Acknowledgments
The authors gratefully acknowledge the previous contributions of James M. Madsen.
Historical perspective
Biological and chemical weapons have been used throughout history. For millennia, indigenous South American peoples deliberately used plant-derived arrow poisons such as curare and toxins from poison dart frogs, although these preparations were used mainly for hunting. Similar toxins were used in Africa. The ancient Greeks, for whom toxikon meant “arrow poison,” tipped arrows with winter aconite, and this practice continued into medieval Europe, persisting into the seventeenth century in Spain and Portugal. Soldiers in India used smoke screens, incendiary weapons, and toxic fumes as early as 2000 bce , and the Sung Dynasty in China employed a wide variety of arsenical smokes and other poisons in battle. The military use of toxins dates from at least the sixth century bce , when Assyrian soldiers poisoned enemy wells with ergot-contaminated rye. In 423 bce , during the Peloponnesian War, Thracian allies of Sparta captured the Athenian fort at Delium by using a long tube and bellows to blow poisonous smoke from coals, sulfur, and pitch into the fort. Greek fire (likely composed of rosin, sulfur, pitch, naphtha, lime, and saltpeter) was invented in the seventh century ce and proved to be a very effective naval weapon. Various poisons saw battlefield use during medieval times, and the use of poisons for murder (including assassinations) became widespread. Other examples before the twentieth century include the contamination of water by dumping the corpses of dead humans or animals into wells, the use of snakes and other creatures as poisonous vectors, and occasionally, fomites to transmit infections such as smallpox to unsuspecting victims. This latter technique was used with remarkable success during the French and Indian War (1754-1767), when Sir Jeffrey Amherst was alleged to have given “gifts” (blankets) harboring the pus and scabs from smallpox victims to unsuspecting Native Americans. The Indians possessed no immunity against smallpox and thus experienced very high rates of infection and mortality as smallpox swept through the local tribes.
During the late nineteenth and early twentieth centuries, the science and technology necessary for the development of sophisticated biological and chemical weapons proceeded apace. World War I saw the first large-scale use of “poison gas,” including lacrimators, chlorine, phosgene, arsenicals, cyanide, and sulfur mustard. By the end of the war, nearly one in every three rounds was a chemical munition. Dr. Shiro Ishii and other Japanese scientists in the infamous Unit 731 worked on the weaponization of anthrax, plague, smallpox, and tetrodotoxin as well as a variety of chemical agents during World War II. There are even suspicions that the bomb used in the assassination of Reinhard Heydrich in Czechoslovakia in 1942 contained botulinum toxin. After World War II, ricin was used as an injectable assassination weapon, and in the 1970s and 1980s T-2 toxin, a trichothecene mycotoxin, was alleged to have been the toxic component of the “yellow rain” employed against H’Mong refugees from Laos. More recently, Iraq and Iran both used chemical weapons against each other in the Iran-Iraq War of the 1980s, and Iraq had a weapons program that included the development of sulfur mustard, nerve agents, “Agent 15” (an anticholinergic incapacitating agent), botulinum toxin, epsilon toxin from Clostridium perfringens , and aflatoxin. Militia groups in the United States and terrorist groups throughout the world have used ricin for political purposes.
American scientists started developing chemical weapons as a response to the use of chemical warfare in Europe during World War I and conducted both offensive and defensive research on biological and chemical weapons. However, in 1969 the United States unilaterally renounced the first use of chemical agents, halted chemical-agent production, and terminated its offensive biological weapons program.
In 1972 the Biological Weapons and Toxins Convention was created; it was signed by representatives from 104 nations, including the United States (which ratified the Convention in 1975), the Soviet Union, and Iraq, although many signatories did not consider toxins to be biological weapons and did not consider the treaty binding on toxin use. Since that time, at least 140 nations have either signed or ratified this treaty. However, the Soviet Union and Iraq began violating the treaty in short order. In the Soviet Union, weapons scientists stepped up research and development of numerous biological and chemical weapons as part of one of the largest and most comprehensive biological-weapons programs in history. Soviet scientists created large stockpiles of weaponized anthrax, plague, smallpox, tularemia, nerve agent, mustard, and other biological and chemical agents.
