© Springer International Publishing Switzerland 2016
Karen Stuart-Smith (ed.)Perioperative Medicine – Current Controversies10.1007/978-3-319-28821-5_1111. Hemostatic Resuscitation for Perioperative Bleeding
(1)
Division of Critical Care, Department of Pediatrics, Washington University in St Louis, St. Louis, MO, USA
(2)
U.S. Army Institute of Surgical Research, FT Sam Houston, TX, USA
Abstract
The perioperative resuscitation approach to hemorrhagic shock is changing due to recent military experience in managing traumatic injury. For the past few decades, resuscitation of perioperative hemorrhagic shock has most commonly consisted of crystalloids and colloids, followed by RBCs, after which, if indicated according to laboratory results, plasma and platelets. If used, empiric ratios of RBC to plasma units ranged between 10 and 4 units of RBCs to 1 unit of plasma, without platelets. This approach was the foundation of Advanced Trauma Life Support (ATLS).
Recent combat experience re-ignited interest in whole blood for the resuscitation of hemorrhagic shock. US Army data indicates that warm whole blood transfusion is associated with improved or comparable survival compared to blood components. This data compliments that of pediatric cardiac surgery trials which found that cold-stored whole blood reduced blood loss and was associated with improved platelet function compared to blood components in a 1:1:1 ratio using platelets were stored at 22 °C.
Whole blood availability is limited in civilian hospitals due to perceived logistical barriers, but recent developments suggest that these barriers are obsolete. Data indicates it is safer to provide group O whole blood than ABO-specific whole blood; whole blood can be leukocyte-reduced with platelet-sparring filters; and whole blood stored at 4 °C retains platelet function during 15 days of storage. This approach will improve the availability of whole blood and facilitate its use for peri-operative hemorrhagic shock. There are also logistic and economical advantages of whole blood based resuscitation compared to components for hemorrhagic shock.
Keywords
TraumaCoagulopathyShockHemorrhageTransfusion11.1 Hemostatic Resuscitation for Perioperative Bleeding
Damage Control Resuscitation (DCR) and hemostatic resuscitation are concepts recently developed to optimize resuscitative and transfusion approaches to patients with hemorrhagic shock and immediately life-threatening injuries. DCR has many components all of which are aimed at preventing or treating shock and coagulopathy and thereby reducing morbidity and mortality from severe traumatic injuries causing massive hemorrhage [1]. Hemostatic resuscitation is the central tenant of DCR. This is a concept that developed with the recognition that a blood based transfusion strategy would be optimal for severe bleeding and that crystalloid or colloid-based resuscitation cause hemodilution and acidosis, which aggravate the underlying coagulation and metabolic disorders that evolve following injury and blood loss. The ultimate hemostatic resuscitation product is whole blood, but since currently it is not commonly available in the developed world many substitute the transfusion of high ratios of plasma and platelets to RBCs that range between 1:1:2 and 1:1:1 units, respectively. Goal-directed hemostatic resuscitation is being explored as a method to alter empiric ratios of blood products and provide specific therapies based upon the rapid results from point-of-care coagulation and shock monitoring.
Recent conflicts in Iraq and Afghanistan re-ignited interest in the concept of transfusing whole blood or “reconstituted whole blood” with components in a 1:1:2–1:1:1 unit ratio as a result of many factors. The large volume of patients presenting in hemorrhagic shock with high mortality forced physicians to think of alternative methods to improve outcomes. Prior to 2005, platelet units were not available in Iraq or Afghanistan and even after that they were in limited supply. There was increased awareness that shock can directly lead to coagulopathy and that a balanced resuscitation that corrected both conditions might reduce death from hemorrhage. Physicians with prior combat experience maintained institutional knowledge of prior successful deployments of whole blood in previous conflicts and the ease with which such a program could be implemented and maintained. As a result, the use of whole blood at large combat support hospitals increased in 2003–2004 from a “rescue” therapy in the initial phases to a more widely adopted strategy that was employed earlier in the resuscitation of patients with life-threatening hemorrhagic shock. It was during the period of 2004–2006 that the concepts of DCR were solidifying into one bundle of care, with hemostatic resuscitation as the centerpiece of clinical practice guidelines (CPGs) for the treatment of severe traumatic hemorrhagic shock. The DCR concept and specific use of whole blood were codified into on-line CPGs and routinely re-evaluated and updated by the US Department of Defense Joint Trauma System.
