One of the goals of the cardiovascular system is to ensure optimum oxygen delivery to peripheral tissues. Oxygen delivery to the tissues (DO2, mL O2/min) is defined as the arterial oxygen content (CaO2, mL O2/dL) times the cardiac output (CO, L/min), described as
Where CO (L/min) = Heart Rate (HR, beats/min) × Stroke Volume (SV, L/beat), and CaO2 (mL O2/dL) = (1.34 × [Hgb (g/dL)] × SaO2) + (0.0031 × PaO2 [mmHg]), where Hgb is hemoglobin, SaO2 the arterial saturation of oxygen, and PaO2 is arterial pressure of oxygen.1,2
Oxygen consumption (VO2) is the amount of oxygen utilized by the tissues and is defined by the following equation:
Given its negligible contribution, the dissolved oxygen in plasma can be omitted and the equation simplified, using the arterial concentration of carbon dioxide (CaCO2), the venous concentration of carbon dioxide (CvCO2), and the venous saturation of oxygen (SvO2):
Normal oxygen consumption is 250 mL of oxygen per minute. When there is a stress or imbalance, the body compensates by increasing cardiac output or by increasing oxygen extraction at the tissue level. Increased oxygen extraction can be seen when there is a larger arterial-venous oxygen saturation difference and when the mixed venous oxyhemoglobin saturation (SvO2) is lower than normal. The body also tries to compensate during these times of stress by redistributing blood to these areas of greatest extraction and with selective vasoconstriction of other capillary beds.1,2
Several of these parameters can be measured using various techniques that are static, intermittent, or continuous. In an acute critical illness, it is preferable to perform hemodynamic monitoring at least intermittently, if not continuously. Not only does this type of monitoring help determine progression of the disease process, but it also can evaluate the success or failure of the interventions performed.3-5
As mentioned above, cardiac output is the heart rate times stroke volume. Stroke volume is the amount of blood ejected per beat and depends on preload (end-diastolic tension), contractility, and afterload (end-systolic tension).3 Preload is determined by the pressure gradient between capacitance veins and the right atrium. Pressure in capacitance veins is determined by mean systemic pressure (MSP), right atrial pressure (RAP), and systemic vascular resistance (SVR) via the following equation:
This is regulated by the sympathetic nervous system on the splanchnic circulation and will adapt to maintain adequate venous return.6
According the Frank-Starling law, the increase in myocyte stretching increases the strength of contraction, stroke volume, and afterload. This forms the concept of fluid responsiveness.3 However, there is a point at which further stretching does not further increase the contraction, thus forms the concept of fluid unresponsiveness3 (Fig. 3-1).
FIGURE 3-1
Frank-Starling relationship. The slope of the Frank-Starling curve depends on the ventricular systolic function. Then, one given level of cardiac preload does not help in predicting fluid responsiveness. By contrast, dynamic tests include a preload challenge (either spontaneous, induced by mechanical ventilation, or provoked, by passive leg raising, end-expiratory occlusion, or fluid infusion). Observing the resulting effects on stroke volume allows for the detection of preload responsiveness. EEO = end expiratory occlusion; PLR = passive leg raising.7
(Source: Monnet X, Marik PE, Teboul JL. Prediction of fluid responsiveness: an update. Ann Intensive Care. 2016;6(111):1-11.)

Blood pressure (BP) is CO × SVR. Blood pressure has two components: systolic blood pressure (SBP) and diastolic blood pressure (DBP). Mean arterial pressure (MAP), or average pressure in one cardiac cycle, can be calculated as: [(2 × Diastolic) + Systolic] divided by 3 or MAP = 1/3 (SBP – DBP) + DBP. Generally, the MAP goal is more than or equal to 65 mmHg.
Noninvasive monitoring is often the initial modality, given its ease of use and ubiquitous availability.8 However, hemodynamic instability or vasopressor dependence demand continuous or more frequent assessment of BP than a noninvasive system can provide.
Invasive arterial monitoring is performed by placing a hollow catheter in a peripheral (radial or brachial) or central (axillary or femoral) artery. Arterial pressure changes are continuously transmitted to a noncompressible fluid column that is connected via noncompliant tubing to an electromanometric transducer at the end. The transducer converts mechanical displacement into an electrical signal that generates a waveform. The systolic arterial pulse pressure increases and the diastolic arterial pulse pressure decreases the further away the vessel is from the thoracic aorta. This phenomenon leads to different waveform morphologies,9,10 depending on where the arterial catheter is placed (Fig. 3-2).
