point prevalence study conducted in 183 acute care hospitals in 10 “geographically diverse states” in the US, 4 % of patients developed one or more HAIs [5]. In this study the most common infections were pneumonia (21.8 %), surgical site infections (21.8 %) and gastrointestinal infections (17.1 %). C. difficile was the most commonly reported pathogen, causing 12.1 % of all HAIs. Device-associated infections (CLABSI, CAUTI and VAP) which have traditionally been the major focus of infection control programs accounted for only 25.6 % of HAIs.
HIAs increase length of stay and hospital costs. The annual excess costs of HAIs in the US is estimated to be $9.8 billion [4]. The per case excess cost of a CLABSI is been estimated to be $45,814, while that for a VAP is estimated to be $40,144, a surgical site infection $20,785, C. difficile infection $11,285 while a CAUTI is estimated to cost $896 [4]. The effects of HAIs on attributable mortality are less clear. Nosocomial infections are more common in those patients with higher acuity illnesses who have a higher mortality and length of stay than less sick patients. It has therefore been unclear as to whether nosocomial infections independently contribute to adverse outcomes. Lambert and colleagues evaluated the clinical impact of HAIs on the risk of ICU death and excess length of stay [6]. Complex statistical modelling was used to answer this question. In addition, the authors evaluated the interaction of bacterial resistance with these outcome variables. This study included 119,699 patients from 537 ICU’s. During their ICU stay 7 % of patients developed hospital acquired pneumonia (HAP) and 4 % had a blood stream infection. For all pathogens the hazard ratio for excess ICU death was 2.3 for pneumonia and 3.1 for blood stream infection. The time-adjusted excess length of stay was 7.2 and 1.1°days for these diagnoses respectively. The hazard ratios for resistant organisms were only slightly larger than for non-resistant organisms. As will be reviewed below (see section. “Ventilator Associated Pneumonia”), the mean attributable mortality for VAP’s is about 13°%. The attributable mortality for the other HAIs has not been studied. It is likely that “CAUTI’s” have a negligible attributable mortality while that for surgical site infections is quite low.
In response to the widespread information generated by HAIs, governmental agencies (in the US) have introduced non-payment policies to punish healthcare providers whose patients’ develop a HAI. The position of the Federal Government, Centers for Medicare and Medicaid Services (CMS), the Agency for Health Care Quality and Improvement (AHQI), the Institute for Healthcare Improvement (IHI), and other health care organizations in the US is that HAIs should never happen (a never event) and that physicians/nurses have failed in their duty to their patient should a HAI occur. This is clearly an absurd and counter-productive approach to a very complex issue. There is no question of doubt that HAIs are associated with significant morbidity and an increase in the length and cost of hospitalization and all reasonable efforts should be taken to avoid such infections. However, the reality of critical care medicine is that HAIs are an inevitable consequence of managing very sick patients in the ICU. This does not mean that ICU managers/directors should not track HAIs. This data is vital to identify trends and to determine the microbiology of the infecting pathogens. In the study by Climo et al., despite strict adherence to comprehensive infection control measures, 4.78 hospital acquired blood stream infections occurred per 1,000 patient days [7]. It is therefore critically important to recognize that while evidence based interventions reduce the risk of HAIs they cannot be totally eliminated and therefore healthcare workers should not be punished for factors beyond their control. By the very nature of their illness, host defense mechanisms are impaired (cough reflex, muco-ciliary clearance, skin and mucosal barrier, innate and acquired immunity) which predispose the critically ill and injured ICU patient to infection. The only way to “completely eliminate” HAIs, is by not recording them accurately (i.e. to cheat) or by admitting low acuity (healthy) patients to the ICU. Nevertheless, in October 2008, CMS discontinued additional payments for HAIs (CLABSI, CAUTI and mediastinitis following CABG) that were deemed preventable. These punitive measures are likely to have a number of adverse effects (unintended consequences), including “If you don’t look, you don’t find and don’t report” (and? don’t treat). Furthermore surveillance bias and data quality bias influence reporting results [8]. Illustrated in Fig. 16.1 is the rate of CLABSI before and after public reporting was mandated in the State of Pennsylvania in 2007 [9]. Common sense would suggest that both rates are incredibly low and not believable… if it looks good to be true… it’s probably not true. Using Medicare impatient claims following 638,761 CABG procedures in 1,234 hospitals in the US, Calderwood and colleagues demonstrated that at the exact time that CMS adopted the withholding of payments for mediastinitis there was a sudden and precipitous fall in the coding (reporting) of mediastinitis [10]. However, mediastinitis rates using National Healthcare Safety Network surveillance data showed no change in mediastinitis infection rates, i.e. if it looks like a duck and quacks like a duck it must be coded as a camel.
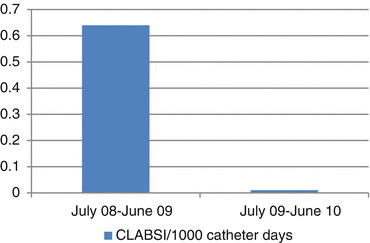
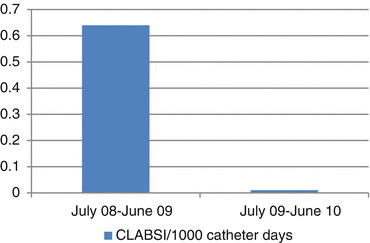
Fig. 16.1
CLABSI before and after mandatory reporting in the state of Pennsylvania
By the very nature of “the beast” critically ill patients will develop HAI’s, and the sicker the patient the greater the likelihood they will develop an infection.
Colonization with Multidrug Resistant Organisms
The sequence of events leading to HAIs has been well characterized. The vast majority of HAIs are due to bacteria and fungi (Candida spp.) which the patients’ acquire while in the hospital and ICU. Colonization of the patient’ skin and upper respiratory tract is the first step in the chain of events leading to a HAI. For example, HAP including ventilator associated pneumonia (VAP) results from the aspiration of colonized oropharyngeal material. It is now well recognized that the most important mode of transmission of nosocomial pathogens are through direct-contact between an infected or colonized person and a susceptible host [11]. This occurs predominantly by means of transmission through the hands of health care providers; i.e. health care providers transfer potential pathogens from infected or colonized patients to other patients. Less commonly, infection occurs by contaminated medical devices, from the patient’s own flora and via airborne particles. In addition, contaminated hard surfaces (bed-rails, bedside tables) and soft surfaces (sheets, pillowcases or gowns) may be a source of microbes contributing to indirect-contact and aerosol transmission of nosocomial-related pathogens [12, 13].
