Section III Gayatri Sonti, Dan Miulli, and Javed Siddiqi The skull is a solid box within which the volume of all the components—the brain, cerebrospinal fluid (CSF), and blood—should remain constant. Alteration in pressure in one compartment of the skull is compensated by volume changes in the other. The energy requirements of the brain are met from oxygenation of glucose. In addition, the autoregulation of the cerebral vasculature attempts to maintain adequate perfusion to the brain.1,2 The homeostasis of the brain results from a balance of the above mechanisms. In the neurologically injured patient, this homeostasis is altered and must be understood. The treatment of patients in the NICU includes a thorough understanding of cerebral metabolism, intracranial pressure, cerebral perfusion pressure, management of fluids and electrolytes, and the correction of these parameters to achieve homeostasis after brain injury (Table 13–1).
Pathophysiology
13
Homeostatic Mechanisms in the Neurosurgical Intensive Care Unit Patient
Homeostasis of the Brain
CPP | >70-95 or >60-95 if concerns about secondary injury from treatment |
ICP | 5–15 mm Hg |
Hemoglobin | 10 |
Hematocrit | 30–35% |
Core temperature | 35–37.2°C, 95–99°F |
PaO2 | >97% |
pO2 | >115 mm Hg for 72 hours, then >100 mm Hg |
Serum osmolality | <320 mOsm/L |
pCO2 | 35–40 mm Hg |
Sodium | 140–145 mmol/L |
Glucose | 80–110 mg/dL |
PCWP | 10–14 |
PAD | 12–16 |
CVP | 6–8 |
CVP, central venous pressure; ICP, intracranial pressure; NICU, neurosurgical intensive care unit; PAD, pulmonary artery diastolic pressure, PCWP; pulmonary capillary wedge pressure.
Cerebral Blood Flow and Perfusion
Cerebral blood flow (50–75 mL/100 g/min) is affected by regional cerebral metabolism, cerebral perfusion pressure, and oxygen and carbon dioxide tension.1 The neurointensivists must prevent ischemia. Without blood flow, glucose and glycogen are depleted in 4 minutes. In the ischemic penumbra, there is an increased metabolism attempting to deliver more substrate that results in increased brain temperature.3 If brain tissue oxygen drops below 20 mm Hg, there is anaerobic respiration, followed by glucose storage dependent lactic acid accumulation, mitochondrial damage, decreased pH, vasodilation, failure of Na–K adenosinetriphosphatase (ATPase) pump, and Na and Cl influx followed by water, all which lead to cytotoxic edema.1,2 Failure in the blood–brain barrier leads to vasogenic edema. Hydrogen ions accumulate and pH drops to 6.0 from 6.4, causing inhibition of mitochondrial respiration and failure to sequester calcium, and, in turn, leading to further cell activation in the face of no substrate. The cell dies. Cerebral autoregulation attempts to maintain blood flow according to this or any other change in cerebral metabolism.
Cerebral perfusion is the blood pressure gradient across the cerebral vasculature and usually is equal to the mean arterial pressure minus the intracranial pressure (MAP – ICP). The ability to maintain cerebral autoregulation is affected by pathological conditions such as hyperventilation, edema, and hypoxia. ICP is controlled through regulation of the intracranial constituents of CSF, blood, and brain volume.1,2 The pressure volume index (PVI) is the ability of the brain to compensate for increased intracranial volume. ICP slowly raises up to a point where a slight increase in intracranial volume causes a big change in ICP. When ICP is consistently increased to greater than 20 mm Hg, it may lead to ischemia and herniation, which eventually lead to brain death. The goal in the management of high ICP is to reduce the CSF, blood, and brain volume. This may be accomplished by CSF drainage, prevention of vasodilation, and surgery to remove a portion of the skull, allowing the brain to expand. A decompressive craniectomy may have some benefit in salvageable patients with consistently elevated ICP. Table 13–2 outlines the basic approach to managing elevated ICP and CPP.
|
Note: Initiate hypothermia and barbiturate coma if the above measures fail and if ICP is consistently above 25 mm Hg.
CPP, cerebral perfusion pressure; GCS, Glasgow Coma Scale; HOB, head of bed; ICP, intracranial pressure.