FIGURE 3-2
(A) Systolic upstroke or anacrotic limb reflects the left ventricle contraction. (B) Peak systole. (C) Decreasing systole. (D) Incisura or aortic valve closure. (E) Diastole. (right panel from: Gorny DA. Arterial blood pressure measurement technique. Figure 2. AACN Clin Issues Crit Care Nurs. 1993;4(1):66-80. All rights reserved. Used with permission.).

Overdamping (Fig. 3-3) of the system with high resistance or an inefficient transmission of pressure leads to an underestimation of pressure. Conversely, underdamping with either low resistance or excessive oscillation overestimates the actual pressure of the system. The air-fluid interface of the catheter system must be leveled at the phlebostatic axis. This axis is the junction of a vertical line drawn from the fourth intercostal space at the sternum and a horizontal line drawn from the midpoint of a line from the anterior to the posterior of the chest. The phlebostatic axis is a reflection of the level of the atrium in either an upright or supine patient.1 It also must be zeroed to establish hydrostatic zero, a reference point for accuracy. If the air-fluid interface is below this point, the pressure will be falsely high, and if the air-fluid interface is above this point, pressure will be falsely low.
FIGURE 3-3
Invasive arterial waveform in red (A) showing systolic upstroke and a dicrotic notch (arrow), followed by downstroke. (B) An underdamped waveform (can overestimate systolic and underestimate diastolic pressure). (C) An overdamped waveform (can underestimate systolic and overestimate diastolic waveform). Note the mean arterial pressure is less prone to errors related to under- or overdamping.

In addition to the influences of the mean arterial pressure, the arterial waveform is influenced by stroke volume, vascular capacitance, peripheral vascular resistance, and heart rate. A reduction in vascular compliance will result in elevated systolic pressures, while reduced volume diminishes vascular recoil, leading to low diastolic pressures.11,12In patients who are hypovolemic, the incisura or dicrotic notch may appear later in the waveform.
Mechanical ventilation has a profound influence on both right- and left-sided heart pressures when compared to spontaneously breathing patients. While on the ventilator, intrathoracic pressure increases during inspiration. Subsequently, the right ventricular (RV) preload decreases and afterload increases, causing a reduction in the RV stroke volume. This decrease in RV stroke volume causes a decrease in left ventricular (LV) filling after a 2- to 3-beat phase lag and, therefore, decreases the LV stroke volume. This beat-to-beat variation in stroke volume and pulse pressure is more pronounced in hypovolemic patients, and is also influenced by tidal volume, inspiratory pressure, and positive end-expiratory pressure (PEEP). In patients with low tidal volumes and decreased lung compliance, this variation in stroke volume is often masked.11,12
Risks of invasive arterial monitoring include vascular injury, such as a hematoma, or wall dissection. Infection, embolization, or thrombosis of the distal vasculature can also occur and can lead to limb-threatening ischemia.13
The decision to give fluids is based on the increase in stroke volume, but all fluid responders are not necessarily hypovolemic and do not necessarily require volume expansion.14 The iatrogenic phenomenon called volume overload gives credence to this idea. Analysis of the Frank-Starling curve and extravascular lung water (EVLW) shows that as the patient becomes less fluid responsive, the EVLW and edema increase.14-16 (Fig. 3-4) This is worse in patients with prior endothelial damage such as sepsis, acute respiratory distress syndrome (ARDS), and burns.14-16 In addition, natriuretic peptides cleave membrane-bound proteoglycans and glycoproteins off the membrane permeability–regulating endothelial glycocalax and, consequently, increase fluid into the interstitial spaces.14-21
FIGURE 3-4
Superimposition of the Frank-Starling and Marik-Phillips curves demonstrating the effects of increasing preload on SV and lung water in a patient who is preload responsive (A) and nonresponsive (B). With sepsis, the EVLW curve is shifted to the left. EVLW = extravascular lung water; CO = cardiac output; SV = stroke volume. (Source: Marik PE Lemson J. Fluid responsiveness: an evolution of our understanding. Brit J Anaesth. 2014;112(4):617-620.)

