INTRODUCTION AND EPIDEMIOLOGY
Traumatic brain injury is brain function impairment that results from external force.1 The clinical manifestations represent a broad constellation of symptoms from brief confusion to coma, severe disability, and/or death. The underlying pathology ranges from temporary shifts in cellular ionic concentrations to permanent structural damage.
Traumatic brain injury (TBI) is classified as mild, moderate, and severe based on the Glasgow Coma Scale (GCS) score. Over 80% of TBI is defined as mild (GCS 14 to 15) (mTBI) and is often called “concussion.”2 The label of mild, however, is a misnomer. mTBI may lead to significant, debilitating short- and long-term sequelae. Moderate TBI (GCS 9 to 13) accounts for approximately 10% of head injuries. Mortality rates for patients with isolated moderate TBI are <20%, but long-term disability can be higher. Overall, 40% of patients with moderate TBI have an abnormal finding on CT scan, and 8% will require neurosurgical intervention. In severe TBI (GCS 3 to 8), mortality rate approaches 40%, with most deaths occurring in the first 48 hours after injury. Fewer than 10% of patients with severe TBI experience good recovery.2,3
The prevalence of TBI is twice as high in males as in females. Distribution of age at injury is trimodal, with peaks at 0 to 4 years, 15 to 24 years, and >75 years of age. Mortality rate increases with age at time of injury.4,5 Motor vehicle collisions are the primary cause of blunt head injury in young adults and children, and falls are more common in the elderly.2 TBI has been called the “signature injury” of the conflicts in Iraq and Afghanistan.6
PATHOPHYSIOLOGY
Autoregulation, cerebral perfusion pressure (CPP), mean arterial pressure (MAP), and intracranial pressure (ICP) are interrelated factors that affect cerebral blood flow (Table 257-1). Under normal circumstances, autoregulation regulates local cerebral blood flow to maintain equilibrium between oxygen delivery and metabolism.7 Other systemic factors, such as hypertension, hypocarbia, and alkalosis, can affect cerebral blood flow by causing vasoconstriction.
Under normal situations, autoregulation can adjust to CPPs from 50 to 150 mm Hg to maintain local cellular oxygen demands and regional cerebral blood flow. In brain injury, autoregulation is often impaired, so even modest drops in blood pressure can decrease brain perfusion and result in cellular hypoxia. A CPP <60 mm Hg is considered the lower limit of autoregulation in humans, below which local control of cerebral blood flow cannot be adjusted to maintain flow adequate for function.8 Traumatic hypotension leads to ischemia within low flow regions of the injured brain, so aggressive fluid resuscitation may be required to prevent hypotension and secondary brain injury. In the absence of an ICP monitor, it is important to maintain a MAP of ≥80 mm Hg, because low blood pressure in the setting of elevated ICP will result in a low CPP and brain injury.
The cranium is an enclosed space with a fixed volume. Any changes to the volume of the intracranial contents (such as bleeding) affect the ICP, and an increase in ICP can decrease the CPP. ICP is determined by the volume of the three intracranial compartments: the brain parenchyma (<1300 mL in the adult), cerebrospinal fluid (100 to 150 mL), and intravascular blood (100 to 150 mL). When one compartment expands, there is a compensatory reduction in the volume of another, and/or the baseline ICP will increase (Figure 257-1). Elevations in ICP are life threatening and may lead to a phenomenon known as the Cushing reflex (hypertension, bradycardia, and respiratory irregularity). Hypertension is an attempt to maintain cerebral perfusion. Normal values for ICP vary with age (Table 257-2).
FIGURE 257-1.
Pressure–volume relationship in brain injury. Normal cerebral blood flow autoregulation curve and the abnormal curve with traumatic brain injury (TBI). Normal autoregulatory control (blue line) maintains a relatively constant cerebral blood flow over a broad range of mean arterial pressure (MAP).9 Loss of autoregulation results in a more linear relationship between cerebral blood flow (CBF) and MAP. Elevated intracranial pressure (ICP) can dramatically decrease CBF when autoregulation is impaired (inflection point of red line). Increases in ICP may result in a net loss in CBF.
