Fluid load versus complications
The challenge for us as clinicians is to keep our patients in the optimal range at all times during the perioperative period. Episodes of hypovolemia or edema if severe can cause major morbidity. More commonly, however, these changes can be subtle, with bowel mucosa ischemia or edema causing gastrointestinal tract dysfunction and prolonged postoperative ileus, with resultant inability to tolerate a normal diet, and increased length of hospital stay.[13]
Traditional monitoring techniques are not useful to accurately detect and optimize the volume status of patients. Healthy volunteers can lose 25% of their blood volume without any discernible change in heart rate or blood pressure, whilst at the same time advanced monitors show a significant reduction in stroke volume and gastric intramucosal pH, implying a degree of ischemia.[14] Central venous pressure (CVP) monitoring has also been shown to be a poor predictor of volume responsiveness.[15] A recent systematic review showed that CVP is not able to identify which patients need more fluid, and concluded that CVP should no longer be routinely measured in the ICU, operating room, or emergency department.[16]
Advanced monitors in GDT can be used to measure CO and SV non-invasively, and thereby allow the clinician to use fluid challenges to achieve SV optimization (see Figure 15.2). When a patient is hypo-volemic and on the steep part of the Starling curve, an intravenous fluid challenge (VC1 in Figure 15.2) will lead to a greater than 10% increase in SV. This patient has “recruitable” SV, and is in a fluid-responsive state. Fluid loading, typically with 250 ml boluses of intravenous colloid, should continue until the increase in SV is less than 10% when the patient has reached the flat part of the Starling curve. This “Frank–Starling fluid challenge” provides a sophisticated method of titrating intravenous fluids to complex patients.
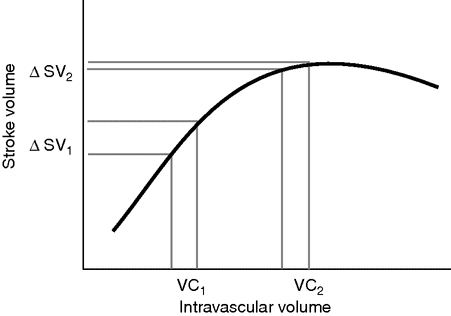
Frank–Starling-based stroke volume optimization.
A crucial difference from the earlier Shoemaker concept for optimization is that the present approach is individualized to optimize flow-related parameters such as SV within the individual’s cardiac capacity, as opposed to using predetermined supraphysiological goals.
Esophageal Doppler
There are a number of technologies that can be used for GDT. The most widely studied is undoubtedly the Esophageal Doppler Monitor (EDM, Deltex Medical, Chichester, UK). The Doppler probe is placed in the esophagus and focused at the descending thoracic aorta, where it uses the Doppler principle to measure blood flow and produce a waveform (Figure 15.3). This is then converted to SV using a nomogram of height, weight, and age to estimate the cross-sectional area of the descending aorta. EDM-derived SV measurements have been well validated in different environments and clinical scenarios.[17–22]
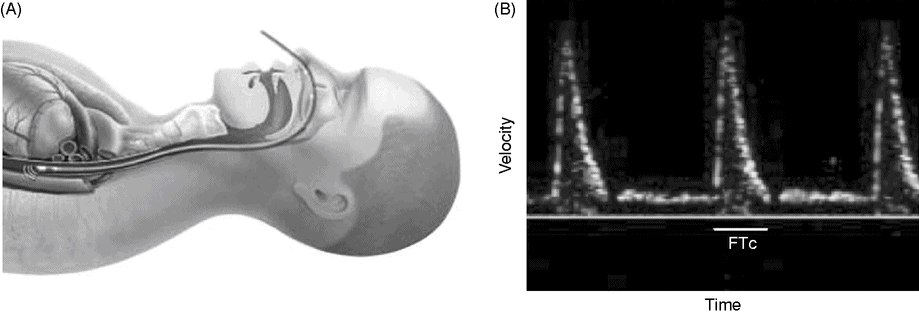
Esophageal Doppler. A: Schematic representation of esophageal Doppler probe in a patient, demonstrating the close relation between esophagus and descending thoracic aorta. B: Characteristic velocity waveform obtained in the descending aorta. FTc: corrected flow time.
Another useful measurement is the corrected flow time (FTc), which is the width of the waveform, or length of systole in ms, corrected to a heart rate of 60. An FTc <350 ms is an additional indicator of recruitable SV.