In 1979 the world was put on notice of the devastating potential that biological weapons pose to humanity. In that year, a small quantity of weapons-grade anthrax was accidentally released from a manufacturing plant located in the former city of Sverdlovsk (now Yekaterinburg) in Russia, resulting in 77 cases and 66 deaths. Dr. Matthew Meselson, a Harvard scientist, was permitted to study the event many years later and reported the results of his work in a 1979 Science article. Meselson determined that the majority of the deaths had occurred among victims living in a narrow, 4-km-wide band downwind from the plant. Animal deaths were confirmed as far as 30 km downwind. Meselson further concluded that less than 1 g of weapons-grade anthrax had been released from the plant. If his calculations are accurate, weaponized anthrax possesses staggering potential as a biological weapon given its stability, its relative ease of production, and its ability to be dispersed in a clandestine manner over great distances.
In March 1995, after having unsuccessfully attempting to deploy biological agents, members of the Aum Shinri Kyo cult executed a coordinated attack with the nerve agent sarin (GB) on the Tokyo subway system. More than 5500 people sought medical treatment, and a dozen died. The Aum Shinri Kyo had used sarin in Matsumoto 9 months earlier in an attack that had exposed more than 300 people and killed 7 in an attempt to assassinate judges unfavorable to their cause. ,
The anthrax attacks in the fall of 2001 involved the use of letters containing weapons-grade anthrax mailed through the U.S. postal system. Five people died, and 17 became ill with either cutaneous or inhalational anthrax. Buildings contaminated with spores included the Hart Senate Office building and the Brentwood postal facilities in Washington, DC. It cost millions of dollars to rehabilitate these buildings. The anthrax used in the attacks was determined to be extremely potent and could have caused far greater numbers of casualties had it been dispersed more widely. ,
The use of chemical weapons also occurred in recent history. In September 2013 the United Nations (UN) released their investigations on the use of chemical weapons in Syria. The UN concluded that sarin gas was used on August 21, 2013, in the Ghouta area of Damascus against “civilians, including children, on a relatively large scale.” These findings were based on interviews with survivors and other witnesses, documentation of munitions and their components, collection of environmental samples for subsequent analysis, assessment of symptoms of survivors, and collection of hair, urine, and blood samples.
According to Dr. Ken Alibek, former Deputy Director of Biopreparat, the Soviet Union’s nominally civilian medical research institute, Soviet scientists and physicians spent large sums of money and manpower during the 1980s and 1990s developing the most lethal and potent biological weapons known to man. In addition to weaponizing the etiologic agents of anthrax, smallpox, Marburg fever, and others, they created antibiotic-resistant strains of Yersinia pestis (plague), Francisella tularensis , and other pathogens. Furthermore, by applying genetic engineering techniques, the Soviets are also alleged to have created pathogens with novel characteristics and strains of several organisms capable of defeating certain vaccines.
As we enter the biotechnological revolution of the twenty-first century, our understanding of molecular biology, genetics, and biochemistry is exploding. The human genome has been sequenced, and it is now possible to manipulate genes from disparate organisms to create new and novel pathogens. Scientists are also able to synthesize and weaponize a number of different endogenous biological-response modifiers including cytokines, hormones, neurotransmitters, and plasma proteases. But even nature continues to surprise us. New, naturally occurring infections with the potential to cause large-scale human diseases and death continue to emerge at an ever-increasing rate throughout the world, and it is conceivable that these pathogens could also be weaponized by enterprising scientists.
This chapter briefly reviews the future of chemical and biological weapons as we enter this new era of explosive growth in our understanding of the life sciences. We are presented with an extraordinary opportunity to solve a host of human afflictions or to create new classes of biological and chemical weapons that have the capacity to destroy our civilization as we know it today.
Future biological weapons
The appearance of a new or reemerging infectious disease has global implications. During the past 20 years, more than 30 new lethal pathogens have been identified. A classic example of this emerging threat is pandemic influenza. In 1918, as World War I was coming to an end, the Spanish flu struck with devastating consequences. In less than 1 year, this virus was able to circumnavigate the globe and kill an estimated 40 million people. More recently, the emergence of severe acute respiratory syndrome (SARS) in Southeast Asia resulted from a coronavirus that jumped species from animals to humans and rapidly spread to 29 countries in less than 90 days. Finally, the 2014 outbreak of Ebola in Western Africa, still raging as of this writing, is an example of how devastating these agents can be when they emerge in a region previously naïve to them. Novel and dormant infectious agents such as SARS, influenza, and Ebola appear to be emerging or reemerging with increasing frequency and with greater potential for serious consequences. Many factors contribute to the emergence of new diseases: environmental changes, global travel and trade, social upheaval, and genetic changes in infectious agent, host, or vector populations. Once a new disease is introduced into a suitable human population, it often spreads rapidly and has a devastating impact on the medical and public health infrastructure. If the disease is severe, it may lead to social disruption and have a profound economic impact. Outbreaks of emerging or reemerging diseases may be difficult to distinguish from outbreaks resulting from intentional introduction of infectious diseases for nefarious purposes.