The initial insult associated with moderate and severe TBI imparts mechanical forces that produce high levels of direct damage and strain to the brain parenchyma. The primary injuries include contusions (bruises to brain parenchyma), hematomas (subdural, epidural, intraparenchymal, intraventricular, and subarachnoid), diffuse axonal injury (stress or damage to axons), direct cellular damage (neurons, axons, and other supportive cells), loss of the blood–brain barrier, disruption of the neurochemical homeostasis, and loss of the electrochemical function.
A wave of secondary damage is unleashed by the impact that results in a series of deleterious cellular and subcellular events (also known as the secondary neurotoxic cascade).10,11 The secondary neurotoxic cascade causes ongoing damage to the brain and ultimately results in a poorer neurologic outcome than might have occurred based on the original mechanism.
The secondary neurotoxic cascade should not be confused with the term secondary insults, a term used in the clinical literature to describe conditions or circumstances (e.g., hypotension, hypoxemia, hyperglycemia) that accelerate neurotoxic damage and worsen long-term outcome.12,13 Mediation of secondary insults reduces morbidity and mortality and is discussed in the treatment section.
The secondary neurotoxic cascade is a massive release of neurotransmitters, such as glutamate, into the presynaptic space, with activation of N-methyl-D-aspartate, α-amino-3-hydroxy-5-methyl-4-isoxazole propionic acid, and other receptors.10 Ionic shifts activate cytoplasmic and nuclear enzymes, induce mitochondrial damage, and lead to cell death and necrosis.10,14,15 Proinflammatory cytokines and other enzymes are released in an attempt to clean and repair the damage. Secondary injury, however, is indiscriminant and produces extensive neuronal loss. Additionally, many survivable cells undergo apoptosis, or programmed cell death, during secondary injury.16 Apoptosis has been reported to occur longer than a year after injury.16,17
Brain edema results from two distinct processes and can be fatal in TBI.18 Cellular swelling, or cytotoxic edema, results from large ionic shifts and the loss of cellular membrane integrity from mitochondrial damage (loss of adenosine triphosphate, ion pump productivity, and increased free radical production). Extracellular edema results from direct damage to, or the breakdown of, the blood–brain barrier, ionic shifts, and alteration of water exchange mechanisms (e.g., aquaporins).19,20 As intracellular and extracellular water content rises, the brain swells and the ICP increases, leading to direct compressive tissue damage, vascular compression-induced ischemia, brain parenchyma herniation, and brain death.
There are four major brain herniation syndromes: uncal transtentorial, central transtentorial, cerebellotonsillar, and upward posterior fossa. The most common is uncal herniation, which occurs when the uncus of the temporal lobe is displaced inferiorly through the medial edge of the tentorium. This is usually caused by an expanding lesion in the temporal lobe or lateral middle fossa. Uncal transtentorial herniation leads to compression of parasympathetic fibers running with the third cranial (oculomotor) nerve, causing an ipsilateral fixed and dilated pupil due to unopposed sympathetic tone. Further herniation compresses the pyramidal tract, which results in contralateral motor paralysis. In some cases, the pupillary changes can be contralateral, whereas the motor changes are ipsilateral.
Central transtentorial herniation is less common and occurs with midline lesions, such as lesions of the frontal or occipital lobes, or vertex. The most prominent symptoms are bilateral pinpoint pupils, bilateral Babinski’s signs, and increased muscle tone. Fixed midpoint pupils follow along with prolonged hyperventilation and decorticate posturing.
Cerebellotonsillar herniation occurs when the cerebellar tonsils herniate through the foramen magnum. This may lead to pinpoint pupils, flaccid paralysis, and sudden death. Upward transtentorial herniation results from a posterior fossa lesion and leads to a conjugate downward gaze with absence of vertical eye movements and pinpoint pupils.
