KEYWORDS
pressure transducers: resonance
damping
noninvasive blood pressure measurement
oscillometry
The frequent measurement of a patient’s arterial blood pressure (BP) is standard care in anesthesia. The guidelines state that BP must be measured, but how it is measured is up to you. Is the presence of a pulse an indicator of adequate BP? No, not really. But that was how it was done in the distant past. In some modern situations, however, a finger on a pulse is all you may have to work with until help comes. Is a sphygmomanometer an acceptable way to monitor BP? Yes, it is, if the operator is capable of reliably using one. The problem with sphygmomanometers is that they are labor intensive. But up until the mid 1980s, that is just how most BPs were taken when a patient was under an anesthetic.
Fortunately for us, there are now ways to measure BP that require less work on our part. Noninvasive BP monitoring (NIBP) relies on an oscillometer to give us an accurate BP reading. All we have to do is place the BP cuff on the patient, press “start,” and tell the monitor how often we want it to measure BP. The other method of measuring BP is with an intraarterial catheter. This is an example of invasive monitoring versus the other ways we mentioned, which are classed as noninvasive monitoring.
Of course, the kind of BP monitoring is chosen for use in an anesthetic is a complicated subject. Much of the decision depends on the kind of case and the physical status of the patient. But we will not get into that here. We want you to understand how both invasive and noninvasive BP monitoring works.
INVASIVE BLOOD PRESSURE MONITORING
Depending on the rotation you are on or the type of patient you anesthetize regularly, you may use a pressure transducer every day. Invasive BP monitoring, central venous pressure (CVP) measurements, and pulmonary artery catheter readings all require a transducer somewhere between the patient and the monitor display. (Okay, you could use a columnar manometer, but that would be really inconvenient.)
You can go into as much physics with pressure monitoring and transducers as you want. It would take an electrical engineer or physicist to understand pressure transducers inside and out. Don’t worry; we aren’t engineers or physicists either. So if we can understand it, so can you. The bottom line is that we use these things very frequently, but we have no idea how they work. Yes, it is complicated, but we will try to make it as simple as possible so we all can understand this extremely important but underappreciated marvel. But we will have to talk about some physics to get to where we want to go.
Parts of a Transducer
You can divide a transducer into two main parts, the patient connection and the electrical system. The patient side includes everything from the catheter inside the patient to the transducer diaphragm, and the electrical system is everything from the diaphragm to the monitor. The diaphragm is inside the plastic part where the connector cable and the flush device are. As you may guess, when all this technology hit the operating room (OR) from the physics lab several decades ago, the transducer was not disposable. Now, of course, they are, and it would be interesting to know how many transducers are wasted each year because a resident decided an arterial line or a CVP would be a good idea but his or her attending said, “No, I think we’ll be okay without one.” We will talk first about the physics and different parts of the electrical system of the transducer and then the physics and parts of the patient-to-transducer part of the system.
Physics of the Transducer–Electrical System
In simple terms, a transducer takes one kind of energy and changes it into another kind of energy. In our case, a transducer takes mechanical energy (hydrostatic pressure) and changes it into electrical energy. The transducers we use in the OR are a type of strain gauge. A strain gauge has a diaphragm that oscillates with each pressure waveform, and this movement changes the resistance in a resistor that is part of a Wheatstone bridge.
Now we’re really getting deep into physics. The Wheatstone bridge was originally invented by a man named Samuel Christie in 1833 but was improved by Charles Wheatstone in 1843. (Somehow Christie was unlucky as far as the eponym. But don’t blame Wheatstone; he still called his improvement the Christie bridge, but everyone else started calling it the Wheatstone bridge.)
Back to physics; the Wheatstone bridge is shaped like a four-sided diamond, and each side has a resistor on it. There are two resistors of a known resistance, one resistor that can be made to vary in its amount of resistance and one of an unknown resistance. There is also a source of electricity attached, allowing current to flow through the bridge, because the purpose is to balance both halves of the diamond, so that the sum of resistors 1 and 2 equals the resistance of the variable and the unknown resistor. It is that resistor with the unknown resistance that is attached to our strain gauge diaphragm.
There is also a galvanometer (something that measures amount and direction of electric flow) that measures the direction of electrical flow between the two sides of the diamond. When the flow is balanced, or a net flow of zero, the resistance of the unknown side of the diamond is known. That is the side, remember, that is attached to our strain gauge, and it is that resistance that is proportional to our pressure we are measuring by our strain gauge. The diagram in Figure 18-1 will help.