The use of whole blood has a long history in military medicine, which began almost 100 years ago in World War I. Briefly, in World War I British and American forces supplied the front line hospitals with low titer Group O whole blood as the universal donor product. In WWII the lessons from WWI were initially side-lined and US forces planned for a plasma-based resuscitation strategy with freeze-dried plasma and albumin. This approach, though practical, was found to be inadequate to the task of preventing or reversing shock physiology. The British Forces primarily transfused whole blood during WWII based on the concept that both shock and coagulopathy needed to be equally addressed for optimal outcomes. After the US leadership recognized the successes the British Forces were having with this approach they also shifted back to a whole blood based resuscitation strategy [2].
US Forces in the World War II established what were termed “Field Blood Banks,” where fresh whole blood was collected from immediately available donors and either used on site immediately, or packaged and delivered as far forward as possible for resuscitation near point of wounding. Units actively engaged in combat used freeze-dried plasma and any available whole blood until casualties could be evacuated to surgical facilities. This approach was replicated successfully in Korea where Group O low titer (<1:200 by saline dilution) whole blood was used almost exclusively [3]. In Vietnam, universal donor whole blood was the primary resuscitation agent until apheresis derived fresh frozen AB plasma was introduced in 1968. Eventually type-specific whole blood became available, but universal donor whole blood was the only blood product used in forward, pre-hospital settings [2].
During and after the Vietnam War Era, crystalloids and colloids became the primary resuscitative solution for hemorrhagic shock, replacing blood products including whole blood. This was in part due to the risks of infectious disease transmission with blood products, but also due to research performed by Carrico and Shires that indicating that the interstitial compartment or “third space” needed to be resuscitated with 1–2 L crystalloids in order to perfuse the tissues, after which the use transfusion of whole blood would be indicated only if hemodynamic instability persisted [4]. Their philosophy for resuscitation in patients with traumatic bleeding was misapplied and led to the overuse of crystalloids, to the detriment of patients with severe bleeding who commonly received 5–10 L of crystalloids prior to any blood product administration. The result was dilutional coagulopathy and severe interstitial edema. In addition, as whole blood became unavailable the use of RBCs took the place of whole blood. The lack of recognition that RBC units were not as efficacious at treating both shock and coagulopathy further exacerbated dilutional coagulopathy. The misapplication of the Carrico and Shires data and the disappearance of whole blood from the clinical armamentarium ushered in an era of ARDS, abdominal compartment syndrome, multi-organ failure and anasarca in ICUs [5, 6]. These outcomes were actually predicted by Shoemaker in 1976, where he challenges the notion that the interstitial compartment required resuscitation and emphasized the need for whole blood for the treatment of significant bleeding when the hematocrit fell below 30 % [7]. Subsequent research has demonstrated that a crystalloid-based resuscitation strategy, leads to increased inflammation and vascular permeability compared to whole blood [8, 9].
The pendulum has now swung back to focusing on a blood-based strategy for patients in hemorrhagic shock. The concept of hemostatic resuscitation as a component of DCR continues to be controversial, with unprecedented attention given to establishing the optimal resuscitation strategy for patients with hemorrhagic shock. Investigations continue to seek novel methods to further improve outcomes by establishing goal-directed hemostatic resuscitation strategies with point-of-care monitoring of shock and functional hemostatic parameters. Perioperative bleeding occurs in many different settings or in patients with varying disorders, requiring caution when extrapolating concepts derived primarily from patients with traumatic injury. Although there are increasing reports of improved outcomes when hemostatic resuscitation principles are used in patients with ruptured aortic aneurysms and in post-partum hemorrhage for example, data outside the trauma literature is still scant [10–13]. Recognizing the lack of robust data available to support the development of optimal resuscitative strategies in a wide variety of perioperative patients with hemorrhagic shock, the following will review the evidence regarding the use of whole blood and blood components in these patient populations.