TBI severity is classified using the GCS (Table 257-3). The scale is composed of three components: eye opening (1 to 4 points), verbal response (1 to 5 points), and motor response (1 to 6 points) (Table 257-3). The sum of these components defines the TBI severity classification into severe (GCS score of 3 to 8), moderate (GCS score of 9 to 13), and mild (GCS score of 14 or 15). The motor score independently correlates with outcome, almost as well as the full score.21
4 y to Adult | Child < 4 y | Infant | |
---|---|---|---|
Eye opening | |||
4 | Spontaneous | Spontaneous | Spontaneous |
3 | To speech | To speech | To speech |
2 | To pain | To pain | To pain |
1 | No response | No response | No response |
Verbal response | |||
5 | Alert and oriented | Oriented, social, speaks, interacts | Coos, babbles |
4 | Disoriented conversation | Confused speech, disoriented, consolable, aware | Irritable cry |
3 | Speaking but nonsensical | Inappropriate words, inconsolable, unaware | Cries to pain |
2 | Moans or unintelligible sounds | Incomprehensible, agitated, restless, unaware | Moans to pain |
1 | No response | No response | No response |
Motor response | |||
6 | Follows commands | Normal, spontaneous movements | Normal, spontaneous movements |
5 | Localizes pain | Localizes pain | Withdraws to touch |
4 | Moves or withdraws to pain | Withdraws to pain | Withdraws to pain |
3 | Decorticate flexion | Decorticate flexion | Decorticate flexion |
2 | Decerebrate extension | Decerebrate extension | Decerebrate extension |
1 | No response | No response | No response |
3–15 |
The GCS is an objective measurement of clinical status, correlates with outcome, is a reliable tool for interobserver measurements, and is effective for measuring patient recovery or response to treatment over time. However, the scale has several limitations. It measures behavioral responses, not the underlying pathophysiology. Patients with similar GCS scores may have dramatically different underlying structural injuries and require different clinical interventions (Figure 257-2). It is not as useful as a single acute measure of severity as it is as a tool to measure disease progression over time. The GCS may additionally be affected by drugs, alcohol, medications, paralytics, or ocular injuries. Finally, the scale lacks the granularity necessary to assess mTBI.
CLINICAL FEATURES
The results of history, examination, and diagnostic imaging will allow the distinction into two categories of injury: moderate-severe brain injury and mild brain injury. Treatment and disposition are quite different in the two categories and are detailed below.
Obtain an accurate history from the patient, witnesses, and EMS crews to gain important insight into the mechanism of injury and overall severity of TBI (e.g., height of fall, impact surface condition, damage sustained to vehicle, airbag deployment, seat belt use, history of ejection from the vehicle, or report of fatalities at the scene). Premorbid medical history, medications (especially anticoagulants), drug use, and/or alcohol intoxication are also important in the assessment and treatment of acute TBI. Initial clinical findings and physical exam as reported by EMS are an essential component of triaging and managing TBI. The presence of a focal neurologic deficit, seizures, emesis, or depressed level of consciousness increases concern for underlying brain injury.
Follow Advanced Trauma Life Support principles to perform the trauma-focused examination, with simultaneous lifesaving procedures as needed. Protect the cervical spine during evaluation, treatment, and imaging.
Obtain the GCS. Classify the injury as severe (GCS score of 3 to 8), moderate (GCS score of 9 to 13), or mild (GCS score of 14 or 15). If emergency intubation is necessary, obtain a preintubation GCS and record the patient’s best score.
Determine pupillary response.22 In an unresponsive patient, a single fixed and dilated pupil may indicate an intracranial hematoma with uncal herniation that requires rapid surgical decompression. Bilateral fixed and dilated pupils suggest increased ICP with poor brain perfusion, bilateral uncal herniation, drug effect (such as atropine), or severe hypoxia. Bilateral pinpoint pupils suggest either opiate exposure or central pontine lesion.
Altered motor function can indicate brain, spinal cord, or peripheral nerve injuries. Assess movement in a coma patient by observing the patient’s reaction to noxious stimuli, such as pressure to a nail bed. Decorticate posturing (upper extremity flexion and lower extremity extension) indicates severe intracranial injury above the level of the midbrain. Decerebrate posturing (arm extension and internal rotation with wrist and finger flexion and internal rotation and extension of the lower extremities) indicates a more caudal injury. For completely unresponsive patients, respiratory pattern and eye movements can provide information regarding brainstem function. Remember, do not assess oculovestibular (cold caloric) and oculocephalic (doll’s eyes) responses in a patient under cervical spine precautions.
IMAGING
Individually assess each patient’s mechanism of injury, history, comorbidities, and signs and symptoms when determining the need for CT imaging of the head and cervical spine.
Head CT is exquisitely sensitive to the presence of blood and guides ED management. Do not delay head CT, because expanding hemorrhagic lesions need emergency neurosurgical intervention. Therefore, if the patient is uncooperative or combative, intubation and sedation are often the best options to enable rapid CT imaging. Other means to control agitated patients with TBI include midazolam (1 to 2 milligrams IV) and propofol (20 milligrams every 10 seconds to desired effect).