As scientists develop more sophisticated laboratory procedures and increase their understanding of molecular biology and the genetic code, the possibility of bioengineering more virulent, antibiotic, and vaccine-resistant pathogens for military or terrorist uses becomes increasingly likely. It is already theoretically possible to synthesize and weaponize certain biological response modifiers (BRMs) as well as to engineer genomic weapons capable of inserting novel DNA into host cells. The potential to cause widespread disease and death with any of these weapons is incalculable and concerning. Scientists and policy makers have begun to address the issue with a robust research agenda to develop medical countermeasures.
Ebola hemorrhagic fever, as of December 4, 2014, had caused 6055 deaths among 17,111 confirmed cases in western Africa. Scientists have debated whether Ebola could be weaponized into a weapon of mass destruction by terrorists. The consensus seems to be that this would be a very difficult undertaking because of the knowledge and laboratory skills that are required. Moreover, the biology of the virus does not lend itself well to weaponization. Of course, nefarious individuals could use Ebola in a number of ways, in much the same way that a suicide bomber straps on a vest laden with explosives. In theory a person could deliberately infect themselves with the virus and then attempt to infect others once they become symptomatic.
Existing Agents and Their Potential for Future Use
Important existing biological agents with the potential for weaponization for military or terrorist use include the following:
- 1.
Biological agents
- a.
Bacillus anthracis (anthrax; see Chapter 124 )
- b.
Yersinia pestis (plague; see Chapter 125 )
- c.
Francisella tularensis (tularemia ; see Chapter 126)
- d.
Brucella species (brucellosis; see Chapter 127 )
- e.
Coxiella burneti (Q fever; see Chapter 128 )
- f.
Rickettsia prowazekii (typhus fever; see Chapter 129 )
- g.
Orientia tsutsugamushi (scrub typhus; see Chapter 130 )
- h.
Rickettsia rickettsii (Rocky Mountain Spotted Fever; see Chapter 131 )
- i.
Vibrio cholerae (cholera; see Chapter 132 )
- j.
Shigella dysenteriae (shigellosis; see Chapter 133 )
- k.
Salmonella species (salmonellosis; see Chapter 134 )
- l.
Salmonella typhi (typhoid fever; see Chapter 135 )
- m.
Burkholderia mallei (glanders; see Chapter 136 )
- n.
Burkholderia pseudomallei (melioidosis; see Chapter 137 )
- o.
Chlamydia psittaci (psittacosis; see Chapter 138 )
- p.
Escherichia coli O157:H7 (hemorrhagic E. coli ; see Chapter 139 )
- a.
- 2.
Viral agents
- a.
Viral encephalitides (alphaviruses; see Chapter140 )
- b.
Tick-borne encephalitis virus (see Chapter 141 )
- c.
Viral hemorrhagic fever viruses (arenaviruses, bunyaviruses, filoviruses, flaviviruses; see Chapter 142 , Chapter 143 , Chapter 144 , Chapter 145 )
- d.
Chikungunya virus (see Chapter 146 )
- e.
Variola major virus (smallpox; see Chapter 147 )
- f.
Influenza virus (see Chapter 148 )
- g.
Monkeypox (see Chapter 149 )
- h.
Hantavirus pulmonary syndrome (see Chapter 150 )
- i.
Henipavirus (Hendra virus and Nipah virus encephalitis; see Chapter 151 )
- j.
SARS-CoV (see Chapter 152 )
- a.
- 3.
Toxins
- a.
Staphylococcal enterotoxin B (see Chapter 153 )
- b.
Clostridium botulinum toxin (botulism; see Chapter 154 )
- c.
Clostridium perfringens toxin (epsilon toxin; see Chapter 155 )
- d.
Marine toxin (see Chapter 156 )
- e.
T-2 toxin (trichothecene mycotoxins; see Chapter 157 )
- f.
Ricin toxin from Ricinus communis (castor beans; see Chapter 158 )
- g.
Aflatoxin ( Aspergillus species; see Chapter 159 )
- a.
- 4.
Other biological agents
- a.
Coccidioides immitis (coccidioidomycosis; see Chapter 160 )
- b.