The colonizing pathogens are usually multidrug resistant organisms (MDR) [6, 14]. The most common nosocomial pathogens are Clostridium difficile, Staphylococci (especially Staphylococcus aureus), Klebsiella species, Escherichia coli, Enterobacter species, Pseudomonas aeruginosa, Streptococcus species and Acinetobacter species [5]. The prevalence rates of pathogens that cause HAIs have a high level of resistance to antibiotics such as MDR Pseudomonas aeruginosa, extended-spectrum beta-lactamase (ESBL) producing Enterobacteriaceae, MDR Acinetobacter baumannii, Carbapenem Resistant Enterobacteriaceae (CRE), Methicillin Resistant Staphylococcus Aureus (MRSA), and vancomycin resistant enterococci (VRE).
Handwashing and Infection Control Measures
Handwashing
Measures which reduce the colonization rate of hospitalized patients with potentially pathogenic organisms reduce the risk of HAIs. Colonization of ICU patients occurs predominantly by means of indirect patient-to-patient transmission through the hands of health care providers. Common sense would therefore suggest that the most effective means to break this cycle is a rigorous program and enforcement of adequate hand hygiene. This seminal observation was made by Dr. Ignac Semmelweis in 1847 when he demonstrated that puerperal fever could be prevented by hand antisepsis (with a chlorine lime solution) [15]. While no randomized controlled trials comparing hand-antisepsis to no hand-antisepsis have been (or are likely to be) conducted, multiple lines of evidence suggest that hand-antisepsis is the single most important intervention to reduce HAIs [16, 17].
ICU managers must enforce strict policies of hand antisepsis of all health care workers on both entry and exit from the ICU room [16]. Alcohol-based hand-hygiene products have excellent in vitro germicidal activity against gram-positive and gram-negative bacteria including multi-drug resistant pathogens [16]. Alcohol-based gels or foam products should therefore be provided outside every ICU room. As hand antisepsis products have very poor activity against bacterial spores, the use of gloves and hand washing with soap-and water should be mandated in all patients with Clostridia difficile infection [16].
Chlorhexidine Bathing
Climo and colleagues demonstrated that daily bathing with chlorhexidine-impregnated washcloths reduces the risk of acquisition of MDR organisms and the development of hospital acquired blood stream infections [7]. The use of chlorhexidine-impregnated washcloths did not alter chlorhexidine susceptibly of clinical isolates collected during the study. This approach is therefore strongly recommended to reduce colonization of ICU patients.
Gloves and Gowns and Healthcare Provider Apparel
The use of “gloves and gowns” has been popularized as a method of reducing the transmission of potential pathogens from the clothes of health care workers. It has however remained rather speculative and unproven that the clothes of health care workers may play a role in the transmission of such organisms. Harris and colleagues demonstrated no benefit from universal “gown and glove” use when compared to usual care [18]. This findings is supported by the study of Huskins et al. who provided convincing evidence that in the setting of a MRSA/VRE screening protocol, barrier precautions are no more effective in preventing the transmission of potential pathogens than “adequate” hand hygiene alone [19].
The Society of Healthcare Epidemiology (SHEA) of America has recently released an official statement regarding heath care workers attire and infection control measures [20]. This policy statement emphasized that “no clinical studies have demonstrated cross–transmission of healthcare–associated pathogens from a health care provider to a patient via apparel”. They further state that “priority should be placed on evidence–based measures to prevent HAIs (e.g., hand hygiene, appropriate device insertion and care, isolation of patients with communicable diseases, environmental disinfection).” Studies which have assessed patient’s preferences for certain types of attire, indicate a strong preference for formal attire, including a white coat. Formal attire and white coats inspire confidence in the physician and is associated with an increased degree of professionalism [20–22]. A “Bare below the elbows” (BBE) mandate has been issued in the UK. BBE is defined as healthcare providers wearing short sleeves, no wristwatch, no jewelry, and no ties during clinical practice. Patients have shown general disapproval of BBE attire [22]. Furthermore, there is no evidence that BBE has any impact on the transmission of pathogens and the acquisition of HAI [23]. SHEA does not recommend limiting the use of specific HCP apparel including neckties and jewelry [20].
Universal Screening for MDR’s and “Protective Isolation”
Screening all ICU admissions for nasal MRSA carriage and isolating those colonized or previously colonized/infected with MRSA has been widely adopted as a method to reduce spread of MRSA. Previous studies have demonstrated that this “vertical” approach has limited or no benefit. More recent prospective, cluster controlled studies have confirmed this finding. Derde et al. performed a large multi-phase, time series, cluster randomized controlled trial comparing usual infection control measures with universal chlorhexidine body-washing combined with enforced hand hygiene (best standard precautions) [17]. They then compared best standard precautions with conventional screening for MRSA and VRE, and with rapid screening (PCR testing for MRSA) with contact precautions for all identified carriers [17]. In this study, improved hand hygiene plus unit-wide chlorhexidine body-washing reduced acquisition of MDR’s. In the context of a sustained high level of compliance to hand hygiene and chlorhexidine bathing, screening and isolation of carriers did not reduce acquisition rates of MDR, whether or not screening was done with rapid testing or conventional testing. In a similar study, Huang et al. compared (1) screening and protective isolation, with (2) screening, protective isolation and decolonization of MRSA with (3) a strategy of universal decolonization using daily chlorhexidine bathing and intranasal mupirocin for 5 days (without screening and isolation) in a very large cohort of patients (76,256 patients and 74 ICU’s) [24]. The strategy of universal decolonization was significantly more effective in preventing infection with MRSA as well as bloodstream infection from any pathogen. These studies in composite now suggest that the screening and isolating patients with MRSA is an ineffective intervention in preventing HAI and should abandoned. Not only is this strategy ineffective but it has many downstream negative consequences. Apart from the costs and time expended, this strategy “isolates” patients from both their health care providers and their family. In the study by Harris et al. universal use of “gowns and gloves” significantly reduced the number of times heath care provides entered the room of their patients. Previous research has demonstrated that patients isolated for infection control precautions experience more preventable adverse events, express greater dissatisfaction with their treatment, and have less documented care [25–27]. Families of patients in “protective isolation” find the procedures involved burdensome and distressing and interfere with their ability to provide emotional support to their loved ones. Protective isolation should however be reserved for patients with C. difficile infection
Oropharyngeal and Gastrointestinal Decolonization
Interventions such as chlorhexidine mouth wash and selective decontamination of the digestive tract with non-absorbable antibiotics kill colonizing pathogens before they can be aspirated and thereby theoretically reduce the risk of VAP [28, 29]. The use of chlorhexidine mouth washes is however controversial, as this intervention has only been demonstrated to reduce this risk of VAP in cardiac surgery patients. A recent metaanalysis demonstrated that chlorhexidine mouth washes had no effect the risk of VAP, duration of mechanical ventilation or ICU LOS in non-cardiac surgery patients and was associated with a trend towards increased mortality in these patients (see Sect. “Ventilator Associated Pneumonia”) [30]. Nevertheless, meticulous oral hygiene is recommended in all intubated ICU patients.