Several decision rules have been developed to minimize unnecessary head CT imaging.23,24,25,26,27 The guidelines strive to identify patients with surgical emergencies. These studies do not specifically address the relationship between minor CT findings (which may place the patient at risk for the development of seizures), the duration of postconcussive symptoms, and progressive changes on CT during the course of a patient’s evaluation. Adults with mTBI and a GCS score of 14 or 15 will have an intracranial lesion on CT about 15% of the time, but <1% will require neurosurgical intervention.28
The prevalence of cervical fractures in comatose TBI patients is approximately 8%, and an estimated 4% of injuries are missed on the initial assessment of the trauma patient.23 Cervical imaging is a vital component in the care of the brain-injured patient. Perform CT imaging of the cervical spine in patients with altered mental status and who were injured by a mechanism that increases the risk of cervical spine injury. CT is superior to plain radiography in patients with altered mental status and can be performed at the same time as the head CT.
The NEXUS and Canadian Cervical Spine Rules are discussed in detail in chapter 258, “Spine Trauma.”
MRI can detect subtle lesions missed by CT imaging and can better define the extent of contusions. However, MRI may not detect subtle lesions, cannot be performed if the patient is unstable, and is not always available.
Decision rules can guide clinical practice, but each patient must be assessed individually, and none of the rules described below address short- or long-term nonoperative sequelae of TBI. See chapter 110, “Pediatric Trauma,” for a discussion of the role of head CT imaging in children with minor head injury.
The two most commonly used evidence-based clinical decision rules for head CT in adults are the New Orleans Criteria29 and the Canadian CT Head Rule.23 Both rules have been validated and are 100% sensitive in detecting patients who will need neurosurgical intervention, but they have limited specificity (5% versus 37%, respectively). The Canadian CT Head Rule is less sensitive (83%) if intracranial lesion is the defined end point. A negative feature of these two decision rules is that loss of consciousness or amnesia is required as the entry point. Most minor brain injury events do not result in loss of consciousness, and loss of consciousness is not the best predictor of intracranial pathology (Table 257-4). Do not apply these rules to patients taking anticoagulants or antiplatelet agents, or to children, because these variables were not included in the validation studies.
New Orleans Criteria—GCS 15* | Canadian CT Head Rule—GCS 13–15* |
---|---|
Headache | GCS <15 at 2 h |
Vomiting | Suspected open or depressed skull fracture |
Age >60 y | Age ≥65 y |
Intoxication | More than one episode of vomiting |
Persistent antegrade amnesia | Retrograde amnesia >30 min |
Evidence of trauma above the clavicles | Dangerous mechanism (fall >3 ft or struck as pedestrian) |
Seizure | Any sign of basal skull fracture |
Identification of patients who have an intracranial lesion on CT | |
100% sensitive, 5% specific | 83% sensitive, 38% specific |
Identification of patients who will need neurosurgical intervention | |
100% sensitive, 5% specific | 100% sensitive, 37% specific |
The National Institute for Clinical Excellence and the Neurotraumatology Committee of the World Federation of Neurosurgical Societies have evaluated clinical signs and symptoms associated with TBI in adults and adolescents and adults, respectively.27,30 The resultant decision rules for head CT have been applied to large data sets and shown to be relatively sensitive (National Institute for Clinical Excellence: 94% for neurosurgical lesions, 82% for intracranial lesions; Neurotraumatology Committee: 100% for neurosurgical lesions and intracranial injuries). One study evaluated 1101 patients with mTBI who had GCS scores of 14 or 15; approximately 2% of these patients without loss of consciousness had intracranial lesions and 0.6% required surgery (rates similar to patients with loss of consciousness).
One of the most important findings from these studies is the relative significance of certain elements of the history and physical examination. For example, nausea and vomiting after concussion has an odds ratio comparable to that of loss of consciousness for a positive CT finding (Table 257-5). Importantly, the predictive value of individual clinical signs and symptoms differs between adults and children (see chapter 138, Head Injury in Infants and Children).