There are a number of studies that show improved outcomes with EDM-guided fluid optimization, as demonstrated by a faster return in gastrointestinal function, a reduction in postoperative complications and reduced length of stay. Five studies were in a major general/colorectal study population,[23–27], two in cardiac surgery,[28,29], and two in patients scheduled for repair of fractured neck of femur [30,31] (see Table 15.1).
Summary of perioperative esophageal Doppler monitor-guided GDT studies
Reference | Surgical group | Patients (n) | Outcome |
---|---|---|---|
Mythen and Webb (1995) [28] | Cardiac | 60 | ⇓ gastric acidosis in GDT ⇓ complications in GDT ⇓ LOS (3.5 days) in GDT |
Sinclair et al. (1997) [30] | Neck of femur fracture | 40 | ⇓ time FFD (5 days) in GDT ⇓ LOS (8 days) in GDT |
Conway et al. (2002) [23] | Major bowel | 57 | ⇑ ICU admissions in control, no difference in LOS |
Gan et al. (2002) [24] | Major general | 100 | ⇑ PONV in control ⇓ time to tolerating oral intake in GDT ⇓ LOS (2 days) in GDT |
Venn et al. (2002) [31] | Neck of femur fracture | 90 | ⇓ time FFD (6.2 days) in GDT (vs. control) ⇓ time FFD (3.9 days) in CVP (vs. control) |
McKendry et al. (2004) [29] | Cardiac surgery | 174 | ⇓ LOS (2.5 days) in GDT, no difference in complications |
Wakeling et al. (2005) [25] | Colorectal | 128 | ⇓ morbidity (GI and overall) in GDT ⇓ time to full diet (1 day) in GDT ⇓ LOS (1.5 days) in GDT |
Noblett et al. (2006) [26] | Colorectal | 108 | ⇓ morbidity in GDT ⇓ time to tolerating diet (2 days) in GDT ⇓ time FFD (3 days) in GDT ⇓ LOS (2 days) in GDT |
Senagore et al. (2009) [27] | Laparoscopic colorectal | 64 | no difference in LOS no difference in complications |
LOS, length of stay; FFD, fit for discharge; GI, gastrointestinal; PONV, postoperative nausea and vomiting.
All of these studies used a 10% algorithm to optimize SV, often combined with assessment of FTc to predict fluid responsiveness. Although there are small differences between the studies, a typical algorithm is shown in Figure 15.4.
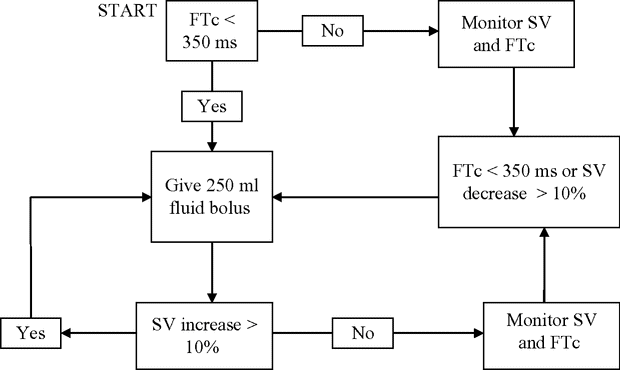
A typical combined FTc and SV optimization algorithm.
The major limitation of the EDM is the occasional need for repositioning of the probe to optimize the signal, which can be time-consuming. There is a learning curve for positioning the probe, and as such it is somewhat user-dependent. The use of electrocautery can also interfere with the signal.
Nevertheless, the evidence base behind its use is relatively strong, and its incorporation into Enhanced Recovery after Surgery (ERAS) programs is currently a strong driving force for increased interest.[32] Recently the Center for Medicare Services in the USA have reviewed the literature and supported a professional fee being paid to clinicians using EDM-guided perioperative volume optimization.[33] The Centre for Evidence-Based Purchasing division of the NHS Purchasing and Supply Agency in the UK has also recommended the EDM.[34]
Arterial pressure waveform analysis
The other major monitoring technique used in GDT is arterial pressure waveform analysis. There are several monitors available that are able to analyze the arterial waveform to calculate SV and CO, and therefore use the “10% algorithm” in response to a fluid challenge.
Arterial waveform analysis is also able to derive dynamic parameters of fluid responsiveness, based on cardiopulmonary interactions, such as stroke volume variation (SVV) and pulse pressure variation (PPV). These dynamic variables are superior to traditional static indices such as CVP in predicting volume responsiveness in mechanically ventilated patients.[35]
The physiology behind SVV and PPV is relatively simple. Positive-pressure ventilation induces cyclical changes in the loading conditions of the right ventricle, with a reduction in preload during mechanical insufflation. This will lead to cyclical changes in SV. If the ventricle is operating on the steep part of the Starling curve, the magnitude of the change in SV and blood pressure will be greater, and will manifest itself as a characteristic “swing” in the arterial line pressure with respiration (Figure 15.5).