11.1.1 Whole Blood Definitions
Whole blood is collected into 450–500 ml bags and then transfused or stored instead of being separated into components. When stored cold at 4 °C it has a shelf life of 21 days if collected in CPD, CP2D or ACD-A anticoagulant and 35 days if collected in CPDA-1. When collected in this manner and standard transfusion-transmitted disease testing is performed it is an approved product. The FDA Circular of Information for Use of Human Blood and Blood Components states that whole blood is indicated for symptomatic anemia with large volume deficits [14]. The FDA also states that whole blood must be type-specific. To differentiate this product from whole blood used in military settings, it is called Cold Whole Blood (CWB). When cold whole blood is used within 48 h of collection it is Cold Fresh Whole Blood (CFWB).
In military or austere settings, where formal testing for TTD’s is not possible, whole blood is collected from pre-tested donors (tested pre-deployment and every 90 days thereafter) and stored warm at 22 °C for up to 8 h and is then stored for a maximum of 24 h at 4 °C. It is typically transfused immediately. This product is termed Warm Fresh Whole Blood (WFWB). Since WFWB is transfused before results of TTD testing are available (testing of each unit is retrospective since samples are sent from expeditionary forces to US labs for testing); it is thus not approvable by FDA and presents a higher risk of disease transmission and is reserved for situations in which tested blood products are unavailable or ineffective. It is important to understand the differences between cold and warm storage of whole blood since there are reports of both in the literature.
The use of the term “fresh” when storage is less than 48 h is arbitrary and not based on data regarding the loss of RBC, plasma or platelet function. It is related to the timing of the removal of platelets from the circulation and the perceived lack of function after this amount of time. The focus on timing of platelet removal from the circulation is a result of decades-old emphasis on circulation time as a measure of function instead of assessing function as a measure of hemostatic capacity. Arguably hemostatic capacity is a more relevant measure of function in patients with severe bleeding who are at high risk of death within hours than circulation time.
Whole blood, stored cold or transfused as WFWB can be leukocyte-reduced with platelet-sparing filters [15]. Leukoreduction decreases the white blood cell count in the whole blood unit to less than 5 × 106.
11.1.2 Cold Whole Blood
The two largest RCTs that have exclusively compared whole blood to blood components were performed in pediatric cardiac surgery patients. These two trials with significantly different methods reported contradictory results. In a double-blinded study, Manno et al. evaluated postoperative blood loss in 161 children undergoing open-heart surgery with cardiopulmonary bypass whose immediate postoperative transfusion requirements were met with either whole blood or reconstituted whole blood (packed RBCs, FFP, and platelets) [16]. The transfusion of whole blood was associated with significantly less postoperative blood loss than the transfusion of packed RBCs, FFP, and platelets in children under 2 of age who underwent complex cardiac surgery (mean 24-h post-operative blood loss in milliliters per kilogram 52.3 ± 10.8 vs. 96.2 ± 10.7, P = .001). In that study, whole blood was associated with improved platelet function according to aggregometry results. Mou et al. compared the use of fresh whole blood with the use of a combination of packed RBCs and FFP (reconstituted blood) for priming of the cardiopulmonary bypass circuit in children less than 1 year of age undergoing cardiac surgery [17]. Children were randomly assigned to receive either fresh whole blood (96 subjects) or reconstituted blood (104 subjects) for bypass-circuit priming. This study did not continue randomization of the intervention in the ICU, where outcomes were measured. The use of fresh whole blood for cardiopulmonary bypass priming had no advantage over the use of a combination of packed RBCs and FFP during surgery for congenital heart disease. Moreover, circuit priming with fresh whole blood was associated with an increased length of stay in the ICU and increased perioperative fluid overload. Unfortunately, this secondary outcome was not adjusted for confounding variables.
