Abstract
The use of fluoroscopy has revolutionized interventional pain management. Fluoroscopy is required in advanced procedures where precise needle placement is necessary. The increasing use of fluoroscopy necessitates that the pain physician be aware of radiation safety to limit the radiation exposure to the patient and personnel. The factors affecting radiation exposure to personnel include the time or duration of x-ray exposure, distance from the source of the x-rays, and protection from the radiation. It should be noted that the major source of radiation to personnel is the patient or fluoroscopy table which serves as a conduit for scattered radiation. There remains a significant dearth of published data on interventions to minimize cumulative radiation exposure in interventional pain physicians. In the small number of available studies, it does appear that procedural and equipment changes, peer-based education, coaching, and shielding improvements effectively reduce radiation exposure in interventional pain physicians.
Low-osmolality contrast media (LOCM) are the most commonly used as they cause less side effects compared to high-osmolality contrast media. Patients with a history of allergic reaction to contrast media should be prepped with steroid, with or without diphenhydramine. Gadolinium-based contrast agents can be used in a patient with a history of contrast allergy as allergic reactions to these agents are extremely rare. It may cause nephrogenic systemic fibrosis and should not be used in patients with renal insufficiency.
Keywords
contrast media, fluoroscopy, radiation dose, radiation safety, radiobiology
The use of fluoroscopy has revolutionized interventional pain management. Fluoroscopy is required in advanced procedures where precise needle placement is required. These procedures include interventions for back and neck pain, such as epidural steroid injections, facet joint injections, facet joint nerve (medial branch) blocks and rhizotomy, sacroiliac joint injections, discography, placement of spinal cord stimulators, and the newer interventional procedures, such as biaculoplasty, nucleoplasty, and vertebral augmentation. Fluoroscopy is also used in lumbar paravertebral sympathetic blocks as well as visceral sympathetic blocks, such as celiac plexus blocks, superior hypogastric plexus blocks, and ganglion impar blocks. Blocks outside the vicinity of the spine also benefit from fluoroscopic guidance and include trigeminal nerve blocks.
Several studies on epidural steroid injections have shown the usefulness of fluoroscopy. Anatomic landmarks can be difficult to recognize especially in obese, elderly, or arthritic patients. Access to the epidural space is not always straightforward, especially in the sacral region where surface landmarks are not clearly delineated in the adult patient. In addition, fluoroscopy can inform the physician of important details not clearly known to the patient. For example, a patient who was treated by one of the authors had a history of a laminectomy and fusion, and presented with a right L1 radiculopathy ( Fig. 77.1 ). She did not realize that she had a bone stimulator in situ that was placed at the time of surgery. As the device was clearly evident on x-ray imaging, a right paramedian epidural steroid injection was safely performed with the needle insertion performed a safe distance from the bone stimulator ( Fig. 77.2 ).


In a nationwide survey done in the United States in 2002, investigators found that there was wide variability in the use of fluoroscopy. At that time, private practitioners used fluoroscopy more than those in academic centers. In the cervical region 73% of private practitioners used fluoroscopy compared to only 39% in academic institutions. The transforaminal approach to epidural injections was used in patients who had previous laminectomy by 61% of private practitioners compared to 15% of those in academic institutions. Although these numbers may not be applicable at the present time, it shows that a fair number of pain practitioners do not use fluoroscopy for their spine interventions. For transforaminal epidural steroid injections, confirmation of correct needle placement and spread of the dye in the anterior epidural space can only be demonstrated by fluoroscopy or more advanced radiological imaging.
One of the earlier studies on epidural steroid injections showed that blind placements were accurate in 83 of 100 patients. In this study, in which 85% of the injections were performed in the lumbar area, the anesthesiologists who performed the interlaminar epidural placements were well experienced, and yet the incidence of inaccurate placement was 17%. Another study in which the epidurals were again placed by experienced anesthesiologists and an orthopedic surgeon showed a 75% success rate with blind epidural placements.
In cervical epidural placements a study noted that there was a 47% success rate on the first attempt of needle placement. In 63% of the placements (24 of 38 epidurals), a second attempt was required. The lack of reliability of the loss-of-resistance technique may be partially due to the lack of continuity of the ligamentum flavum in the cervical area. Another finding in the study was a 51% incidence (19 of 38) of unilateral spread of contrast, although the authors inserted their needle slightly lateral to the midline. In addition to the slightly lateral insertion of the needle, the unilateral spread may also be caused by the plica mediana dorsalis, a thin septum dividing the posterior epidural space. The presence of the plica mediana dorsalis has not been demonstrated in the cervical region, but in the lumbar and thoracic levels the plica mediana dorsalis has been shown to divide the posterior epidural space into compartments hindering the free flow of the injected solution. Knowledge that the contrast spread is unilateral can allow for readjustment of the needle tip. One of the more interesting findings in the study by Stojanovic et al. is that the spread of the contrast in the ventral epidural space occurred in only 28% of the patients (11 out of 38 epidurograms). The spread of the injectate in the anterior epidural space is important since this is the location of herniated intervertebral disc(s) and the interface between the herniated disc and the nerve root. The placement of the drug in the anterior epidural space is the rationale for transforaminal epidural steroid injections (see Chapter 63 ).