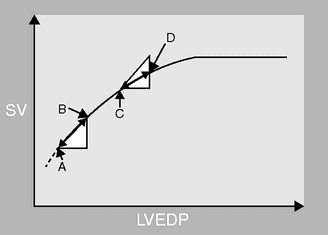
A Starling curve of left ventricular stroke volume (SV) against left ventricular end-diastolic pressure (LVEDP) demonstrating the change in SV that occurs with positive-pressure ventilation. The starting position on the curve determines the magnitude of the change in SV, and hence the SV variation. If the patient is hypovolemic the LVEDP will cycle between A and B, with respiration causing a cyclical change in SV and a swing in the arterial line. If the patient is euvolemic LVEDP will cycle between C and D, causing a much smaller change in SV.
PPV and SVV represent “virtual” preload challenges occurring during each respiratory cycle in ventilated patients. There is no need to administer fluid to predict responders, with SVV or PPV greater than 10% accurately predicting a positive response to a fluid challenge.[36] As these metrics predict responders with more accuracy than CVP or pulmonary artery occlusion pressure (PAOP), there is less need for invasive central lines.
Targeting a PPV or SVV <10–13% has been shown to improve postoperative outcomes. At least eight randomized controlled trials have demonstrated that hemodynamic strategies based on PPV or SVV monitoring allow a significant reduction in post-surgical complications and hospital length of stay after major abdominal surgery.[37]
There are some important limitations to the use of the dynamic variables. The main limitation is that patients should be fully mechanically ventilated without any spontaneous breathing, and with a regular R:R interval. Fortunately in the operating room this is generally the case; less so in the intensive care unit. For optimal results the tidal volume should be 8 ml/kg with “normal” intrathoracic and intra-abdominal pressures. For example, during laparoscopic surgery after insufflation of the pneumoperitoneum, there will be an elevation of intra-abdominal pressure (IAP) that will decrease chest wall compliance, and consequently SVV and PPV will increase independently of changes in blood volume.[38,39] However, when IAP <15 mmHg, SVV and PPV still appear to predict fluid responsiveness fairly well, although the threshold may be raised (fluid responsive when PPV >15% rather than 10%).[38,40]
In practice, the limitations of each dynamic index should be taken into consideration and the dynamic variables used in combination with changes in SV to ensure that fluid boluses are given at the appropriate time to improve hemodynamics without any increase in risk.
Other technologies
An increasing number of new technologies that have recently been marketed have the ability to monitor CO non-invasively.
The NICOM (Cheetah Medical, Boston, MA) is a continuous non-invasive CO monitor based on chest bioreactance that is accurate when compared with the PAC.[41,42] It is totally non-invasive, comprising four surface electrodes that are placed across the chest. The NICOM has been shown to perform similarly to the EDM in guiding GDT, with no clinically significant differences in outcomes, and offers increased ease of use as well as fewer missing data points.[43]
The ClearSight system (Edwards Lifesciences, Irvine, CA) is another completely non-invasive method of measuring CO using an inflatable finger cuff. The cuff “clamps” the pulsating finger artery to a constant volume by applying a varying counterpressure equivalent to the arterial pressure. This results in a continuous pressure waveform that resembles an arterial line waveform, reliably tracks blood pressure,[44] and serves as the basis for determining continuous CO. There appears to be good correlation between ClearSight-derived CO and the PAC.[45]
The pleth variability index (PVI) is a similar value to SVV and PPV that is continuously calculated from the pulse oximeter tracing by the non-invasive Masimo monitor (Masimo Corporation, Irvine, CA). PVI-based goal-directed fluid management has been shown to reduce intraoperative and postoperative lactate levels.[46] As is the case with SVV and PVV, one should always bear in mind the same limitations before taking clinical decisions regarding the hemodynamic optimization of patients.
The BioZ (Cardiodynamics Intl. San Diego, CA) uses thoracic bioimpedance, which is less robust.[47] The Aesculon (Osypka Medical, La Jolla, CA) uses electrical velocimetry to interpret the maximal change in thoracic bioimpedance to calculate CO, and has been shown to be accurate.[48] The challenge for manufacturers is to produce not only a well-validated, reliable monitor, but to show an outcome benefit in this increasingly competitive field.