The only available prospective evidence in trauma patients that utilized whole blood came from a single small RCT of 107 patients requiring massive transfusion [18]. One arm received leukoreduced whole blood (with platelets removed during filtration) supplemented with platelets, and the other was treated exclusively with components (RBCs, plasma, platelet units). While the primary outcome of 24-h transfusion volume was equivalent between groups, a secondary analysis excluding patients with severe TBI demonstrated reduced 24-h blood use for the modified whole blood group. It is difficult to know whether the addition of platelets to whole blood sufficiently changed the results to limit generalizability, thus the study was insufficient to definitively establish the efficacy of whole blood.
A small RCT published in 1990 that compared WFWB to CFWB enrolled 36 adult patients requiring cardiac surgery and cardiopulmonary bypass. The hemostatic effect of fresh non-leukoreduced WFWB was compared to CFWB stored at 4 °C for both 5 and 24 h, and investigators found that blood loss increased postoperatively in those transfused with whole blood stored at 4 °C, suggesting that WFWB may be superior to 4 °C-stored WB [19]. Since WFWB is not clinically available, except for in austere or military environments, these results may be relevant only for this population of patients.
In vitro data indicates that the hemostatic capacity of whole blood is higher than that of blood reconstituted from individual components in a 1:1:1 ratio [19]. In one analysis in which platelets were stored at 22 °C for up to 5 days, and RBCs were stored at 4 °C for up to 35 days, investigators compared reconstituted whole blood to WFWB and found that the hemostatic capacity of platelets was reduced as storage duration increased [20]. Conversely, as RBC and platelet storage age increased more thrombin-antithrombin complexes were detected, indicating increased thrombin generation, which was confirmed in a thrombin generation assay. Similarly, WFWB platelet aggregation response to collagen was consistently better than that of reconstituted whole blood. A similar in vitro study compared warm and cold whole blood with and without additional platelets to reconstituted whole blood with components in a 1:1:1 unit ratio. No differences in platelet function or viscoelastic monitoring results were noted between warm and cold whole blood and reconstituted whole blood when RBCs and platelets were at their maximum storage duration [21]. Differences in study methods reported for these two studies may account for the conflicting results.
In vitro studies also indicate that considerable CWB hemostatic capacity is maintained for at least 14 days. One analysis of non-leukoreduced whole blood stored at 4C reported normal platelet function as reflected by TEG-MA values for all 21 samples out to 14 days of storage, with the median TEG-MA values within normal range out to 31 days of storage [22]. In addition light transmission aggregometry analysis of platelet function exhibited no change from Day 1 to Day 21 with adenosine diphosphate and epinephrine stimulation, but did report a decline in response to collagen (Day 7) and ristocetin (Day 17). These results were reported without adjusting a dramatic reduction in platelet count over time, which dropped to a median value of less than 100 × 109/L by day 7 of storage.
In vitro measures of hemostasis studied in whole blood units stored at 4 °C versus 22 °C over 21 days reported superior PT/PTT, impedance platelet aggregation, and TEG results in cold-stored blood [23]. In this study non-leukocyte reduced whole blood was stored for up to 21 days. Whole blood at 4 °C was superior to those units stored at 22 °C based on PT/PTT, impedance platelet aggregation, and TEG parameters. Refrigeration of whole blood units also increased shear-induced platelet aggregation and ristocetin-induced platelet agglutination as well as the proportion of GPIb-expressing platelets. Furthermore, platelet function in stored whole blood measured by shear- and Von Willebrand factor-dependent methodology is retained at 4 °C for up to 7 days [24].
These findings are similar to those regarding platelet units stored at 4 °C. Refrigerated PLTs perform better than room temperature–stored PLTs in aggregation assays as has been reported by multiple investigative teams [25–29]. This includes an RCT demonstrating that platelets at 4 °C were superior in reducing bleeding time in both patients on aspirin and in thrombocytopenic patients when compared to platelets at 22 °C [30].