Histoplasma capsulatum (histoplasmosis; see Chapter 161 )
- c.
Cryptosporidium parvum (cryptosporidiosis; see Chapter 162 )
- a.
Another way to view the relative importance of the above list of agents and diseases list is to consider The Centers for Disease Control and Prevention (CDC) strategy. The CDC categorizes bioterrorism agents or diseases as category A, B, or C. Category A agents pose the highest risk to the public and are characterized as follows:
- •
Easily disseminated or transmitted from person to person
- •
Can cause high mortality rates and possess the potential for profound public impact
- •
Could cause public panic and social disruption
- •
Require special preparations for adequate public health preparedness
Diseases and agents
- •
Anthrax ( Bacillus anthracis )
- •
Botulism ( Clostridium botulinum toxin)
- •
Plague ( Yersinia pestis )
- •
Smallpox (variola major)
- •
Tularemia ( Francisella tularensis )
- •
Viral hemorrhagic fever: filoviruses (e.g., Ebola and Marburg) and arenaviruses (e.g., Lassa and Machupo)
Category B agents are the next highest priority and are characterized by
- •
Μoderately easy to disseminate
- •
Cause moderate morbidity and low mortality
- •
Require specific enhancements of CDC’s diagnostic capacity and enhanced disease surveillance
Diseases and agents
- •
Epsilon toxin of Clostridium perfringens
- •
Food safety threats ( Salmonella species, Escherichia coli O157:H7, Shigella )
- •
Glanders ( Burkholderia mallei )
- •
Melioidosis ( Burkholderia pseudomallei )
- •
Psittacosis ( Chlamydia psittaci )
- •
Q fever ( Coxiella burnetii )
- •
Ricin toxin ( Ricinus communis —castor beans)
- •
Staphylococcal enterotoxin B
- •
Typhus fever ( Rickettsia prowazekii )
- •
Viral encephalitis (alphaviruses: Venezuelan equine encephalitis, eastern equine encephalitis, western equine encephalitis)
- •
Water safety threats ( Vibrio cholerae , Cryptosporidium parvum )
Category C agents form the third highest priority and include emerging pathogens that could be engineered for mass dissemination in the future because of the following:
- •
Availability
- •
Ease of production and dissemination
- •
Potential for high morbidity and mortality rates and major health impact
Agents
- •
Emerging and reemerging infectious diseases such as Nipah virus, hantavirus, human influenza, avian influenza, SARS and SARS-associated coronavirus (SARS-CoV), and Middle East respiratory syndrome (MERS)
Selected Emerging and Reemerging Infections with Weaponization Potential
Because emerging diseases are so diverse and endemic to different geographic locations, their complete description is beyond the scope of this chapter. However, some of these infections may become future threats as agents of biological warfare or terrorism. The most worrisome emerging infectious disease may well be the one we do not know about. Recent experience with HIV, Ebola hemorrhagic fever, SARS, monkey pox, West Nile fever, and hundreds of other “new” diseases reveal that we will continue to be surprised.
Avian Influenza
Avian influenza, or highly pathogenic avian influenza, has periodically caused human infections primarily through close contact with avian species, most often through occupational contact at chicken or duck farms in Southeast Asia. A large outbreak of avian influenza involving the H5N1 strain and human cases occurred in 2004 and originated in two countries from this region. No sustained human-to-human transmission was reported, but there is some evidence that isolated episodes did occur, and the potential exists for genetic reassortment between avian and human or animal strains of influenza. A recent report in the journal Science linked the influenza virus responsible for the 1918 epidemic to a possible avian origin. If true, avian influenza may pose a much greater danger to human populations than previously reported. The disease presents in humans in a fashion similar to other types of influenza viruses. It usually begins with fever, chills, headaches, and myalgias and often involves the upper and lower respiratory tract with development of cough, dyspnea, and, in severe cases, acute respiratory distress syndrome. Laboratory findings may include pancytopenia, lymphopenia, elevated liver enzymes, hypoxia, a positive reverse transcriptase-polymerase chain reaction (RT-PCR) test for H5N1, and a positive neutralization assay for H5N1 influenza strain. in vitro studies suggest that the neuraminidase (NA)-inhibitor class of drugs may have clinical efficacy in the treatment and prevention of avian influenza infection.
Human Influenza
The threat for pandemic spread of human influenza viruses is substantial. The pathogenicity of human influenza viruses is directly related to their ability to alter their eight viral RNA segments rapidly; the new antigenic variation results in the formation of new hemagglutinin (HA) and NA surface glycoproteins, which may go unrecognized by an immune system primed against heterologous strains.