Fluid response challenges can be given with a trial of fluid bolus or a passive leg raise (PLR). A PLR is a reversible preload challenge involving a 250- to 500-mL bolus of blood and can be measured in patients with arrhythmias, spontaneous breathing, low tidal volumes, and low lung compliance.7,22 The best sensitivity and specificity is an increase in CO greater than 10%, with a sensitivity of 85% and specificity of 91%.23 Cardiac output effects of PLR reach their maximum within 1 minute. Therefore, continuous monitoring is advocated. PLR can induce changes in: (1) peak velocities of carotids or femoral arteries; (2).bioreactance; (3) cardiac output via pulse contour analysis of arterial wave via photoplethysmography; and (4) in end-tidal CO2.7
Central venous pressure was thought to monitor volume status and fluid responsiveness, but recent literature suggests it is a poor indicator of either.14,24 Static measurements such as pulmonary artery occlusive pressure (PAOP), global end-diastolic volume via transpulmonary thermodilution, and flow time of aortic flow via transesophageal Doppler are poor indicators due to the variability of Frank-Starling mechanics from person to person and from time to time.25-27 Certain authors still found the utility of measuring the central venous pressure (CVP) in shock, since it can be marker of organ perfusion (MAP – CVP), and elevated CVP is associated with acute kidney injury (AKI).27-29
There has been a focus toward dynamic interactions between the heart and lung during positive-pressure ventilation. Dynamic preload indices such as pulse-pressure variation (PPV) and stroke volume variation (SVV) are based on physiologic changes occurring in the preload and afterload conditions of the ventricles with positive-pressure ventilation, as described earlier in the chapter. A stroke volume (and pulse pressure) variation of more than 13% indicates fluid responsiveness in a patient.30
Several devices in common clinical use (pulse index continuous cardiac output [PiCCO], lithium dilutional cardiac output [LiDCO], pressure recording analytical method [PRAM]) are based on arterial pulse waveform or contour analysis. These devices may also include indicator dilution cardiac output for calibration of the pulse contour. The principle of pulse contour analysis is that the area under the curve of the systolic portion of the arterial waveform is proportional to the stroke volume.12 These systems also allow for calculation of pulse-pressure variation (direct, waveform analysis) or stroke-volume variation (indirect, pulse contour analysis). Both pulse-pressure variation and stroke-volume variation are useful indicators of volume responsiveness in a patient in shock.31,32 The accuracy of pulse waveform–based devices is limited in patients with arrhythmias, decreased arterial compliance, and aortic diseases like aortic insufficiency and severe atherosclerosis.
Limitations of pulse pressure variation and stroke volume variation include needing tidal volumes greater than 8 mL/kg and a sinus rhythm, and having a patient not spontaneously breathing. They are also limited in the following clinical scenarios: ARDS, intra-abdominal hypertension, and right-heart failure. In ARDS, tidal volumes are advocated to be 6 mL/kg but can be transiently increased to 8 mL/kg, and fluid responsiveness would be defined as PPV of 3.5% or greater, or SVV of 2.5% or greater.33 However, the low compliance prevents transmission of alveolar pressures to intravascular and cardiac pressures, therefore limiting its use.34 In intra-abdominal hypertension, the respiratory variations of stroke volume are not necessarily related to volume status.35-37 Right ventricular failure can cause increased afterload that might suggest false positive PPV and SVV.27
Bioimpedance cardiography is based on the principle that the thorax is a fluid-filled cylinder (filled with tissue fluid, pulmonary and venous blood volume, and aortic blood volume) that has an intrinsic resistance to the flow of current, or impedance. With systolic and diastolic changes in the fluid content of the thorax, the impedance of the thorax changes. This change in current or impedance is measured by applying low-voltage electrodes on the chest and detecting the difference between systole and diastole. Cardiac output is subsequently calculated with the assumption that the change in voltage correlates with the change in stroke volume.38 In the clinical setting, however, several reports describing a poor correlation between cardiac output using pulmonary artery catheters (PAC) and bioimpedance monitors in cardiac surgery,39 as well as in critically ill patients.30,40 Given its low fidelity, newer monitoring devices have since been developed.