11.1.3 Warm Whole Blood
Retrospective data in adults, further support the use of whole blood compared to exclusive use of components, including an analysis of US casualties with 100 % follow up which reported an independent association between survival and the addition of whole blood as an adjunct to resuscitation [31]. Additionally, a study of US and non-US casualties reported no difference in outcomes for those transfused whole blood as part of their resuscitation compared to those who only received blood components; however, results should be viewed with caution as approximately 33 % of patients were lost to follow-up [32]. These two studies of combat casualties are unique in that the whole blood transfused in combat was given immediately after collection or was stored very briefly (<24 h). They also differ from civilian data in that whole blood accounted for only a portion of the total blood used for resuscitation during the initial 24 h of admission. In the study that found an association with survival, 30 % of the 24-h blood volume transfused was whole blood, whereas in the study that demonstrated equivalence, only 20 % of the 24-h blood volume transfused was whole blood.
In a retrospective civilian study, the use of whole blood as part of a resuscitation was associated with similar outcomes compared to the exclusive use of blood components. In patients with multiple etiologies of hemorrhagic shock requiring massive transfusion, there were no differences in blood utilization rates and clinical outcomes [33].
Two small RCTs have compared WFWB to platelet concentrates stored at 22°C. One published in 1989, of 24 patients requiring cardiopulmonary bypass compared WFWB to platelet concentrates stored at 22°C in patients undergoing cardiac surgery [34]. A scanning electron microscope was used to assess platelet aggregation. Their results indicated that one unit of WFWB had improved hemostatic effect compared to 8–10 whole blood derived platelet units. In another small randomized study, published in 1988, of 27 patients requiring cardiopulmonary bypass, WFWB was also found to be associated with improved platelet function compared to platelet units stored at 22 °C as assessed with aggregation studies [35].
11.1.4 Whole Blood Availability
Whole blood is widely available in much of the world, particularly in developing nations such as those of Sub-Saharan Africa. For example, in Kenya at the Kijabe Hospital, CWB is commonly provided, where it is stored at 4 °C for up to 35 days, is typically group O, and is not leukocyte reduced (Kibuchi J and Letchford S, 2015, oral communication, 15th August).
In the developed world, the clinical availability of whole blood since the 1970s to 1980s has been dramatically reduced. WFWB essentially disappeared from US hospitals during the early days of the HIV epidemic. CWB, though fully tested for TTDs also vanished from blood banks. This limitation of whole blood availability has been driven due to four main concerns about whole blood.
The first is that regulatory bodies have mandated that whole blood be ABO-specific due to risks of ABO incompatibility, where ABO incompatible RBCs will cause severe hemolytic reactions and ABO incompatible plasma might uncommonly cause mild to moderate hemolytic reactions. Providing ABO specific WB introduces a significant logistical concern since maintaining sufficient inventory to ensure a ready supply of every ABO type would result in significant cost and waste.
The second barrier has been the lack of a platelet-sparing leukoreduction filter. Since leukoreduction has become either mandatory in most countries or widely used in others, the fact that whole blood could not be leukocyte reduced while maintaining platelets reduced the supply of whole blood.
The third barrier is due to the concern that the platelets in CWB stored at 4 °C might not be functional or viable, calling into question whether WB is truly a platelet-containing product. Due to this concern when whole blood is used in most developed countries it is used within 48–72 h of storage, even though it is licensed for storage between 21 and 35 days. Even in the rare US hospitals where WB is used, most transfusions occur within 7 days of collection. This is in contrast to hospitals in the developing world that transfuse whole blood until the date of expiration if necessary.
A fourth belief that has limited whole blood availability is the notion that blood components are equally efficacious compared to whole blood for patients with shock and coagulopathy. This concept has not been studied well, except in one pediatric cardiac surgery trial, where the use of whole blood did reduce blood loss secondary to improved platelet function compared to blood components [16]. As mentioned above, all other trials either added platelets to whole blood when compared to blood components, or whole blood was only given during a portion of the study period, potentially limiting generalizability [17, 18].