Smits et al30 | Ibanez et al26 | Fabbri et al27,70 | |
---|---|---|---|
OR (95% CI) | OR (95% CI) | OR (95% CI) | |
Glasgow Coma Scale score of 14 | 2 (1–3) | 7 (4–14) | 19 (14–26) |
Neurologic deficits | 2 (1–3) | 7 (2–25) | 19 (13–28) |
Signs of basilar skull fracture | 14 (8–22) | 11 (6–23) | 10 (6–16) |
Loss of consciousness | 2 (1–3) | 7 (4–11) | 2 (2–3) |
Posttraumatic amnesia | 1.7 (1–2) | 3 (2–5) | 8 (6–12) |
Headache | 1.4 (1–2) | 3 (2–6)* | — |
Vomiting | 3 (2–4) | 4 (2–7) | 5 (3–8) |
Posttraumatic seizure | 3 (1–10) | 2 (0.25–17) | 3 (2–5) |
Intoxication | 1 (0.6–2) | 1 (0.3–3) | — |
Antithrombotics | 2 (1–4) | 4 (3–7) | 8 (3–9) |
Age >65 y | — | 2 (1–3) | 2 (1–3) |
Dangerous mechanism of injury | 2 (1–4) | — | 3 (2–4) |
A summary of the American College of Emergency Physicians recommendations28 is given in Table 257-6. A combination of rules helps identify patients at risk and determine the possible need for a head CT (Tables 257-4, 257-5 and 257-6).
Adults with a Glasgow Coma Scale score of < 15 at the time of evaluation should undergo CT imaging Mild traumatic brain injury with or without loss of consciousness:if one or more of the following is present:
Mild traumatic brain injury with loss of consciousness or amnesia: if one or more of the following is present:
|
TREATMENT
Early appropriate management can have a profound impact on the patient’s final outcome. For patients with moderate to severe head injury, provide stabilization and rapid transport to a facility with experience in the management of brain injury. The most important prehospital interventions are airway and blood pressure management. If the patient needs prehospital intubation, avoid hyperventilation (which causes cerebral vasoconstriction and can negatively affect outcome), and use capnometry to keep PCO2 at 35 to 45 mm Hg. Treat hypotension aggressively. If transport times are short, do not give mannitol or hypertonic saline for elevated ICP. Guidelines for prehospital care are available at http://www.braintrauma.org.
Principles for ED care of moderate/severe brain injury are provided at http://www.braintrauma.org and are discussed in the following section. The primary goals of treatment are to maintain cerebral perfusion and oxygenation by optimizing intravascular volume and ventilation; prevent secondary injury by correcting hypoxia, hypercapnia, hyperglycemia, hyperthermia, anemia, or hypoperfusion; recognize and treat elevated ICP; arrange for neurosurgical intervention to evacuate intracranial mass lesions; and treat other life-threatening injuries.
Systolic blood pressure of <90 mm Hg and hypoxemia (PaO2 <60) are associated with a 150% increase risk in mortality.31
Observe for the signs/symptoms of elevated ICP: change in mental status, pupillary irregularities, focal neurologic deficits, decerebrate or decorticate posturing, or CT pathology. Some CT signs of intracranial hypertension are attenuation of the visibility of sulci and gyri, because the brain is compressed against the skull; compressed lateral ventricles; and poor grey/white matter distinction. Papilledema may not be evident if pressure rises rapidly. Sedation and analgesia may decrease baseline ICP and prevent transient rises in ICP from agitation, coughing, or gagging from the endotracheal tube. Prevent and control seizure activity.
Treat hypotension, hypoxemia, hypercarbia, and hyperglycemia. A single occurrence of hypotension and hypoxia after brain injury is associated with a 150% increase in mortality.22 TBI is progressive, so appropriate early management will have a greater impact on outcome than treatments initiated after neuronal cell death and the development of secondary injury, such as cerebral edema. Jointly develop and apply goal-directed protocols with emergency medicine, trauma, neurosurgery, and intensive care teams. An example of early goal-directed therapy is provided in Table 257-7.