Selective decolonization of the digestive tract (SDD) was popularized in Europe over 30 years ago [31]. SDD regimens typically include oropharyngeal and enteral antimicrobials (usually tobramycin, polymyxin E, and amphotericin B) throughout the period of endotracheal intubation in combination with parenteral antimicrobials (usually cefotaxime) for the first few days. Various modifications of the “full SDD” protocol have been studied including selective digestive decontamination (without parenteral antibiotics) and selective oropharyngeal decontamination. The goal of SDD is to prevent patient colonization with potentially pathogenic organisms. Since the original study by Soutenbeek et al. in 1984 [31], over 50 RCT’s have been performed in medical-surgical, medical, surgical, pediatric and burn ICU’s. In general these studies have shown a reduction in HAIs particularly VAP as well as a reduction in the duration of mechanical ventilation and duration of ICU stay [32–34]. The effect of SDD on mortality has been somewhat inconsistent [33–35]. However, a meta-analysis which included 21 “full SDD” studies demonstrated a significant reduction in mortality (OR 0.71; 95 % CI 0.61–0.82, p < 0.001) [36]. While SDD is “popular” in Europe this technique has not been adopted in the US for fear of increasing bacterial resistance. However, this fear appears unfounded. In a review of 64 studies, Daneman and colleagues detected no relation between the use of SDD and the development of antimicrobial resistance, and concluded that the “perceived risk of long–term harm related to selective decontamination cannot be justified by available data” [37]. Oostdijk et al. performed a cost effective analysis of the use of SDD/SOD in 13 Dutch ICU’s [38]. In this analysis SDD/SOD were associated with relative risk reductions of mortality at day 28 of 13 % and 11 %, respectively, as compared with standard care with total patient costs being lower with SDD/SOD.
Private Rooms and Environmental Control
Teltsch and colleagues demonstrated a reduction in colonization rates following ICU room privatization (single patient rooms) [39]. This finding is likely explained by the fact that it is difficult to operationalize and enforce hand hygiene when patients are in close proximity in a multi-bed ICU room.
ICU rooms should be cleaned (terminal cleaning) once a patient has been discharged from the room and prior to the admission of the next patient; this is particularly important in patients with a C. difficile infection. A novel approach to environmental control of MDR organisms involves the use of copper impregnated hard and soft surfaces within the ICU. Metallic copper has intrinsic broad-spectrum antimicrobial activity. In vitro, copper surfaces reduce bacterial concentration by at least seven logs within 2 h, including bacteria commonly encountered in the ICU. Schmidt et al. demonstrated that copper limits the concentration of bacteria on bed rails within the ICU [40]. Salgado et al. demonstrated that patients cared for in ICU rooms with copper alloy surfaces had a significantly lower rate of incident HAIs and colonization with MRSA or VRE than did patients treated in standard rooms [41].
An aggressive policy of hand washing together with chlorhexidine body bathing appears to be the most effective method of limiting colonization of ICU patients with MDR organisms and thereby reducing the risk of HAI’s. Although the data supporting SDD appears compelling, the use of this technique remains controversial.
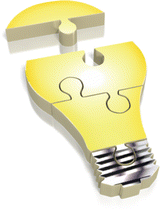
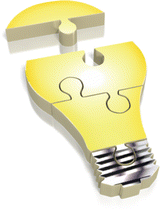
Central Line Associated Blood Stream Infection
Central venous catheters (CVC’s) are ubiquitous in the ICU. In the US alone 15 million CVC days occur in ICU’s each year [42]. CVC’s are not without risk during both placement and while in situ. The most important complication associated with use of CVC’s are central line associated blood stream infections (CLABSIs). Approximately 250,000 cases of CLABSI (80,000 in the ICU) occur in hospitals in the US annually; an estimated 30,000–62,000 of these patients die as a result of this infection [42, 43]. In response to this problem, a number of healthcare organizations in the US and Europe have published “Clinical Practice Guidelines” with the aim of reducing the incidence of CLABSIs [43–49]. In common, these guidelines recommend “bundles of care” which include selection of catheter type, method of placement, care of the catheter and timely removal of the catheter. With the institution of these “bundles” the incidence of CLABSI has been reduced to less than 3 per 1,000 catheter-days in most hospitals in the US and Europe [50]. Current data demonstrates a mean CLABSI rate of between 1.1 and 1.8/1,000 catheter days in non-burn ICU’s in the US [51].