Two distinct phenomena contribute to a renewed susceptibility to influenza infection among persons who have had influenza illness in the past. Clinically significant variants of influenza A viruses may result from mutations occurring in the HA and NA genes and expressed as minor structural changes in viral surface proteins. As few as four amino acid substitutions in any two antigenic sites can cause such a clinically significant variation. These minor changes result in an altered virus able to circumvent host immunity. Moreover, genetic reassortment between avian and human or avian and porcine influenza viruses may lead to the major changes in HA or NA surface proteins known as antigenic shift. In contrast to the gradual evolution of strains subject to antigenic drift, antigenic shift occurs when an influenza virus with a completely novel HA or NA formation moves into humans from other host species. Global pandemics result from such antigenic shifts.
Influenza causes in excess of 30,000 deaths and more than 100,000 hospitalizations annually in the United States. Pandemic influenza viruses have emerged regularly in 10- to 50-year cycles for the last several centuries. During the last century, influenza pandemics occurred 3 times: in 1918 (“Spanish influenza,” an H1N1 virus), in 1957 (Asian influenza, an H2N2 subtype strain), and in 1968 (Hong Kong influenza, an H3N2 variant). The 1957-1958 pandemic caused 66,000 excess deaths, and the 1968 pandemic caused 34,000 excess deaths in the United States. The 1918 influenza pandemic illustrates a worst-case public health scenario; it caused 675,000 deaths in the United States and 20 to 40 million deaths worldwide. Morbidity in most communities was between 25% and 40%, and the case-mortality rate averaged 2.5%. A reemergent 1918-like influenza virus would have tremendous societal effects, even in the event that antiviral medications were effective against this more lethal influenza virus.
SARS and SARS-Associated Coronavirus
SARS-associated coronavirus (SARS-CoV) emerged as the cause of SARS during 2003. That year, SARS was responsible for approximately 900 deaths and more than 8000 infections in people from at least 29 countries worldwide. Before a case definition had been clearly established, Chinese authorities reported to the World Health Organization (WHO) more than 300 cases of an atypical pneumonia with 5 related deaths, all originated from Guangdong province in China during February 2003. The infection quickly spread as infected patients traveled to Hong Kong and from there to Vietnam, Canada, and other locations. Only eight laboratory-confirmed cases occurred in the United States, but there is concern that the U.S. population is vulnerable to a widespread outbreak of SARS such as the one that occurred in China, Hong Kong, Singapore, Toronto, and Taiwan in 2003.
A SARS case definition evolved from this initial report to the WHO by Chinese health authorities in February 2003. A case was initially defined by clinical criteria; a suspected or probable case was defined as an illness that included potential exposure to an existing case and fever with pneumonia or respiratory distress syndrome. In April 2003, a confirmed case was defined as a case from which SARS-CoV was isolated from culture. SARS-CoV infections have an incubation period of 2 to 10 days. Systemic symptoms such as fever and chills followed by a dry cough and shortness of breath begin within 2 to 7 days. Patients may develop pneumonia and lymphopenia by days 7 to 10 of the illness. Most patients with SARS-CoV have a clear history of exposure either to a patient with SARS or to a setting in which SARS-CoV is known to exist. Laboratory tests may be helpful but do not reliably detect infection early during the illness. SARS-CoV should be suspected in patients requiring hospitalization for radiographically confirmed pneumonia or acute respiratory distress syndrome of unknown etiology and one of the following risk factors during the 10 days before the onset of illness: ( ) travel to China, Hong Kong, or Taiwan, or close contact with an ill person having a history of such travel; ( ) employment in an occupation associated with a risk for SARS-CoV exposure; or ( ) inclusion in a cluster of cases of atypical pneumonia without an alternative diagnosis.