Figure 18-1 Schematic of a Wheatstone bridge. The value of resistors R1 and R2 are known. Rx is the unknown resistance caused by the action of the pressure waveform on the transducer diaphragm. Rv is a resistor whose resistance is varied until there is no net flow through the galvanometer, G. When R1 R2 is equal to Rv Rx, the bridge is balanced, and the resistance value is converted into a hemodynamic pressure value by the monitor.
The important thing to remember is that the transducer changes the pressure that we are measuring in the patient (arterial, CVP, and so on) and changes it into electrical energy that is read by our monitor by use of measuring resistance through a Wheatstone bridge.
The electrical adaptor of the transducer attaches to a cable that goes to our monitoring system either directly or through a shelf of “boxes” that is ultimately attached to the monitor. There is an amplifier that increases the intensity of the reading coming from the transducer, and then there is various hardware and software that gives us the nice tracing with numbers that we see on the screen.
So now we’re all done with the physics part of this chapter, right? We are sorry, but no. We need to discuss the physics of the patient part of the transducer, too.
Physics of the Transducer–Patient Connection
At first, it might not seem that there is any physics relating to the section of the pressure transducer between the patient’s vascular system and the electrical stuff. But there is, and the physics of the patient connection involves things that we can control.
Damping and Underdamping
The physics of the patient side of the transducer has to do with the concept of damping, its counterpart underdamping, and the resonance of the system. These concepts are often seen on keyword lists, and you are expected to know about them for your board examinations.
Damping (sometimes called overdamping) is a term that relates to anything that decreases the quality or intensity of the pressure waveform energy before it gets to the transducer diaphragm. Underdamping is a term that relates to anything that exaggerates the quality or intensity of the pressure waveform on its way to the transducer. There are more things that cause damping (or overdamping) than things that cause underdamping. We will discuss damping and underdamping as they relate to each part or factor of the patient side of the transducer.
Tubing The initial thing you may have noticed when you were hooking up your first art line was that the tubing is different than regular IV tubing. Because it is different, there must be a reason it is different, right?
Pressure transducers come with high-pressure tubing. The tubing is thicker, stiffer, and less compliant than an intravenous (IV) setup. This is because regular IV tubing would interfere with accurate pressure readings because it would absorb some of the energy of the pressure waveform and cause damping of the waveform. The waveform that was generated would be less in intensity, especially for the higher or systolic value. This wouldn’t be too much of a problem if measuring CVP, but it would be a problem for pulmonary artery pressure monitoring and especially systemic arterial monitoring.
Tubing Length The length of tubing for a transducer as it comes out of the package is around 4 feet. That length is long enough to reach from the catheter site to where you have placed the transducer. Although the length is not written in stone, it is a compromise between having enough tubing to work with but not enough to cause significant damping. We all have probably had to add high-pressure extension tubing to a transducer setup to meet our needs in the OR, but you should remember that additional tubing length causes damping even if you use high-pressure tubing. Likewise, less tubing than normal can cause underdamping.
Tubing length affects damping in two ways. The first way is that with excess tubing, there is more surface area of tubing to absorb the waveform traveling inside it. Second, more tubing increases the mass of fluid that the waveform must move in order to propagate its energy to the transducer diaphragm. Both of these contribute to damping of the waveform.
Fluid Path The fluid path is the medium in which the waveform energy travels. Fluid is relatively incompressible compared with air. As an example, fill a 10-cc syringe with air and attach it to a closed stopcock. You will be able to push down on the plunger to a certain degree, compressing the air (gas) inside it. Try again with the syringe full of saline instead of air. You will not be able to compress the plunger at all.
In a similar fashion, bubbles of air in the fluid path will be compressed by the waveform energy traveling through the fluid. Even though it seems like this loss of energy would be so small as to not influence the pressure reading, it is enough to cause damping of the waveform even when there are very small bubbles present. Conversely, if a transducer system is underdamped, an old trick was to place a small amount of air in the line to purposely dampen the waveform. It was a potentially dangerous practice, however, because if you flushed the line without removing the bubble, it would go right into the patient.
Viscosity of the fluid itself affects accuracy of the waveform. The preferred fluid for transducers is normal saline (heparinized or not). An increase in fluid path viscosity, like in an unflushed line full of blood, will dampen the waveform tracing. Although blood versus saline in a line probably will not make a significant clinical difference, blood left in a line can form a clot, usually in the catheter itself or at the tip of the catheter, which certainly will dampen the waveform.