Central Line Associated Blood Stream Infection (CLABSI) may be caused by:
non-tunneled central venous catheters (CVC’s)
tunneled CVC’s
peripherally inserted central venous catheters (PICC)
arterial lines
non-tunneled hemodialysis catheters
tunneled hemodialysis catheters
subcutaneous ports
Generally tunneled/cuffed CVCs and PICC catheters (out-patients) have a lower risk of CLABSI than non-tunneled CVCs. Tunneled hemodialysis catheters have a lower risk of CLABSI than non-tunneled hemodialysis catheters. Similarly, the risk of CLABSI is lower for arterial lines as compared to non-cuffed CVCs. However, it should be noted that the simultaneous placement of multiple CVC’s significantly increases the rate of CLABSI [52]. The incidence of CVC related blood stream infection is reported to vary from about 1 to 5 per 1,000 catheter-days (mean about 2/1,000 catheter days); for arterial lines the incidence varies from about 1 to 3 per 1,000 catheter-days [51, 53–55]. While the risk of CLABSI with PICC lines is generally believed to be less than those of CVC’s, this may not be so in hospitalized patients. A prospective cohort study by Safdar and Maki reported that ICU patients with PICCs had rates of CLABSI that were similar to those in patients with non-tunneled central catheters and higher than infection rates for PICCs in the outpatient setting [56]. Pongruangporn et al. reported a PICC CLABSI rate of 3.13/1,000 catheter days in hospitalized patients [57]. In this study the risk of infection was greater for double lumen (OR 1.89) and triple lumen PICC’s (OR 2.87). Similarly, Baxi et al. reported a PICC CLABSI rate of 2.69/1,000 catheter days [58]. In this study double and triple lumen PICCs as well as “Power PICCS” had an increased risk of CLABSI. It should be noted that PICCs are associated with an increased risk of thrombosis and pulmonary emboli. In a single-center study of hospitalized patients, the incidence of PICC thrombosis was 4.9 % with those patients with a history of prior venous thromboembolism having an extremely high risk for this complication (OR 10.83). The size and shape of the tip of the PICC influences the risk of thrombosis; Power PICCs appear to be associated with an increased risk of thrombosis [58].
The pathogenesis of non-tunneled CVC infections is usually due to extraluminal colonization of the catheter by skin commensal flora with hub colonization playing a lesser role. In comparison, tunneled CVC’s or implantable devices, contamination of the catheter hub and intraluminal infection is the most common route of infection [59]. A number of factors have been identified as increasing the risk of CLABSI including, length of time in situ, the number of lumens in the CVC, the number of stopcocks, the transfusion of blood and blood products, parenteral nutrition, and an open infusion system. While the risk of CLABSI increases with time the catheter remains in-situ, changing catheters at regularly scheduled interval has not been shown to reduce the risk of CLABSI. Furthermore, guidewire exchanges may increase the risk of infection [60].
The preferred site for placement of a non-tunneled CVC is controversial. The subclavian site is associated with a lower rate of cutaneous colonization, dressing disruption, catheter colonization and infection and is recommended in US and European guidelines [61]. While the subclavian site is the preferred site for CVC placement in most patients, this is not always possible. Many guidelines recommend that the femoral site be avoided due to the perceived higher risk of CLABSI associated with this site. The 2011 recommendations from the “Healthcare Infection Control Practices Advisory Committee” of the Centers for Disease Control (CDC) state “Avoid using the femoral vein for central access in adult patients” (Class 1A recommendation) [43]. A meta-analysis by Marik et al. demonstrated no difference in the risk of CLABSI between the subclavian, internal jugular or femoral sites [62]. This study was limited by the fact that the quality of the studies included in the meta-analysis were generally poor, however the authors found no evidence to support the CDC recommendations [62]. More recently, Timset and colleagues analyzed the risk of colonization and CLABSI in 2,128 patients who had either a femoral (n = 1,301) or internal jugular (n=1,001) CVC placed as part of two RCT’s [63]. As the data were obtained from prospective RCT’s specifically studying CLABSI they were able to perform a very detailed analysis from the combined data set. Despite the fact that patients with femoral catheters were sicker than those with an internal jugular CVC’s (higher SAPS II score) the risk of CLABSI, catheter-tip colonization, dressing disruption and skin colonization did not differ between the two sites. This very well executed study therefore supports the meta-analysis by Marik et al. Although the meta-analysis by Marik et al. did not show a difference between the internal jugular and subclavian sites, “soft data” suggests that the risk of infection is lowest at the subclavian site [64–67]. In the study by Parienti et al., patients with a BMI > 28.4 kg/m2 had a higher risk of CLABSI with femoral as compared to jugular placement of a dialysis catheter [68]. Parienti and colleagues have recently completed the 3–SITES Multicenter Randomized Controlled Trial in which over 3,000 patients were randomized to placement of a CVC at either the internal jugular, femoral or subclavian site (clinTrials.gov NCT01479153). It should be noted that in this study patients with morbid obesity underwent restrictive randomization to the subclavian or internal jugular sites. The results of this study should (hopefully) provide high quality evidence to resolve this ongoing debate.
Based on these data, a pragmatic approach to site selection for central venous cannulation is recommended. The site of preference should depend on the expertise and skill of the operator and the risks associated with placement. In an emergent situation or in high risk patients femoral placement may be preferred. All lines that are placed under less than ideal emergency circumstances should be removed and re-sited within 24–48 h. Lines placed in the internal jugular and femoral site should be placed under direct ultrasound guidance as this technique reduces the risk of placement complications [69–72]. The subclavian site should be avoided in patients with chronic renal failure to preserve the arm veins and subclavian vein for possible fistula placement [48]. The femoral veins should be avoided in renal transplant patients. The femoral site should be avoided in morbidly obese patients (BMI > 40 kg/m2). Another potential reason to avoid the femoral site is to allow early mobilization; this is particularly true for dialysis catheters. The internal jugular site is associated with a higher risk of CLABSI in patients with tracheotomy and this site should therefore be avoided in these patients [73, 74].
Coagulase-negative staphylococci (CNS) account for up to 40 % of cases. Methicillin resistant S. aureus (MRSA) and vancomycin resistant Enterococci are becoming important causes of CLABSI [75]. The implicated pathogens vary somewhat with the site of catheter placement, with the incidence of Candida species and gram-negatives being higher for femoral catheters [76, 77].