GDT within an Enhanced Recovery program
In the past few years, Enhanced Recovery programs are becoming standard of care for colorectal surgery, and are increasingly being applied to other major surgery.[49] Enhanced Recovery programs have been shown to reduce length of stay for colorectal surgery by 2.5 days, and decrease perioperative complications by 50%.[50] Such programs aim to avoid prolonged preoperative fasting, so that the patient is euvolemic upon arrival to the OR, potentially making intraoperative fluid management easier.
Single-center studies of GDT within an ERAS program have failed to find the same benefit on postoperative outcomes as the early studies.[51,52] This is perhaps not surprising, as care within the control group has significantly improved, making it harder to observe a difference versus GDT.
The multicenter POEMAS study randomized 142 patients undergoing major abdominal surgery within an Enhanced Recovery pathway to GDT using the NICOM or control, and found no difference in the incidence of overall complications or length of stay.[53]
In contrast, the larger multi-center OPTIMISE study focused on GDT using the LiDCO rapid system (LiDCO Ltd, Cambridge, UK) on 734 high-risk patient undergoing major abdominal surgery.[54] A significant proportion of these patients were managed within an ERAS pathway. In this high-risk population there was a non-significant trend towards decreased complications (36.6% vs. 43.4%, p = 0.07) and 180-day mortality (7.7% vs. 11.6%, p = 0.08) in the GDT group compared with usual care. The study was underpowered, as the complication rate in both groups was less than expected. However, inclusion of these data in an updated meta-analysis indicated that the intervention was associated with a reduction in complication rates.[54]
Therefore, whilst ERAS programs may have raised the threshold for benefit, current evidence suggests that there will still be many patients, whether expected or unexpected, for whom SV optimization will be beneficial. Many patients who are undergoing major surgery outside of ERAS pathways, such as vascular surgery, will also benefit from GDT.
Ultimately the need for GDT is specific to the patient, surgeon, procedure, and institution. Each institution needs to look at its outcomes and benchmark data for the benefitting population against other institutions before making an informed decision about appropriate implementation of GDT. This data should include length of stay, readmission rate, mortality, and costs. All patients should have an individualized plan for fluid management rather than a formulaic approach based on body weight and duration of surgical exposure. For patients undergoing colorectal surgery with limited blood loss within an ERAS pathway, minimal fluid may be required, and GDT may not offer additional benefits.[55] However, current evidence suggests that for the majority of patients undergoing major surgery, GDT remains the technique of choice for perioperative fluid and hemodynamic optimization.[56]
Which fluid should I use?
Although the need for maintenance fluids has recently been questioned, most GDT algorithms use a background infusion of a balanced crystalloid solution at 1–3 ml per kg per hour based on ideal body weight.
In addition, most GDT algorithms in the literature have used a colloid solution for fluid boluses. This is based on the principle that in the setting of hypovolemia a colloid will restore blood pressure and therefore organ perfusion faster and with less volume.[57] However, in clinical practice the superiority of colloids over crystalloids remains unresolved.[58] A recent study comparing crystalloids and colloids for fluid boluses within a GDT algorithm found no difference in the primary outcome of postoperative gastrointestinal morbidity.[59] Therefore, in practice, a combination of crystalloids and colloids are used for fluid challenges, with colloids typically reserved for definitive evidence of significant intravascular hypo-volemia with blood loss.
Conclusion
As the population ages, the number of patients requiring high-risk non-cardiac surgery is only going to increase. The concept of individualized GDT for these patients seems to improve outcomes by ensuring adequate tissue perfusion at all times perioperatively. The underlying mechanism behind the success of GDT is related to the optimization of DO2 to the tissues. This avoids an oxygen debt, which can cause mitochondrial damage and organ dysfunction.
Many authors have described how the use of GDT can reduce morbidity and length of stay in high-risk surgical patients, although most of the studies have been performed on small sample sizes from single centers. Two recent meta-analyses focusing on renal function [60] and gastrointestinal function [61] have also showed that surgical patients receiving perioperative GDT are at decreased risk of renal and gastrointestinal impairment, which account for a significant proportion of postoperative morbidity. The largest study of GDT to date is the OPTIMISE study which showed a trend towards a reduction in complications that did not reach statistical significance. A larger OPTIMISE–2 study is planned.
Perioperative GDT has not become standard of care for a variety of reasons. It remains a challenge to implement GDT because of the significant commitment and resources needed. However, with an increasing number of studies being published on the clinical utility of non-invasive hemodynamic monitoring, the use of GDT should continue to gain popularity.