To guard against clot formation, transducer kits are made so that when the IV bag is placed under pressure, a small amount of IV fluid infuses into the catheter each hour. This amount is around 3 cc/hr. It is important to remember to make sure the IV bag is under pressure or the catheter will clot. It is also important, like when anytime you place a bag under pressure, that you take all of the air out of the bag. If the bag or drip chamber to be upside down when you flushed the system, air could be introduced, not just into the tubing but potentially into the patient, which is a bad thing, of course.
Flushing System Every transducer comes with some form of flushing system. Some may be similar to a button that is pressed, and some may be a “pigtail” that is pulled to open the flushing system from the pressurized IV bag. Care must be taken when flushing. Our inclination is to flush until we see the line clear all the way into the patient. However, this is not a good idea. There are cases of retrograde arterial line flow from prolonged flushing. The flush goes proximally back into central circulation. For instance, if you have a radial arterial line and flush too vigorously, the flush fluid can travel up the arm, into the aortic arch, and then perhaps up the carotids to the brain. This is a bad thing, especially if there is a tiny bubble or clot traveling along with the flush. When you flush a transducer, you should flush for only a second or so, repeatedly, until the line is clear. It is believed that a short flush will not travel in a retrograde direction long enough to reach the central circulation.
Frequency Things have a natural frequency. You may have heard that soldiers are supposed to walk, not march, over bridges so the frequency of the synchronized footsteps will not approach the natural frequency of the bridge. If the soldiers were marching in step, the bridge would begin to sway in rhythm to the steps and could possibly collapse. If you have ever gone over a swinging footbridge, you probably know what we mean. The more forcefully you step, the more the bridge swings and sways, and if you kept stomping as you walked, it would be hard to keep standing because the bridge would be moving so much.
If you drop a tennis ball on the floor, it will bounce several times before coming to rest. In addition, each bounce will not be as high as the preceding one. We can say that the natural frequency of the tennis ball in this case is how quickly the ball bounces. Another concept in this example is the damping coefficient, which has to do with how quickly the ball comes to rest. We will discuss natural frequency to an extent here, but if you want to learn more about the damping coefficient, get out your old physics textbook and turn to the classical mechanics section.
Transducers also have a natural frequency. It is supposed to be anywhere from 10 to 15 Hertz (Hz). You would think that frequency of the patient’s intravascular waveform will correspond to whatever the heart rate is; 60 beats/min would be 1 Hz, 90 beats/min would be 1.5 Hz, 120 beats/min would be 2 Hz, and so forth. But that is not really the case because the waveform is not a true simple sine wave. Rather, the waveform itself is a combination of more than one sine wave. That is why it looks the way it does; for instance, the dicrotic notch is one sine wave added to the systolic sine wave and so on, so we get the waveform shape we are used to seeing.
These different component waves have their own frequencies. This is why sometimes you see “ringing,” or an exaggerated systolic component of an arterial waveform. Such exaggerations of the systolic or diastolic curve influence what the monitor reads out as being the systolic or diastolic pressure; it will overestimate both systolic and diastolic pressures if there is too much ringing.
So how can one tell if your transducer system is too sensitive? One way is called a fast flush, or step test. You pull the flush for a second or so and make a square wave on your screen. Then release the flush quickly and see how quickly and smoothly the waveform goes back to normal. If a system is dampened, the waveform will not return to the zero line and will take its time going down. If it is underdamped, the waveform will not only return to zero, but also overshoot zero and show extra squiggles as the waveform calms back down again. If the system is okay, after you release the flush, the waveform will go back to or go slightly past zero quickly and show four or five squiggles before it calms down.
It is possible to calculate the natural frequency (fn) of your transducer setup. You record a paper strip of your waveform tracing and then divide the paper speed by the distance between oscillations after the fast flush.
Although this is the correct and proper procedure, who really has time to do this during a case? In our opinion, it is better to learn the three general response patterns (normal, overdamped, and underdamped) by morphology and compare mentally with the fast flush arterial line result when performing an anesthetic with an arterial line (Figure 18-2).