Caudal epidural steroid injections are ideally performed under fluoroscopic guidance. Without image guidance, experienced radiologists incorrectly place the caudal needle 38% of the time. Renfrew et al. showed that the experience of the physician improved the success rate of blind caudal epidural placements. Physicians who performed less than 10 epidurals had a success rate of 48% compared to 62% by experienced physicians. Another study showed that senior physiatrists successfully placed the caudal needle in 74% of their initial attempts. Their success rate improved to 88% when landmarks were identified easily. It appears that the most common site of incorrect needle placement is in the subfascial plane posterior to the sacrum. Correct placement of the caudal needle is intuitively improved when fluoroscopy is utilized. In a study of 116 caudal steroid injections done under fluoroscopy, radiologists found that the success rate was 97%. In this study, it was found that the injection of 9- to 15-mL volume reached the mid to upper lumbar spine except in those patients with a severely stenotic spinal canal.
In patients who have had a previous laminectomy, it was noted that the mean number of attempts to place the needle in the epidural space successfully is 2 ± 1. The difficulties in placing the epidural needle may be due to the resection of the ligamentum flavum, as well as fibrosis and adhesions within the epidural space, rendering the loss-of-resistance technique equivocal. In a study by Fredman et al. in 25 of 48 patients, the Touhy needle and epidural catheter were placed one or two intervertebral spaces above or below the desired level. The lack of reliability of surface landmarks may be due to the surgical removal of the posterior spinous process making the count of the vertebral levels difficult. When 5 mL of contrast medium was injected, the contrast reached the level of pathology in only 26% (12 of 47) of the patients. It has been postulated that this is due to postoperative adhesions that hindered the spread of the dye. The success rates of needle placements in the studies are shown in Table 77.1 .
Route | Blind/Fluoroscopy | Physician | Experience/Faculty | Success Rate (%) | Reference |
---|---|---|---|---|---|
Cervical | Fluoroscopy | Anesthesiologists | Faculty/house staff | 100 a | 4 |
Lumbar b | Blind | Anesthesiologists | Experienced | 83 | 1 |
Lumbar | Blind | Anesthesiologists and orthopedic surgeons | Experienced | 75 | 3 |
Lumbar, s/p surgery | Blind | Anesthesiologists | Attending | 92 | 12 |
Caudal | Blind | Radiologists | Attending | 48–62 c | 9 |
Caudal | Blind/fluoroscopy | Radiologists | Attending | 74–88 | 10 |
Caudal | Fluoroscopy | Radiologists | Attending | 97 | 11 |
a Up to four attempts were made in successfully placing the needle in the epidural space.
b Eight-five percent of the injections were in the lumbar area.
c Experienced radiologists had a success rate of 62% compared to 48% for inexperienced anesthesiologists (see text).
Manchikanti et al. emphasized the necessity of using fluoroscopy in epidural steroid injections. The low incidence of contrast reaching the level of pathology during “blind” injections requires the use of fluoroscopy to eliminate the question of incorrect needle placement. Documentation of the spread of contrast can be correlated with the response of the patient. However, it should be noted that there are differences in the flow characteristics between the contrast media and the steroid solution, and that the flow of dye may not completely predict the flow of the steroid injectate. The steroid solution may be more limited in its distribution because it tends to precipitate in its diluent, which is typically either a local anesthetic or saline.
In addition to confirmation of the correct needle placement, the other main advantage of using fluoroscopy is the determination of the needle tip in an inadvertent location prior to injection. Unintentional intravascular or intrathecal injection may occur in spite of negative aspiration of blood or cerebrospinal fluid, respectively, through the needle. The vascular uptake of contrast can be detected when live fluoroscopy is used during injection, or can be suspected when there is immediate contrast disappearance after injection. Intravascular injections are especially hazardous via the transforaminal route as the radicular arteries accompanying the nerve root feed the spinal cord, depending on the level involved. Smuck et al. performed a prospective observational study on the incidence of simultaneous epidural and vascular injection during cervical transforaminal epidural injections. They found that vascular-only injection had a 13.9% incidence where as a vascular and epidural injection occurred in 18.9% of their study patients. They recommended real-time use of fluoroscopy for contrast injection. A similar study was performed by the same group of authors at lumbosacral levels. Although the incidence of vascular and vascular plus epidural injection was much lower than at cervical levels, they made the same recommendation for using live fluoroscopy during these procedures. Digital subtraction angiography (DSA) can further increase the sensitivity of real-time fluoroscopy for intravascular detection. McLean et al. found that with live fluoroscopy alone, intravascular injection was detected in 17.9% of their study population. When DSA was employed, the rate rose to 32.8% (see Chapter 63 ).