The diagnosis of CLABSI can be challenging. Routine culture of blood withdrawn from the catheter is not recommended, however, the catheter exit site should be inspected daily for evidence of erythema or pus. The absence of local infection, however, does not exclude CLABSI. In a patient with an indwelling CVC who develops a fever two sets of peripheral blood cultures should be drawn. If the patient has systemic signs of infection and no other identifiable source of infection, the catheter should be removed and empiric antibiotics commenced, pending culture results (see Chap. 18; Fever in the ICU). In patients with limited venous access the central catheter may be replaced with a new catheter over a guidewire, however, both the catheter tip as well as the intracutaneous portion of the catheter should be sent for culture. If the catheter culture returns positive (>15 cfu) or the blood cultures are consistent with a CLABSI, the line that was changed over a guidewire must be removed and replaced with a new catheter at a clean site. Followup blood cultures should be obtained in patients with CLABSI. If blood cultures remain positive a thorough investigation for septic thrombosis, infective endocarditis, and other metastatic infections should be pursued.
Staphylococcus epidermidis is the most commonly isolated CNS. Currently, there are more than 40 recognized species of CNS. These organisms typically reside on healthy human skin and mucus membranes, rarely cause disease, and are most frequently encountered by clinicians as contaminants of microbiological cultures. Other important CNS include Staphylococcus lugdunensis, Staphylococcus saprophyticus, and Staphylococcus haemolyticus. The pathogenic potential of CNS lies in their ability to colonize and proliferate on biomaterials. As CNS account for the majority of CLABSI it is often difficult to distinguish a true CLABSI from a “contaminant.” A single positive culture should be regarded as a contaminant while multiple positive cultures suggest a CLABSI. When in doubt cultures should be repeated. The Infectious Diseases Society of America consider the following criteria to indicate probable or definite CNS CLABSI [78]:
At least two positive blood cultures
At least one blood culture should yield a quantitative count of ≥ 15 colony-forming units (CFU)/m
The blood samples that yielded positive culture results should have been collected within a 72-h period.
This issue is further complicated by the fact that the biotype cultured from the blood may not be the same biotype as cultured from the catheter [79]. Furthermore, it would appear that CNS strains that cause CLABSI may be genetically distinct from common skin colonizers [80]. It is therefore likely that genetic biotyping may assist in distinguishing true “pathogens” from colonizers. Procalcitonin (PCT) assays may be useful in distinguishing true infection from contamination. Scheutz and colleagues demonstrated that patients with CNS blood stream infection had higher PCT concentration than those with blood contamination [81]. At a cutoff of 0.1 ng/dL, PCT had a sensitivity of 100 %, and a specificity of 80 % for the diagnosis of BSI.
In a number of patients the fever is noted to defervesce after removal of the catheter, yet the blood cultures remain negative. These patients are considered to have “culture-negative” CLABSI; presumably the bacteremia was intermittent or below the threshold required for a positive culture. Antibiotics are generally not required in these cases.
The usual approach to patients’ with suspected CLABSI involves removal of the catheter. However, only about 20 % of patients who have a CVC removed due to suspected CLABSI are proven to have CLABSI. This subjects patients with negative cultures to the added risk of line placement. To avoid this problem a number of methods have been investigated for the diagnosis of CLABSI which do not require removal of the CVC [82]. Comparison of blood cultured from the CVC with that from a peripheral venous site is currently the most widely used technique. In patients’ with CLABSI time to positivity is shorter (more than 2 h) for blood withdrawn from the catheter as opposed to the peripheral site. However, Kaasch et al. demonstrated that the differential time to positivity was not useful in diagnosing CRBSI in routinely obtained blood cultures [83]. This study raises doubt about the utility of this technique.
Management of CLABSI’s
In patients with suspected CLABSI all indwelling catheters should be removed and empiric antibiotic therapy commenced. Vancomycin is usually recommended because of its activity against coagulase negative staphylococci and S. Aureus. Additional empiric coverage for enteric gram-negative bacilli and Pseudomonas aeruginosa with the use of a third or fourth-generation cephalosporin, such as ceftazidime or cefepime, may be needed for severely ill or immunocompromised patients.
Patients with CLABSI should be classified into those with uncomplicated bacteremia or those with complicated infections, in which there is:
Septic thrombosis
Endocarditis
Osteomyelitis
Possible metastatic seeding
If there is a prompt response to initial antibiotic therapy, most patients who are not immunocompromised without valvular heart disease or an intravascular prosthetic device should receive 10–14 days of antimicrobial therapy. A more prolonged course of therapy (4–6 weeks) should be considered if there is persistent bacteremia or fungemia after catheter removal or if there is evidence of endocarditis or septic thrombosis, and 6–8 weeks of therapy should be considered for the treatment of osteomyelitis. Echocardiography including transesophageal ECHO should be considered in patients with persistent bacteremia/fungemia or lack of clinical improvement to exclude endocarditis.
CNS are the most common cause of CLABSI. CLABSI due to coagulase-negative staphylococci usually present with fever alone, rarely developing “frank sepsis” [84]. While coagulase-negative staphylococci CLABSI may resolve with removal of the catheter and no antibiotic therapy, most experts believe that such infections should be treated with antibiotics (vancomycin) [84]. Furthermore, it has been suggested that catheter removal is not required with CNS CLABSI and that treatment with antibiotics alone is adequate. This approach has been endorsed by international guidelines [78]. However, Raad and colleagues demonstrated that while this approach was effect in the resolution of infection within 48 h of commencing antimicrobial therapy, it was associated with an unacceptably high rate of recurrence [85]. This is probably related to the fact that CNS develop a multilayered biofilm matrix on the catheter in which they embed themselves and that eradication of catheter colonization is difficult without removal of the catheter.
Antibiotics Lock Therapy
In patients with CLABSI removal of all indwelling vascular catheters is recommended. This may be problematic in patients with tunneled catheters and infusion ports; this necessitates removal of the device and re-insertion once the infection has cleared. A potential solution to this problem is based on the fact that the majority of infections in tunneled catheters originates in the catheter hub and then spreads to the catheter lumen. This fact has prompted the “antibiotic-lock” technique, where the catheter lumen is filled with pharmacologic concentrations of antibiotics where they are allowed to dwell for hours or days. Antibiotic lock therapy, with concomitant parental therapy has a reported response with a catheter salvage rate between 60 and 82 % [84, 86, 87].