Control of Blood Pressure
The CPP should be maintained >70 mm Hg and below 100. If there is a concern about secondary injury from therapy, consider maintaining CPP above 60 mm Hg. Significant decreases in blood pressure are a direct effect of ICP treatments using sedation, paralytics, or diuretics. In these hypotensive situations, moderate intravenous (IV) fluids followed by vasopressors can elevate blood pressure. In brain injury, the autoregulation of cerebral blood flow is altered; if CPP increases above 95 to 125, there can be an increase in blood volume and an increase in ICP. The first-line antihypertensive medication should be β-blockers or angiotensin-converting enzyme (ACE) inhibitors. Other medications such as nitrogen-containing compounds act quickly, but because they cause a direct vasodilator effect, they increase ICP.2
Control of Serum Glucose
Tight control of glucose concentration is essential in head injury patients. Hypoglycemia of 50 mg/dL causes neuronal injury by changing cerebral blood flow and cerebral metabolism. In addition, glucose levels >110 to 150 mg/dL in nondiabetics and >200 to 250 mg/dL in diabetics also have deleterious effects. IV regular insulin infusion and frequent blood glucose checks are essential to maintain glucose levels between 80 and 110 mg/dL.4
Fluids and Electrolyte Balance
The principles of homeostasis of body water are as follows: ⅔ of total fluid resides inside cells, and ⅓ of total fluid resides in the extracellular space. The extracellular space is composed of ⅓ plasma and ⅔ interstitial fluid. The osmotic forces created by solutes determine the movement of water across membranes. An estimate of serum osmolality is determined by the equation
2Na + BUN/2.8 + glucose/18,
where BUN is the blood urea nitrogen level.
Sodium is the main determinant of osmotic force because it does not easily cross the membrane. Therefore, an imbalance in sodium concentration and volume status results in disturbance in homeostasis.1 The most common conditions seen are diabetes insipidus, syndrome of inappropriate antidiuretic hormone (SIADH), and cerebral salt wasting (CSW) (Table 13–3).1,5
Hypernatremia
Hypernatremia is usually caused by hypovolemia due to free water loss. It is often related to defective antidiuretic hormone (ADH) release or due to osmotic diuresis. When sodium levels are >160 mmol/L, decreased level of consciousness and confusion set in. Hypernatremia should be treated first with crystalloids, 0.45 or 0.9% NaCl; if needed, colloids such as 5% albumin can be used. Patients with diabetes insipidus should be carefully monitored for fluid balance, body weight, serum and urine sodium, and urine specific gravity. If urine output exceeds the input by 250 mL/hr for 2 consecutive hours, hormonal replacement with IV 1-deamino-8-D-arginine vasopressin (DDAVP, or desmopressin) 0.5 to 2 μg may be administered with caution.5
Hyponatremia
In NICU patients, hyponatremia results primarily from SIADH and CSW. In SIADH, the extracellular volume is increased, and CSW is decreased. If sodium is less than 135 mmol/L, due to excessive water retention or sodium excretion, serum osmolality should be measured immediately. An increase in glucose concentration by 100 mg/dL also accounts for a fall in sodium by 1.6 mmol/L and a rise in serum osmolality by 2 mOsm/kg. Patients usually become symptomatic after a drop of sodium below 120 mOsm/L, although mentation changes can be seen with levels below 130 mmol/L in the acutely injured patient. Some of the common symptoms are headache, nausea, and vomiting. Patients may also become lethargic or develop tonicoclonic seizures at low levels. Hyponatremia should be corrected slowly to prevent central pontine myelinolysis.1,2
Hypothermia and Brain Injury
Brain temperature is higher than the core body temperature by 1°C. Several studies demonstrate that in head-injured patients, there are moderate to severe elevations in brain temperature. Hyperthermia is neurotoxic, and hypothermia attenuates these effects. Mild hypothermia is between 32 and 35°C and moderate hypothermia, 30 and 33°C.6,7 Although spontaneous hypothermia and hyperthermia result from damage to the hypothalamus, several studies demonstrate the neuroprotective effects of iatrogenic-induced hypothermia.3 Hypothermia can be induced by antipyretic medication, surface cooling using hypothermia blankets, gastric lavage with ice-cold water, or saline. However, the most efficient way is by an endovascular cooling device placed in the inferior vena cava via the femoral vein.8 The duration, speed of cooling, and rate of rewarming are likely factors in determining whether hypothermia will be effective. Tables 13–4 and 13–5 outline the advantages and disadvantages of hypothermia for brain-injured patients.9–13
|
CPP, cerebral perfusion pressure; ICP, intracranial pressure.