Although not typically as catastrophic as injection into an artery, intrathecal injection of injectate needs to be detected as well. For example, the unintentional injection of 3 mL of lidocaine 1% into the intrathecal space is enough to cause a significant motor and sensory block with accompanying hemodynamic alterations. In addition, as the injectate is not placed in the epidural space, the intended therapeutic effect may be lacking. Recognizing the characteristic spread of contrast intrathecally can help to avoid this complication. One may look for a distinctive contrast-fluid level seen on lateral imaging with the patient in the prone position ( Fig. 77.3 ).

There are reasons for not utilizing fluoroscopy in epidural steroid injections. These include the avoidance of radiation; costs associated with the fluoroscopic equipment, its maintenance and technicians; inconvenient scheduling; location of the x-ray facility; and allergy to iodinated contrast agents (though this does not preclude fluoroscopy use, and there are other contrast alternatives that can be substituted). However, the substantial potential for incorrect needle location makes fluoroscopy desirable for epidural steroid injections, and necessary or strongly recommended for other procedures. The added benefits of fluoroscopy include the documentation of whether the spread of contrast is unilateral, located in the ventral epidural space, or whether it reached the desired level of pathology. The documentation of correct needle placement and ideal spread of the injectate eliminates technical factors as a cause of lack of response of the procedure in the patient. For these reasons, the use of fluoroscopy and contrast epiduroscopy is becoming the standard of care for epidural steroid injections as well as for other spinal procedures. The Practice Guidelines for Spinal Diagnostic and Treatment Procedures published by the International Spine Intervention Society (ISIS) mandates the use of fluoroscopy for the performance of transforaminal epidural steroid injections as well as medial branch blocks. One final factor to consider when deciding whether to make the use of fluoroscopy standard during spine procedures is reimbursement. For example, in January 1, 2010, the established Current Procedural Terminology (CPT) codes for medial branch blocks were removed and replaced with codes that bundle together the medial branch block(s) and use of fluoroscopy for needle guidance. There is no longer a separate billable code for a medial branch block that has been performed without x-ray guidance.
The above discussions focused on the importance of fluoroscopy in epidural steroid injections. While one can do interlaminar epidural steroid injections (ESIs) without fluoroscopy, fluoroscopy is an integral part of facet and sacroiliac joint injections, and the other interventional pain procedures.
Fluoroscopy Machine
For medical imaging purposes, x-radiation can be produced with the aid of electricity. A current, which is measured in milliamperes (mA), passes from an electrically heated negatively charged filament (the cathode) to an anode under high voltage (kilovolt peak, kVp) within an x-ray tube. The anode is typically tungsten, which has a high melting point. As the electrons interact with the anode, energy is released as both heat and photons called x-rays. These x-rays will then exit the tube and either become absorbed by or pass through the patient. The energy that passes through the patient will enter an image intensifier where it is converted to a visible image that is displayed on a monitor screen and can be saved as part of the permanent medical record.
The important parts of the fluoroscopy machine include the x-ray tube, the image intensifier, the C-arm, and the control panel ( Fig. 77.4 ). The x-ray tube fires the beam of electrons through a high-voltage vacuum tube, forming x-rays that are emitted through a small opening. The image intensifier collects the electromagnetic particles and translates them into a usable image that can be viewed on a television monitor. The C-arm allows for the x-ray source and recording source (i.e., the image intensifier) to be on opposite sides of the patient. By its design, it will also facilitate the positioning of the fluoroscope for the physician to easily obtain anteroposterior, oblique, and lateral views of the patient. The control panel ( Fig. 77.5 ) contains the controls for the technician to make adjustments either to the image itself or the settings used to create the x-ray image. For the latter, typically the “automatic brightness control,” or ABC, system is employed (see below). Also located in the control panel are the controls for magnification and collimation of the image. Many machines also have the software required for DSA, which is useful to detect inadvertent vascular placement. The quality of image contrast depends on the balance between the tube voltage (or kVp) and the tube current. The kVp is the voltage through which the electron beam passes in the x-ray vacuum tube. Increasing the kVp increases the penetrability of the x-ray beam through the patient and thereby decreases its absorption. This will act to produce brighter, more exposed images, but will decrease the contrast. The fluoroscopic examination of the spine of a normal-sized adult starts with the kVp set at ∼75; larger patients require a higher kVp. The typical settings are 80–100 kVp for the back, 50 kVp for the hands, and 70 kVp for the abdomen. Broadman recommends the highest kVp setting that produces the adequate contrast or grayscale ordering to minimize x-ray exposure for the patient and personnel. The x-ray (tube) current reflects the number of electrons fired through the high-voltage vacuum tube. Higher tube currents mean more x-rays are produced and emitted. The tube current is set between 1 and 5 mA; lower settings are adequate for most interventional fluoroscopy procedures.