A “respiratory hygiene/cough etiquette” strategy should be adopted in all SARS-affected health care facilities. All patients admitted to the hospital with suspected pneumonia should receive the following measures: (1) they should be placed in droplet isolation until it is determined that isolation is no longer indicated (standard precautions are appropriate for most community-acquired pneumonias; droplet precautions for nonavian influenza); (2) they should be screened for risk factors of possible exposure to SARS-CoV; and (3) they should be evaluated with a chest radiograph, pulse oximetry, complete blood count, and additional workup as indicated. If the patient has a risk factor for SARS, droplet precautions should be implemented pending an etiologic diagnosis. When there is a high index of suspicion for SARS-CoV disease, the patient should be treated in terms of SARS isolation precautions immediately (including airborne precautions), and all contacts of the ill patient should be identified, evaluated, and monitored. Although ribavirin, high-dose corticosteroids, and interferons have been used in treatment, it is unclear what effect they have had on clinical outcome. No definitive therapy has been established. Empiric antibiotic treatment for community-acquired pneumonia following the current American Thoracic Society/Infectious Diseases Society of America guidelines is recommended pending etiologic diagnosis. Diagnostic tests for SARS-CoV include antibody testing using an enzyme immunoassay and RT-PCR tests for respiratory, blood, and stool specimens. In the absence of known SARS-CoV transmission, testing is recommended only in consultation with public health authorities. Testing for influenza, respiratory syncytial virus, pneumococcus, chlamydia, mycoplasma, and legionella should be conducted, as the identification of one of these agents excludes SARS by case definition. Clinical samples can be obtained during the first week of illness with a nasopharyngeal swab plus an oropharyngeal swab and a serum or a plasma specimen. After the first week of illness, a nasopharyngeal swab plus an oropharyngeal swab and a stool specimen should be obtained. Serum specimens for SARS-CoV antibody testing should be collected when the diagnosis is first suspected and at later times as indicated. An antibody response can occasionally be detected during the first week of illness, is likely to be detected by the end of the second week of illness, and at times may not be detected until more than 28 days after the onset of symptoms. Respiratory specimens from any of several different sources may be collected for viral and bacterial diagnostics, but the preferred specimens of choice are nasopharyngeal washes or aspirates.
Middle East Respiratory Syndrome (MERS-CoV)
MERS-CoV is a disease caused by a coronavirus and results in severe acute respiratory disease including fever, chills, cough, and dyspnea. Some patients also develop nausea, vomiting, and diarrhea. It has a 30% mortality rate. Most patients who have died had underlying comorbidities and developed pneumonia or renal failure. The illness was first reported in Saudi Arabia in September 2012; most cases appear to be limited to the Arabian Peninsula. The incubation period ranges from 2 to14 days. The illness can spread from person to person but it requires close contact, and no sustained transmission had been reported by late 2014. The virus could evolve and lead to sustained transmission, but this cannot be predicted with certainty. There is no vaccine for MERS-CoV and there is no specific treatment, but there is ongoing research by the National Institutes of Health (NIH) and other entities to fill this gap.
Nipah and Hendra Viruses
The Nipah and Hendra viruses are closely related but distinct paramyxoviruses that compose a new genus within the family Paramyxoviridae. The Nipah virus was discovered in Malaysia in 1999 during an outbreak of a zoonotic infection, now called Nipah virus encephalitis, involving mostly pigs and some human cases. Hendra, the causative agent of Hendra virus disease, was identified in a similar outbreak involving a single infected horse and three human cases in Southern Australia in 1994. It is believed that certain species of fruit bats are the natural hosts for these viruses and remain asymptomatic. Horses and pigs act as amplifying hosts for the Hendra and Nipah viruses, respectively. The mode of transmission from animal to humans appears to require direct contact with tissues or body fluids or with aerosols generated during butchering or culling. Personal protective equipment including gowns, gloves, and respiratory and eye protection is advised for agricultural workers culling infected animal herds. Thus far, human-to-human transmission of these viruses has not been reported.
In symptomatic cases, the onset of disease begins with flu-like symptoms and rapidly progresses to encephalitis with disorientation, delirium, and coma. Fifty percent of those with clinically apparent infections have died from their disease. There is currently no approved treatment for these infections, and, therefore, therapy relies heavily on supportive care. The antiviral drug ribavirin has been used in past infections, but its effectiveness remains unproven in clinically controlled studies. Although no person-to-person transmission is known to have occurred, barrier nursing and droplet precautions are recommended because respiratory secretions and other bodily fluids are known to harbor the virus. The clinical laboratory should be notified before specimens are sent as these may pose a laboratory hazard. Specimens for viral isolation and identification should be forwarded to a reference laboratory. Requests for testing should come through public health departments, which should contact the CDC Emergency Operations Center at 770-488-7100 before sending specimens.