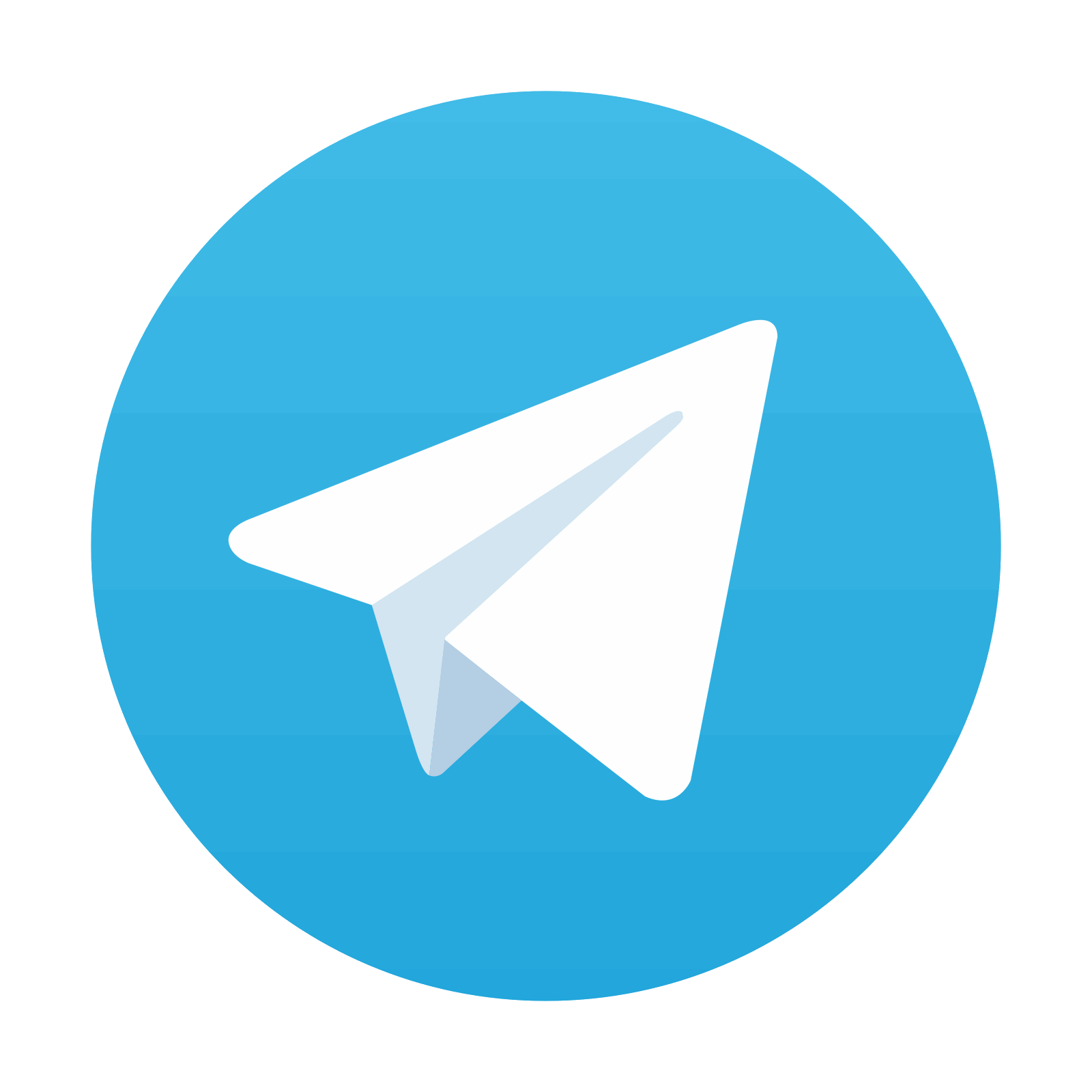
Stay updated, free articles. Join our Telegram channel

Full access? Get Clinical Tree
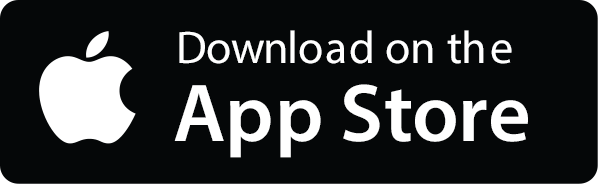
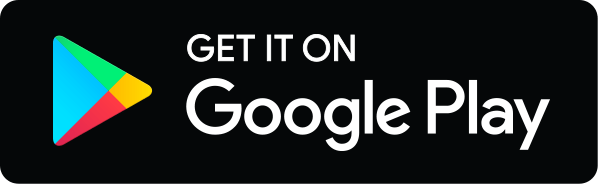