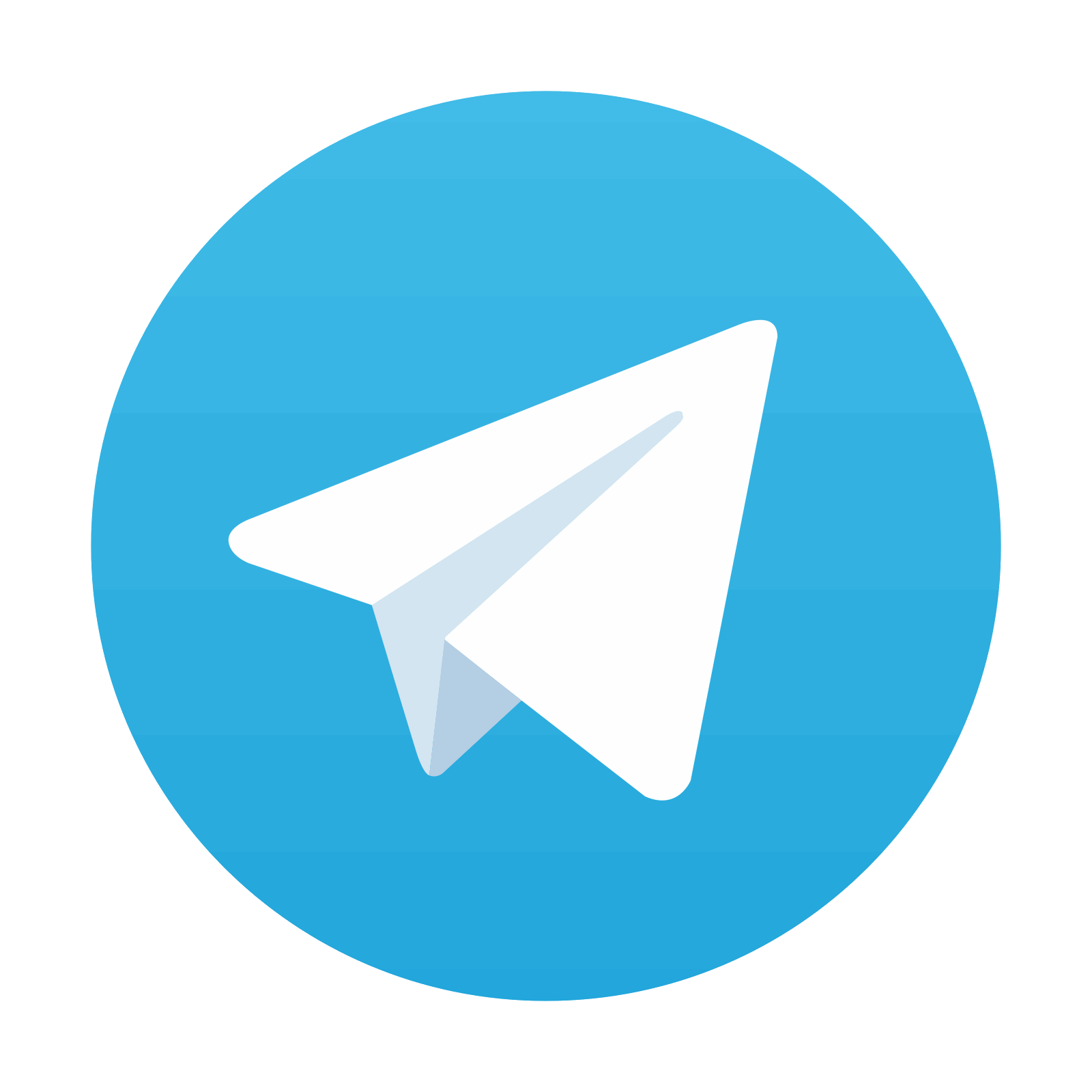
Stay updated, free articles. Join our Telegram channel

Full access? Get Clinical Tree
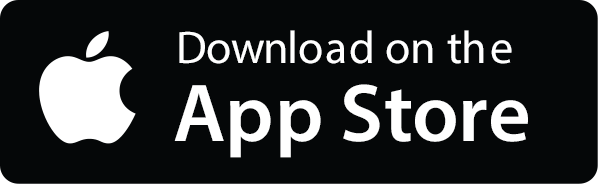
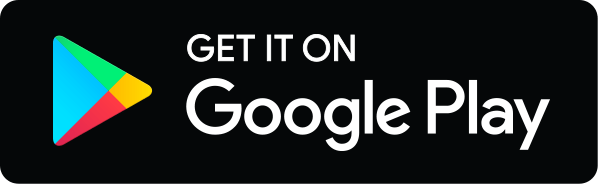