Prevention of CLABSI
The best way to avoid a CLABSI is to avoid placement of a central line. While entrenched dogma suggests that vasopressor agents should never be administered using a peripheral venous catheter, this appears to be incorrect (see Chap. 12) [88]. The administration of vasoactive agents using a peripheral catheter in selected patients should reduce the need for central venous catheterization.
While CLABSI is an inevitable complication of critically ill patients who require a central venous catheter, the risks can be significantly reduced by specific interventions [49, 89]. When inserting a new catheter the following precautions should be followed:
Full drapes and strict sterile precautions
Use of a line cart
Skin cleansing with chlorhexidine
ICU nurse for observation (sterility not broken) and assistance
Additional measure include:
Clear adhesive dressings
Limit blood draws from CVC’s
Limits “breaks” in the circuit
Remove the catheter when no longer required
All catheters inserted in clinically urgent situations without maximal sterile barrier precautions should be replaced as soon as possible
Before accessing catheter hubs or injection ports, clean them with an alcoholic chlorhexidine preparation or 70 % alcohol to reduce contamination
Replace administration sets not used for blood, blood products, or lipids at intervals not longer than 96 h
Povidone-iodine or polysporin ointment should be applied to hemodialysis catheter insertion sites in patients with a history of recurrent Staphylococcus aureus CLABSI
Guidewire exchanges are strongly discouraged [60]
Antimicrobial coated/impregnated CVC’s have been demonstrated to reduce the risk of colonization and CLABSI [90, 91]. These catheters should be considered in ICU’s when
the incidence of CLABSI > 3/1,000 catheter/days despite all the precautions listed above
Patients who have limited venous access and a history of recurrent CLABSI.
Patients who are at heightened risk for severe sequelae from a CLABSI (e.g., patients with recently implanted intravascular devices, such as a prosthetic heart valve or aortic graft).
The use of strict aseptic techniques, chlorhexidine skin cleansing and anti-microbial impregnated catheters reduce CLABSI by reducing extraluminal colonization of the catheter. These measures however do not address CLABSI caused by hub colonization. Wright et al. demonstrated that the use of disinfection caps with 70 % alcohol reduced the rate of CLABSI in patients with central lines [92]. The disinfection cap is a plastic threaded cap that houses a small sponge saturated with 70 % isopropyl alcohol. The disinfection cap is threaded onto any lumen not actively in use and remains in place until the lumen is accessed, at which time the disinfection cap is removed and discarded. After the lumen is accessed, a new disinfection cap is threaded on to the lumen. In patients receiving hemodialysis through a catheter, routine interdialytic use of an antimicrobial lock solution has been demonstrated to reduce the risk of CLABSI and is strongly recommended especially in high risk patients [93, 94]. Timset and colleagues demonstrated that disruption of catheter dressings is a common problem and an independent risk factor for CLABSI [67]. In this study dressing disruption occurred less frequently with subclavian catheters, which may largely explain the lower risk of CLABSI that has been reported at this site [64–66].
Do not routinely replace CVCs or arterial catheters. As the risk of infection is less with tunneled dialysis catheters, a tunneled catheter should be placed as soon as feasible (patients with ARF generally will require 2–6 weeks of renal replacement therapy). The role of PICCs in the ICU is unclear. PICCs should be considered in patients who will require prolonged venous access.
Catheter Associated Urinary Tract Infection
It is widely quoted that catheter related urinary tract infections (CAUTI) are the most common HAIs in the ICU, accounting for approximately 23 % of HAI infections among adult ICU patients in the US [95]. It is however likely that this data is wrong and that most ICU patients who are treated for CAUTI have “asymptomatic” bacteriuria which does not require treatment. The treatment of patients with “asymptomatic bacteriuria” is based on a single study performed in the early 1980s that may not be applicable today [96]. Platt and colleagues demonstrated that in hospitalized patients bacteriuria with greater than >105 colony forming units (CFU) of bacteria per milliliter of urine during bladder catheterization was associated with a 2.8-fold increase in mortality. Based on this study hundreds of thousands (if not millions) of hospitalized patients with urinary tract colonization have been treated with antibiotics. In patients with indwelling urinary catheters, colonic flora rapidly colonizes the urinary tract. Stark and Maki have demonstrated that in catheterized patients bacteria rapidly proliferate in the urinary system to exceed 105 cfu/mL over a short period of time [97]. Bacteriuria develops at an average daily rate of 3–10 % per day of catheterization. Almost 26 % of patients with a catheter in place for 2–10 days develop bacteriuria while virtually all patients who have been catheterized for 1 month develop bacteriuria.
The terms bacteriuria and UTI are generally although incorrectly used as synonyms. Indeed, most studies in ICU patients (at least prior to 2009) have used bacteriuria to diagnose a UTI. Bacteriuria implies colonization of the urinary tract without bacterial invasion and an acute inflammatory response. Urinary tract infection implies an infection of the urinary tract. However, criteria have not been developed for differentiating asymptomatic colonization of the urinary tract from symptomatic infection. Furthermore, the presence of white cells in the urine is not useful for differentiating colonization from infection, as most catheter-associated bacteriurias have accompanying pyuria [98].
According to National Healthcare Safety Network (NHSN) data from 2006 to 2007, rates CAUTI ranged from 2.6 to 6.5/1,000 catheter days in non-burn ICU’s with an average rate of about 2.9/1,000 catheter days [99]. Rates on general care wards were higher, ranging from 4.7/1,000 catheter days in adult step-down units to 16.8/1,000 catheter days in rehabilitation units. In January 2009, the NHSN and the CDC made a significant change to the definition of CAUTI: asymptomatic bacteriuria was removed, leaving only symptomatic and bacteremic cases of CAUTI to be counted [51]. This change likely affected the reported incidence of CAUTI. According to NHSN data for 2009, CAUTI rates ranged from 1.2 to 2.3/1,000 catheter days in non-burn ICUs [51]. Similarly, Press and Metlay reported that the incidence of CAUTI at their institution fell from 12.5 ± 0.4/1,000 catheter days to 5.3 ± 0.5 following the change in definition [100].