Biological Response Modifiers
BRMs direct the myriad complex interactions of the immune system. BRMs include erythropoietins, interferons, interleukins, colony-stimulating factors, granulocyte and macrophage colony-stimulating factors, stem cell growth factors, monoclonal antibodies, tumor necrosis factor inhibitors, and vaccines. A growing understanding of the structure and function of BRMs is driving the discovery and creation of many novel compounds including synthetic analgesics, antioxidants, and antiviral and antibacterial substances. For example, BRMs are being used to treat debilitating rheumatoid arthritis by targeting cytokines that contribute to the disease process. By neutralizing or eliminating these targeted cytokines, BRMs may reduce symptoms and decrease inflammation. BRMs may also be used as anticarcinogens, with the following goals: ( ) to stop, control, or suppress processes that permit cancer growth; ( ) to make cancer cells more recognizable, and therefore more susceptible, to destruction by the immune system; ( ) to boost the killing power of immune system cells, such as T cells, natural killer cells, and macrophages; ( ) to alter growth patterns in cancer cells to promote behavior like that of healthy cells; ( ) to block or reverse the processes that change a normal cell or a precancerous cell into a cancerous cell; ( ) to enhance the ability of the body to repair or replace normal cells damaged or destroyed by other forms of cancer treatment, such as chemotherapy or radiation; and ( ) to prevent cancer cells from spreading to other parts of the body. ,
More of these promising new drugs are currently in development. It can be readily theorized that research to develop various BRMs can be subverted to a malicious end. That is, instead of using BRMs to suppress cancer growth or to decrease disease susceptibility, researchers could develop compounds to cause illness and death. Other drugs could be designed to alter certain metabolic processes or to alter brain chemistry to affect cognition or mood. The opportunity for mischief is limited only by the imagination of the person with ill intent.
Synthetic Biology and Bioengineered Pathogens
The field of synthetic biology had its beginnings near the turn of the millennium with the idea that basic engineering principles could be applied to biological systems at the cellular and genetic levels to create new and improved organisms. Synthetic biology is the “engineering of biology.” The goal and end products of the engineering are conventionally to be used for the benefit of humankind. However there has been increasing concern that synthetic biology could be used for nefarious purposes.
A precise definition of synthetic biology has not yet been established; however, a consensus is building that synthetic biology is defined as the use of molecular biology tools and techniques to forward the engineering of cellular behavior. There is disagreement among scientists whether the new field of synthetic biology will allow terrorists to create biological agents with more lethal characteristics or create completely novel pathogens with enhanced pathogenicity and weaponization potential in an easier fashion. Some argue that synthetic biology causes “de-skilling” of biological techniques and allows laboratory processes to become easier for less experienced scientists or even lay-people to master. Others maintain that the tacit or unwritten laboratory skills that only a few highly trained scientists possess and which are very difficult to transfer and very difficult for a terrorist to acquire. Social scientists have carefully studied these tacit skills and argue that these techniques are very difficult to pass on from scientist to scientist without considerable effort that is often impossible even under the most ideal circumstances. This difficulty was historically present in both the U.S. and Soviet biological weapons programs each of which were extremely well funded and staffed with competent scientists.
Nevertheless, the rapid advance of synthetic biology has the potential to alter the present and future threat of biological weapons. , , Already, complete or partial genomic sequence data for many of the most lethal human pathogens (such as anthrax, plague, and the smallpox virus) have been published and are widely available via the Internet. In addition to the enormous explosion in our knowledge of human pathogens, there is a parallel increased understanding of the complexities of the human immune response to foreign agents and toxins. Such knowledge has led to a deeper understanding of the development of basic immunity to a variety of different human infectious diseases.
With this increase in scientific knowledge has come the power to manipulate the immune system at its most fundamental level. As we prepare for future threats, we must not ignore the potential quantum leap that synthetic biology offers to terrorists for developing new biological-warfare threats. Examples of biological threats that could be produced through the use of synthetic biology include the following: ( ) microorganisms resistant to antibiotics, standard vaccines, and therapeutics; ( ) innocuous microorganisms genetically altered to produce a toxin, a poisonous substance, or an endogenous bioregulator; ( ) microorganisms possessing enhanced aerosol and environmental stability characteristics; ( ) immunologically altered microorganisms able to defeat standard threat identification and diagnostic methods; ( ) genetic vectors capable of transferring human and foreign genes into human cells for therapeutic purposes ; and ( ) combinations of these with improved delivery systems.