These four issues have caused concern regarding the efficacy, safety and logistic feasibility in providing whole blood for patients with life-threatening bleeding and have led to its dramatic reduction in use in the developed world. As a result of these concerns or beliefs, whole blood is rarely available in the developed countries such as Australia, the UK or Canada (Devine D, Doughty H, Irving D, 2015, oral communication, 15th August). While the concepts above have been challenged in an effort to provide patients with a better, more efficacious resuscitation product, acceptance and implementation have been slow, thus whole blood availability remains limited. Some developed countries do still use whole blood for pediatric transfusion, including France (Sailliol A, 2015, oral communication, 15th August), and in 15 % of children’s hospitals in the US [36]. In both the US and France despite licensing for up to 35 days CWB storage duration is limited to 2 to 7 days in clinical settings (Jobes D and Sailliol A, 2015, oral communication, 15th August).
11.1.4.1 Group-Specific Versus O-Negative Low Titer Cold Whole Blood
Transfusion of type O low titer CWB is an alternative solution to group-specific CWB, but despite being the standard of care for treating hemorrhagic shock up to and during the Vietnam War, it is currently not permitted by AABB standards, although it is permitted in military scenarios during combat operations in a few countries, such as the US, United Kingdom, France, Australia, and Norway [37]. The rationale for this prohibition is difficult to understand. Hundreds of thousands of units of type O whole blood, with low anti-A and anti-B titers to reduce the risk of plasma incompatible ABO reactions, were used during both World Wars, the Korean War, and the war in Vietnam [38–40]. The threshold for low titer set by the US military in the Korean War was <1:256 [41]. A report by Nessen et al. indicated that, at US military forward surgical bases, in a subset of patients, the use of type O whole blood was associated with improved outcomes when compared to RBCs and plasma alone [42]. In addition to decades of use with few reports of adverse complications, there are multiple reasons to suppose that type O low titer CWB is safer than type-specific CWB. While a small risk of severe hemolytic reaction to type O low titer CWB does exist, it is similar to that of transfusing type O RBCs, which are universally available and the standard of care for emergency release at major medical centers. It is the plasma contained in the blood that causes the greatest concern for ABO incompatibility, but the associated hemolytic reaction is typically mild to moderate and the incidence, only 1:120,000 transfusions of type-incompatible platelet units (which contain an equivalent amount of plasma as that found in a unit of WB) in the UK SHOT database, is low [37]. In contrast to this non-fatal risk, type-specific WB transfusion carries a higher risk (1:80,000) of often-fatal severe hemolytic reactions, mainly due to human error in matching donor and recipient appropriately. Therefore, high-quality hemovigilance data reveals that the risks of hemolytic transfusion reactions are likely to be lower, and of much less clinical severity with Type Specific CWB. The use of type O low titer CWB would further reduce the risk of mild to moderate transfusion reactions since the UK shot database data on ABO incompatible plasma risks incorporated platelet transfusions that were not tittered for ABO antibodies. It’s also likely that the risks of human error and ABO mismatch would be higher in the clinical circumstances surrounding massive transfusion protocol activation in a severely bleeding patient. In summary, our current standard practice of requiring whole blood to be ABO specific puts patients at higher risk of severe transfusion reactions.