The distinction between asymptomatic bacteruria and “symptomatic CAUTI” is very important, as asymptomatic catheter associated bacteriuria or candiduria is rarely associated with adverse outcomes and generally does not require treatment with antimicrobials. The diagnosis of “symptomatic CAUTI” is however exceedingly problematic (if not impossible) in ICU patients. The diagnosis of a symptomatic CAUTI requires one or more symptom of a UTI and a positive urine culture growing >105 cfu/mL with no more than two microorganisms. Symptoms associated with a UTI include urinary frequency, urgency, dysuria, and suprapubic tenderness, symptoms that are unlikely to be volunteered by any ICU patient. Indeed, if an ICU patient is able to volunteer these symptoms he/she does not need a Foley catheter and is unlikely to require continued treatment in an ICU. Furthermore, while fever is common in the ICU, a CAUTI is rarely the cause (see Chap. 18). Tambyah and Maki assessed 1,497 newly catheterized hospitalized patients for evidence of “CAUTI” [101]. In this study, 235 new cases of CAUTI were diagnosed. There was no difference in the signs and symptoms between the patients with and without CAUTI. Only 1 of the 235 (0.42 %) episodes of CAUTI that were prospectively studied was equivocally associated with secondary bloodstream infection. i.e. less than 0.01 % of catheterized patients are likely to develop a significant infection (which is easily treated with antibiotics). Similarly, in a study of 4,465 patients who were admitted to the ICU for a least 48 h, 6.5 % developed a UTI (most were likely asymptomatic bacteruria) with an overall incidence of 9.6 per 1,000 ICU days [102]. However, the incidence of bacteremia and fungemia was only 0.1 per 1,000 catheter days.
Foley catheters are essential in critically ill ICU patients to monitor volume status and renal function and in sedated and comatose patients who have lost voluntary control over micturition. The benefit of these devices clearly outweighs the very low risk of a true CAUTI (approx. 1 in 1,500 patients), which it itself is easily treated with antibiotics and very rarely associated with adverse outcomes. Once the ICU patient has recovered the Foley should be removed. This data suggests that with very few exceptions (patients with urological surgery, stones, stents, renal transplant, etc.,) the urine of ICU patients should NEVER BE cultured… as thousands of patients without an infection (asymptomatic bacteruria) will be treated needlessly with antibiotics (akin to giving antibiotics to sterilize the gut). Catheterized patients with a fever and systemic signs of sepsis should have blood cultures performed and treated with broad-spectrum antibiotics pending culture data (see Chap. 18). Avoiding treatment of asymptomatic bacteriuria should reduce the risk of development of antibiotic resistance and is consistent with the Infectious Diseases Society of America and US Preventive Services Task Force guidelines on bacteriuria [103].
While true CAUTI’s are uncommon in ICU patients, Foley catheters should only be placed by adequately trained healthcare professionals using sterile precautions. The Foley should be connected to a closed system and removed when no longer required. Despite the fact that anti-infective urinary catheters seem to reduce bacteriuria in patients with short-term urinary catheterization, there is no convincing evidence that use of these catheters prevents CAUTI, UTI-related bloodstream infection, or mortality.
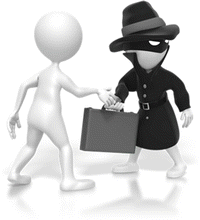
Ventilator Associated Pneumonia
Ventilator-associated pneumonia (VAP) is defined as a pneumonia which develops after 48 h of mechanical ventilation. VAP is believed to be the commonest nosocomial infection in the ICU and an important cause of morbidity. While the incidence varies according to the diagnostic criteria used and the patient population, it complicates the hospital course of about 20 % of patients receiving mechanical ventilation or about 5 episodes per 1,000 ventilator days [104]. More recent data however suggests an incidence rate of 1.33 per 1,000 ventilator days [4]. VAP has been associated with higher mortality, increased length of ICU stay, and greater hospitalization costs. The diagnosis of VAP is extremely difficult as there is no “Gold Standard” diagnostic test. The usual clinical signs of fever, elevated white cell count, change in quality of endobronchial secretions and the presence of a new infiltrate on a portable chest radiograph lack sensitivity and specificity. It is therefore likely that many patients who are diagnosed with VAP don’t have VAP and many patients have an undiagnosed VAP. The misdiagnosis of VAP will lead to unnecessary, prolonged and harmful antibiotic treatment. Nussenblatt and colleagues evaluated patients treated for a VAP in 6 ICUs over a 1 year period [105]. A multidisciplinary adjudication committee determined whether the ICU team’s VAP diagnosis and therapy were justified, using clinical, microbiologic, and radiographic data. In this study 58.4 % of cases treated for VAP were determined not to have VAP by the committee. Surveillance definitions for VAP have been used by the Centers for Disease Control (CDC) to compare rates between hospitals in the United States. New surveillance definitions for VAP were developed by the CDC in 2013 [106, 107]. These surveillance definitions have little clinical utility (discussed below).
While it is clearly evident that almost all CAUTIs have no clinical significance, what about the clinical significance of VAPs? Do critically ill patients with multi-organ failure who develop a VAP die from the VAP or is the VAP just an epiphenomena of critical illness. In a cohort of 2,873 patients of whom 434 developed VAP, Nguile-Makao and colleagues estimated an attributable mortality of 8.1 % [108]. In this study, attributable mortality was higher in surgical patients and patients with an intermediate severity of illness score (SAPS II) at ICU admission. Melsen and colleagues determined the attributable mortality of VAP by performing a meta-analysis of individual patient data from randomized prevention studies [109]. Individual patient data were available for 6,284 patients from 24 trials. The overall attributable mortality was 13 %. Most importantly the attributable mortality was close to zero in trauma, medical patients, and patients with low or high severity of illness scores. Highest cumulative risks for dying from VAP were noted for surgical patients and patients with midrange severity scores at admission (APACHE II Score between 20 and 29). This study suggests that in medical and trauma patients VAP occurs as part the multi-organ dysfunction syndrome and is merely a concomitant epiphenomena. However, in patients undergoing surgery, VAP is an important complication which contributes to excessive mortality. This finding has important implications for the adoption of measures to “prevent” VAP and for the treatment of VAP.