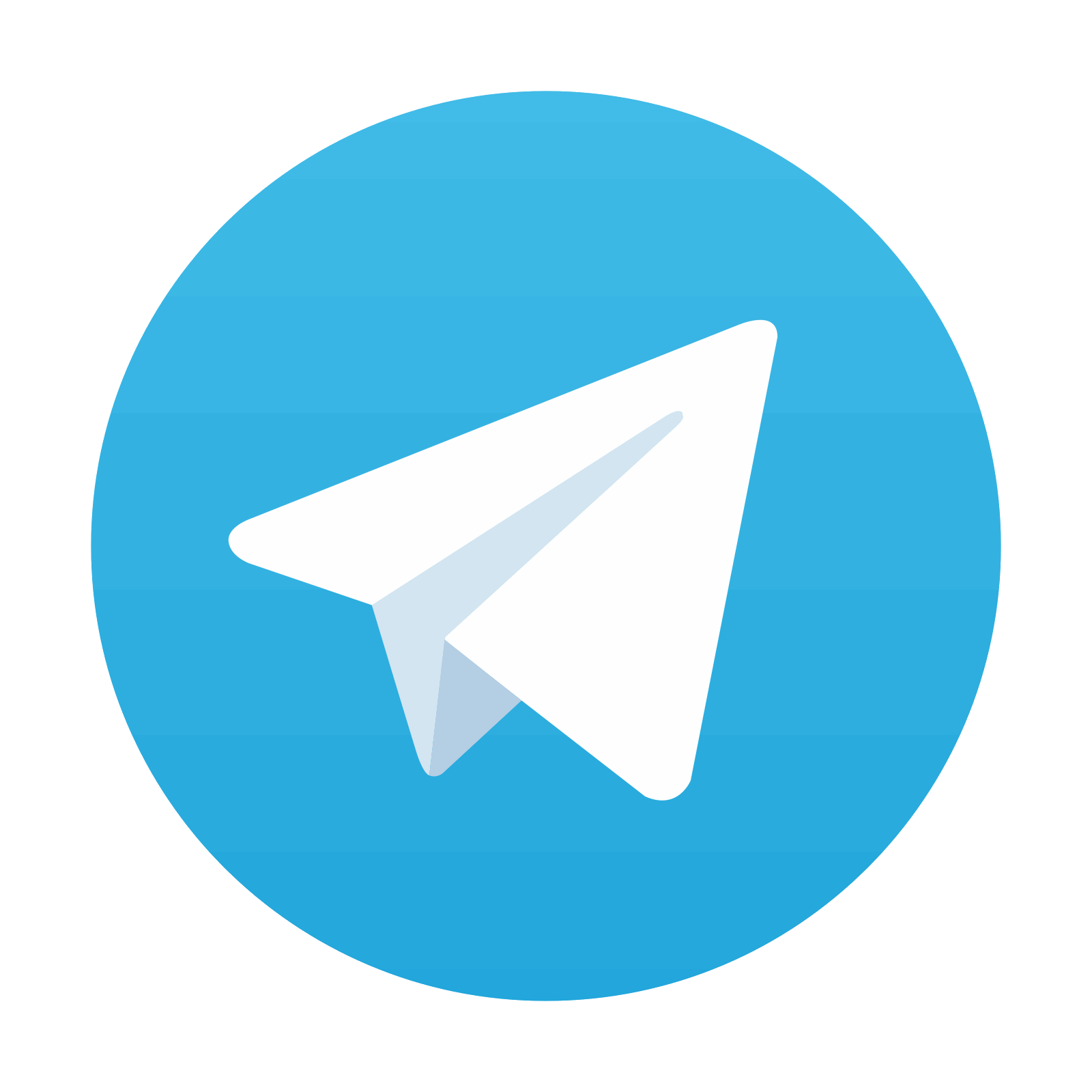
Stay updated, free articles. Join our Telegram channel

Full access? Get Clinical Tree
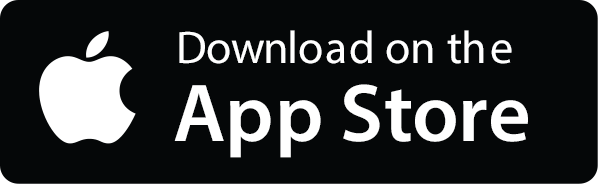
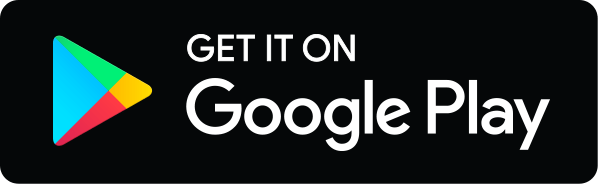