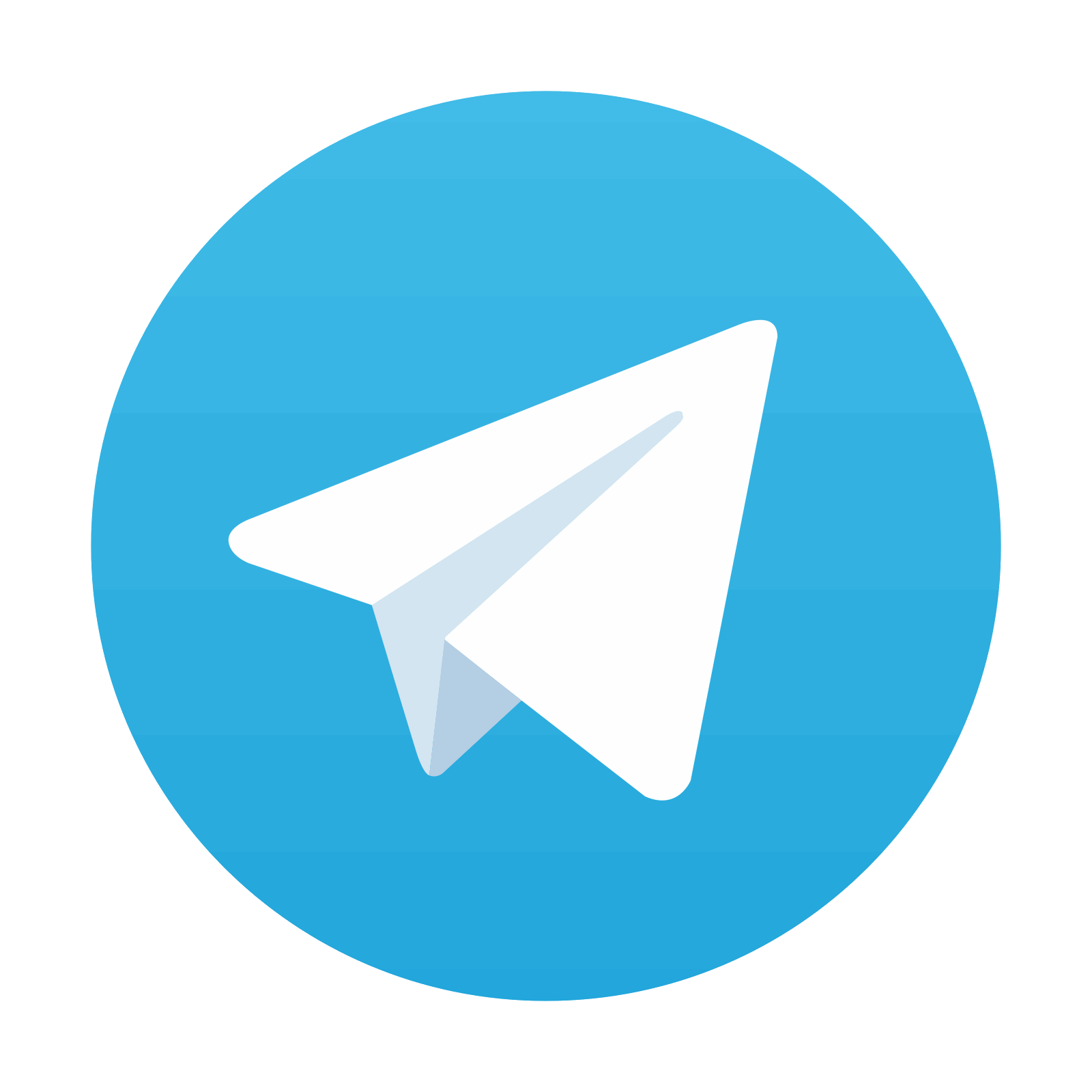
Stay updated, free articles. Join our Telegram channel

Full access? Get Clinical Tree
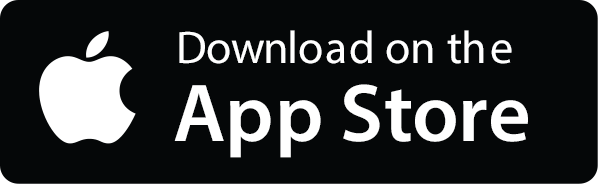
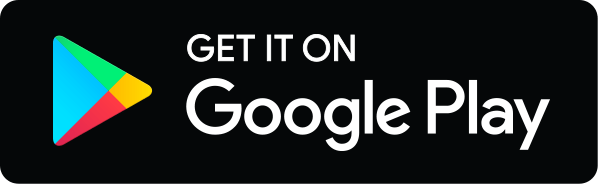