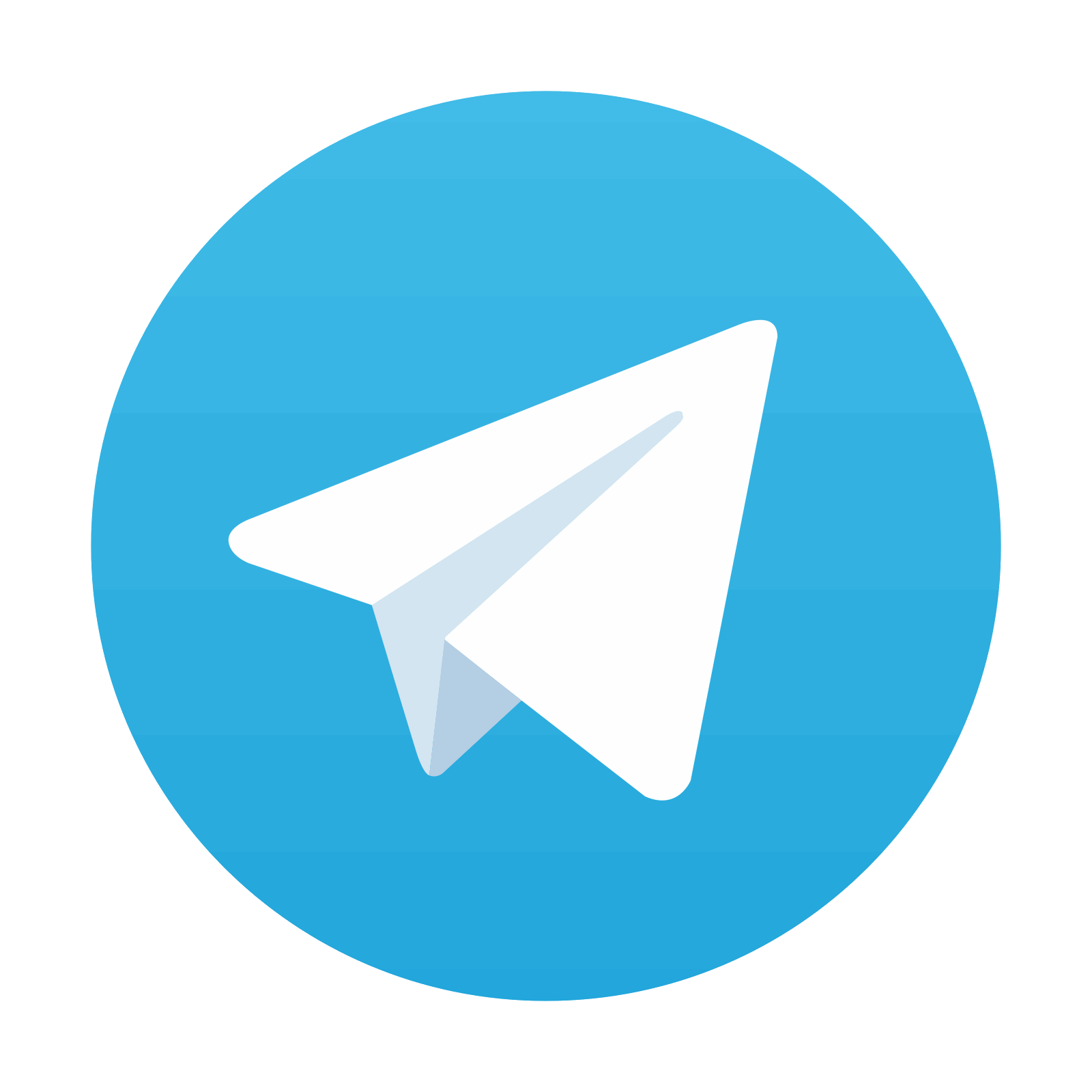
Stay updated, free articles. Join our Telegram channel

Full access? Get Clinical Tree
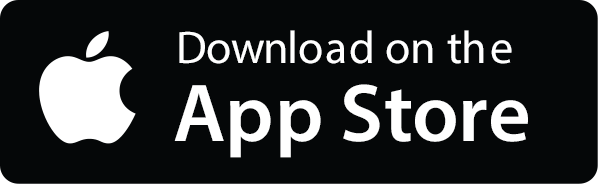
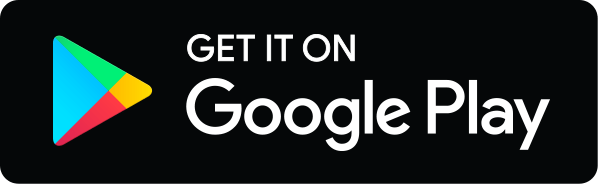