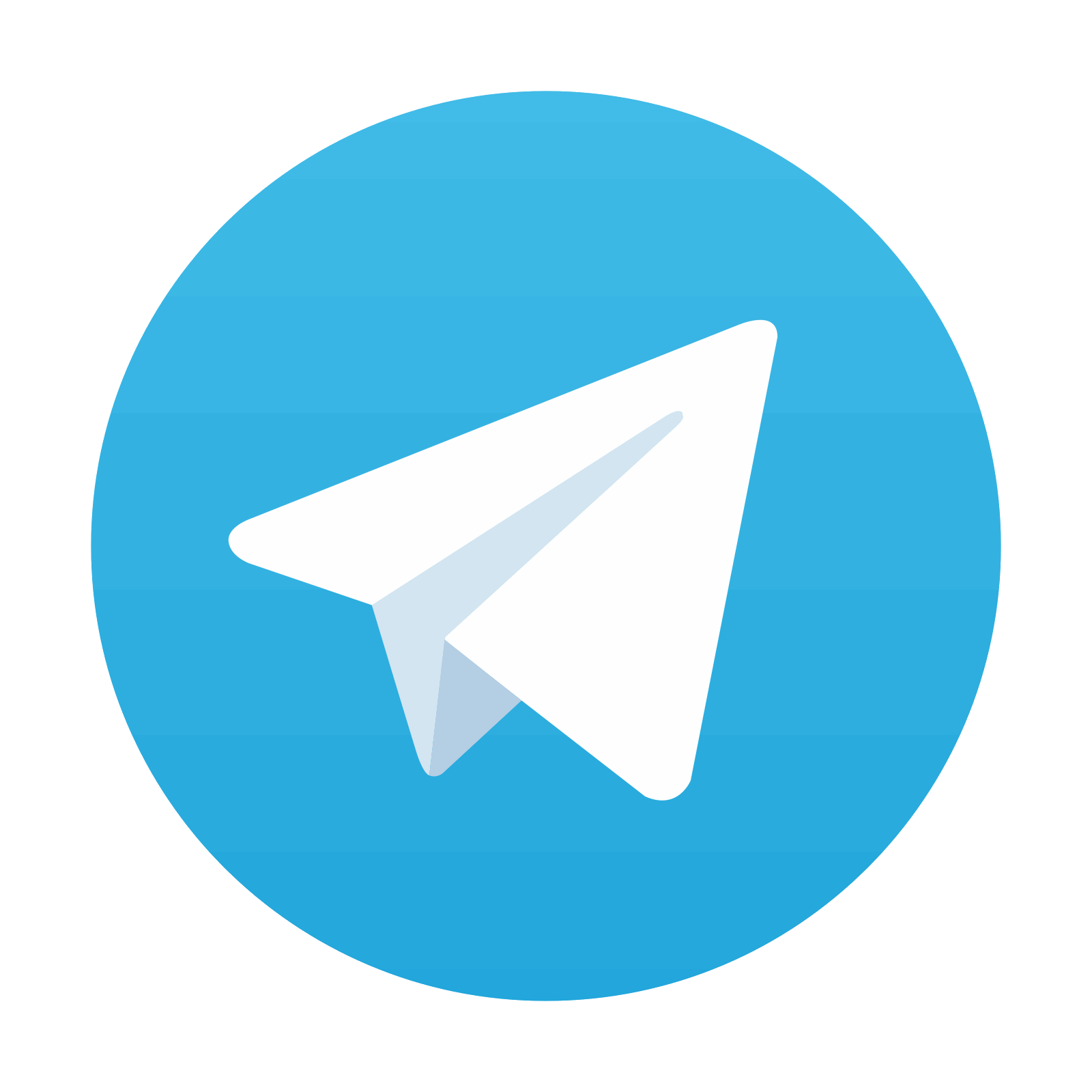
Stay updated, free articles. Join our Telegram channel

Full access? Get Clinical Tree
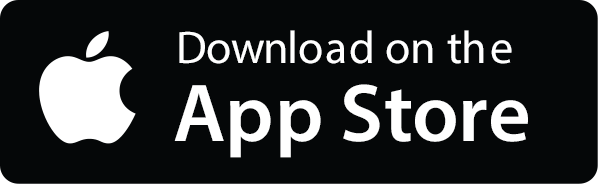
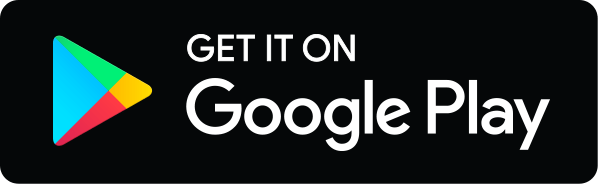