|
< div class='tao-gold-member'>
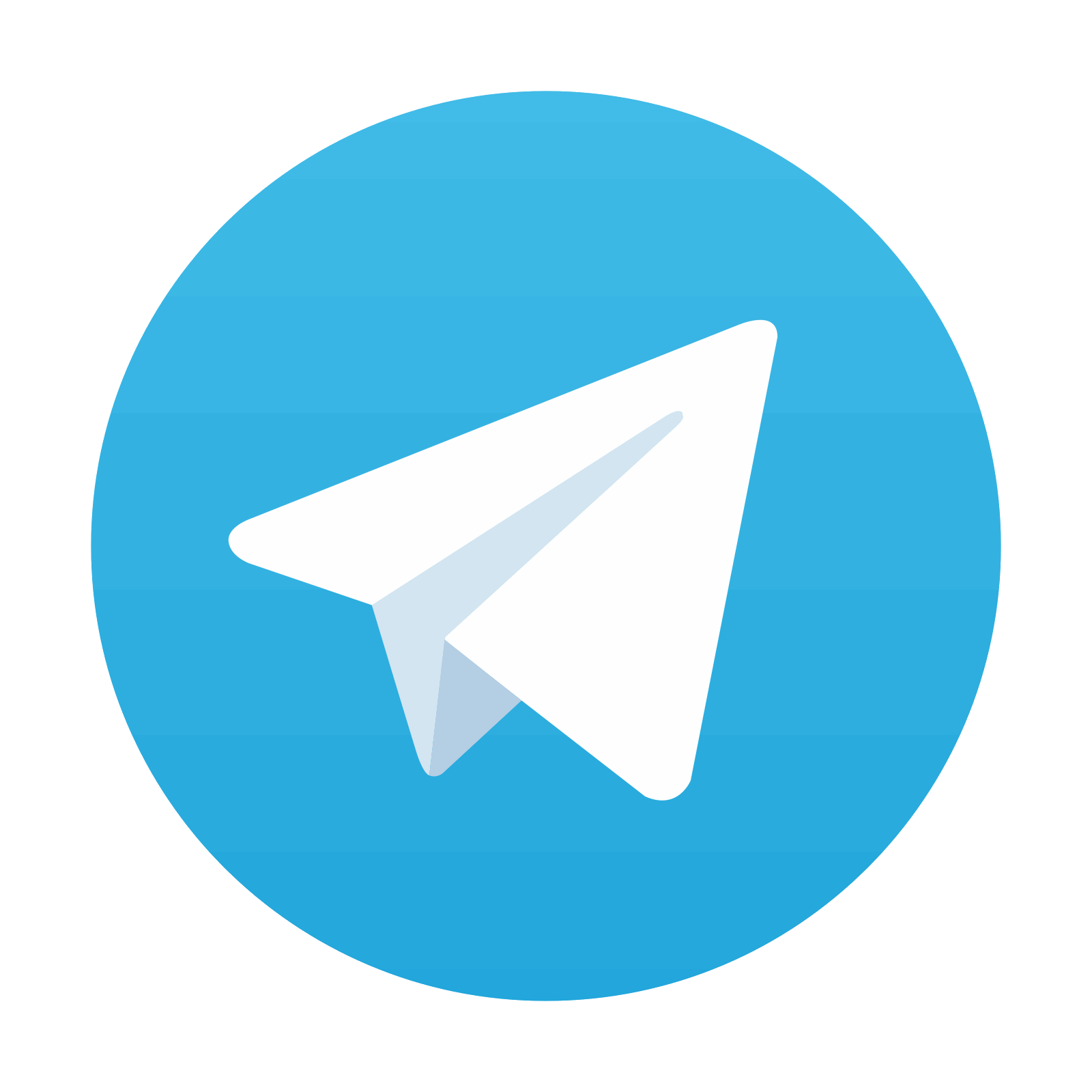