Pathogenesis of VAP
In mechanically ventilated patients colonization of the oropharynx with potentially pathogenic organisms (PPO) occurs within 36 h of intubation with colonization of the endotracheal biofilm within 96 h [110]. Furthermore, endotracheal intubation compromises the patient’s natural anatomic barriers (glottis, larynx, ciliated epithelium and mucus). Subglottic pooling of colonized oropharyngeal secretions with subsequent leakage of secretions around the endotracheal cuff allows PPO to gain entry to the lower respiratory tract [111]. Patients are unable to cough and the mucociliary escalator is rendered ineffective by endotracheal intubation. Intubated patients are therefore unable to clear these colonized secretion from the lower respiratory tract predisposing to both infection and atelectasis. The “aspiration” of colonized oropharyngeal secretions is therefore believed to be the major pathogenic mechanism causing VAP [112]. Using molecular bio-typing Bahrani-Mougeeot and colleagues demonstrated that 88 % of patients with VAP had the same bacteria isolated from the lung (by bronchoalveolar lavage) as their oral cavity [113].
The major risk factors for VAP include factors that increase oropharyngeal colonization, increase the risk or degree of aspiration as well as factors that impair local defense mechanisms. The major risk factors include:
Supine positioning
Previous antibiotics (esp. broad spectrum)
Ventilation lasting greater than 7 days
Reintubation
Intra-hospital patient transport
Chronic obstructive pulmonary disease
ARDS
Thoraco-abdominal surgery
Trauma
Burns
Central nervous system disease
The common pathogens causing VAP include:
Pseudomonas aeruginosa
Methicillin resistant Staphylococcus aureus (MRSA)
Klebsiella pneumonia
Acinetobacter species
Stenotrophomonas maltophilia
Streptococcus pneumoniae (early VAP)
Haemophilus influenzae (early VAP)
Less common pathogens include:
Escherichia coli
Enterobacter spp.
Citrobacter spp.
Serratia spp.
Legionella spp.
Colonization (often extensive) of the respiratory tract with Candida species is common in mechanically ventilated patients [114]. However, invasive Candida pneumonia is extremely rare. Respiratory tract colonization with Candida species has been reported to be an independent predictor of mortality; this is likely related to the fact that colonization is associated with severity of illness and prior use of broad-spectrum antibiotics rather than being a direct contributor to excess mortality [115]. There is no data to suggest that colonization of the respiratory tract with Candia species, nor its presence in high concentration in quantitative culture requires treatment with anti-fungal agents. Aspergillus fumigatus may occur in organ transplant, immunocompromised and neutropenic patients; an environmental source such as contaminated air ducts or hospital construction should be suspected.
Polymicrobial infection is common. Importantly, the incidence of VAP caused by MDR organisms is increasing [116]. VAP caused by MDR organism(s) is associated with increased mortality [111, 117, 118]. Risk factors for infection by MDR organisms include:
Intubation for longer than 7 days
Previous broad spectrum antibiotics
Hemodialysis
Hospitalization for 2 days or more (in the last 90 days)
Prior to admission to the ICU
Immunosuppression
Poor functional status
Taking these factors into account, patients with suspected VAP should be divided into two groups; namely those at high or low risk of having an infection with multi-resistant pathogens [119]. This is similar to the approach for treating patients with other types of pneumonia (see Chap. 17) This classification is more useful that the traditional distinction between early and late VAP [120, 121].
Diagnosis of VAP
The clinical criteria that have “traditionally” been used to diagnose VAP include: a new or progressive pulmonary infiltrate, fever, leukocytosis and purulent trachea-bronchial secretions. These clinical criteria are however non-specific and of little clinical utility in the diagnosis of VAP [122, 123]. An autopsy investigation demonstrated that only 52 % of patients with pneumonia at autopsy had a localized infiltrate on their chest radiograph and that 40 % did not have a leukocytosis close to their death [124]. The Clinical Pulmonary Infection Score (CPIS) was developed as a “non-invasive” method to diagnose VAP, and uses a combination of clinical features together with the culture of a tracheal aspirate to diagnose pneumonia [125]. The CPIS assigns 0–12 points based on six clinical criteria:
Fever
Leukocyte count
Oxygenation
Quantity and purulence of secretions
Type of radiographic abnormality
Results of sputum (tracheal aspirate) gram stain and culture.
Both the original CPIS and the modified CPIS have, however, proven unreliable for the diagnosis of VAP, with a low sensitivity and specificity with considerable inter-observer variability in the calculation of the score [123, 126–128]. It should be emphasized that the upper respiratory tract of intubated patients are rapidly colonized with PPO and that gram stain and culture of tracheal aspirates are unable to distinguish between upper airway colonization and lower respiratory tract infection (pneumonia).
As the clinical criteria of VAP lack specificity, a number of diagnostic techniques have been reported which attempt to distinguish between patients with lung infection as opposed to those colonized with potentially pathogenic organisms or those with a tracheo-bronchitis. Lower respiratory tract sampling is based on the premise that the lower respiratory tract is normally sterile, that there is a good correlation between the concentration of bacteria in the lung and the severity of the pulmonary inflammatory process and that bronchoalveolar lavage (BAL) quantitative culture closely correlates with the concentration of bacteria in the lung [129, 130]. BAL specimens may be obtained bronchoscopically or blindly using the mini-BAL technique [125, 131]. The advantages of m-BAL is that bronchoscopy is not required and that sampling can readily and safely be performed by trained respiratory care practitioners [132, 133]. Effective antibiotic therapy causes a rapid decline in the BAL bacterial load within 24 h. BAL sampling should therefore be performed prior to the initiation of antibiotics. False negative results are therefore more common in patients who have had a new antibiotic instituted within 72 h of sampling. To lower the risk of not treating patients with VAP (false negatives) we suggest a diagnostic threshold of 104. While this “quantitative” diagnostic approach appears to be logical and is supported by experimental studies it has not been proven to have a major impact on clinical outcomes [134]. Furthermore, procalcitonin (PCT) appears to have a poor diagnostic accuracy for VAP (for reasons that are unclear), PCT may be used to guide the duration of antibiotic therapy [135, 136].