The image contrast is obtained by balancing the tube voltage or kVp against the tube current. Higher kVp settings reduce the number of x-rays absorbed and decrease exposure time. However, if the kVp settings are too high, the image will lack the necessary contrast to provide useful visualization. In terms of the resulting image, it can be likened to a photograph taken with an inappropriately bright flash in which all objects appear overexposed and the ability to distinguish features falters. A nice component of fluoroscopy machines is the ABC system in which the computer automatically analyzes the image contrast and makes the appropriate tube current adjustments, balancing image contrast and patient safety. It is recommended that the interventional pain physician leave the machine on the ABC system during the performance of most interventional procedures.
Radiation Safety
The increasing use of fluoroscopy necessitates that the pain physician be aware of radiation safety to limit the radiation exposure to the patient and personnel. Editorials, review articles, book chapters, monographs, and government publications are available to help the interventional pain physician better understand the concept of radiation safety.
Radiation is the process by which energy, in the forms of waves or particles, is emitted from a source. Radiation includes x-rays, gamma rays, ultraviolet, infrared, radar, microwaves, and radio waves. Radiation absorbed dose (rad) is the unit of measure that expresses the amount of energy deposited in tissue from an ionizing radiation source. Units of gray (Gy) are preferred, instead of rad, in the International System of Units (SI). A gray is defined as the quantity of radiation that results in an energy deposition of 1 joule per kilogram (1 J/kg) within the irradiated material; 1 Gy is equivalent to 100 rad and to 1000 mGy.
Different types of radiation may have similar absorbed doses but produce different biologic effects. To predict occupational exposure from x-radiation, the rad is converted to radiation equivalent man (rem) in a 1:1 ratio. The unit of dose equivalent to rem in the SI system is the sievert (Sv); 1 rem is equivalent to 1 rad and 100 rem is equivalent to 1 Sv.
There remains a paucity of literature on ionizing radiation exposure and dose among interventional pain physicians. The majority of published reports use fluoroscopic or screening times as a proxy for radiation dose ( Table 77.2 ). In general, published data have shown that fluoroscopic times were shorter in private practice compared to university settings where supervision of trainees occurs. Nevertheless, there is wide variation in fluoroscopy times and radiation dose in attending physicians in both private practice and university settings. Fluoroscopic times may further vary by mode used (continuous vs. pulsed), the experience of the radiographer, technician, or interventionalist, and difficulties in obtaining a satisfactory image given the severity of degenerative disease or patient body habitus. Overweight patients also appear to require significantly longer fluoroscopy times and have higher radiation exposure compared to normal-weight patients.
Fluoroscopy Time Per Procedure (Seconds) | ||||||||||||
---|---|---|---|---|---|---|---|---|---|---|---|---|
Facet Joint Nerve Block | Lumbar Facet Joint Injection | Facet Denervation | Caudal Epidural | Translaminar Epidural | Transforaminal Epidural | Sympathetic Block | Sacroiliac Joint Injection | SCS Trial | ||||
Cervical | Lumbar | Cervical | Lumbar | SGB | LSB | |||||||
Manchikanti et al. | 5.9 ± 0.07 | 5.7 ± 0.09 | — | 12.7 ± 1.49 | 3.75 ± 0.13 | 8.8 ± 0.78 | 10.9 ± 0.72 | 6.5 ± 0.68 | 12.0 ± 1.49 | — | — | |
Manchikanti et al. | 5.8 ± 0.11 | — | — | 3.7 ± 0.29 | 10.6 ± 0.60 | 7.4 ± 1.09 | — | — | ||||
Manchikanti et al. | 4.5 ± 0.07 | — | — | 2.7 ± 0.27 | 8.4 ± 0.5 | 4.0 ± 0.35 | — | — | ||||
Botwin et al. | — | — | — | — | 12.55 | — | — | — | — | — | — | |
Botwin et al. | — | — | — | — | — | — | — | 15.16 | — | — | — | — |
Zhou et al. | 81.5 ± 12.8 | — | 46.6 ± 4.2 | — | — | 50.6 ± 41.9 | — | |||||
Wininger et al. | — | — | — | — | — | — | — | — | — | — | — | 133.4 ± 84 |
Hanu-Cernat et al. | — | 28.41 ± 18.5 | 29.50 ± 17.39 | 38.23 ± 13.33 | 16 ± 6.98 | — | — | — | — | 43 ± 20.73 | — |
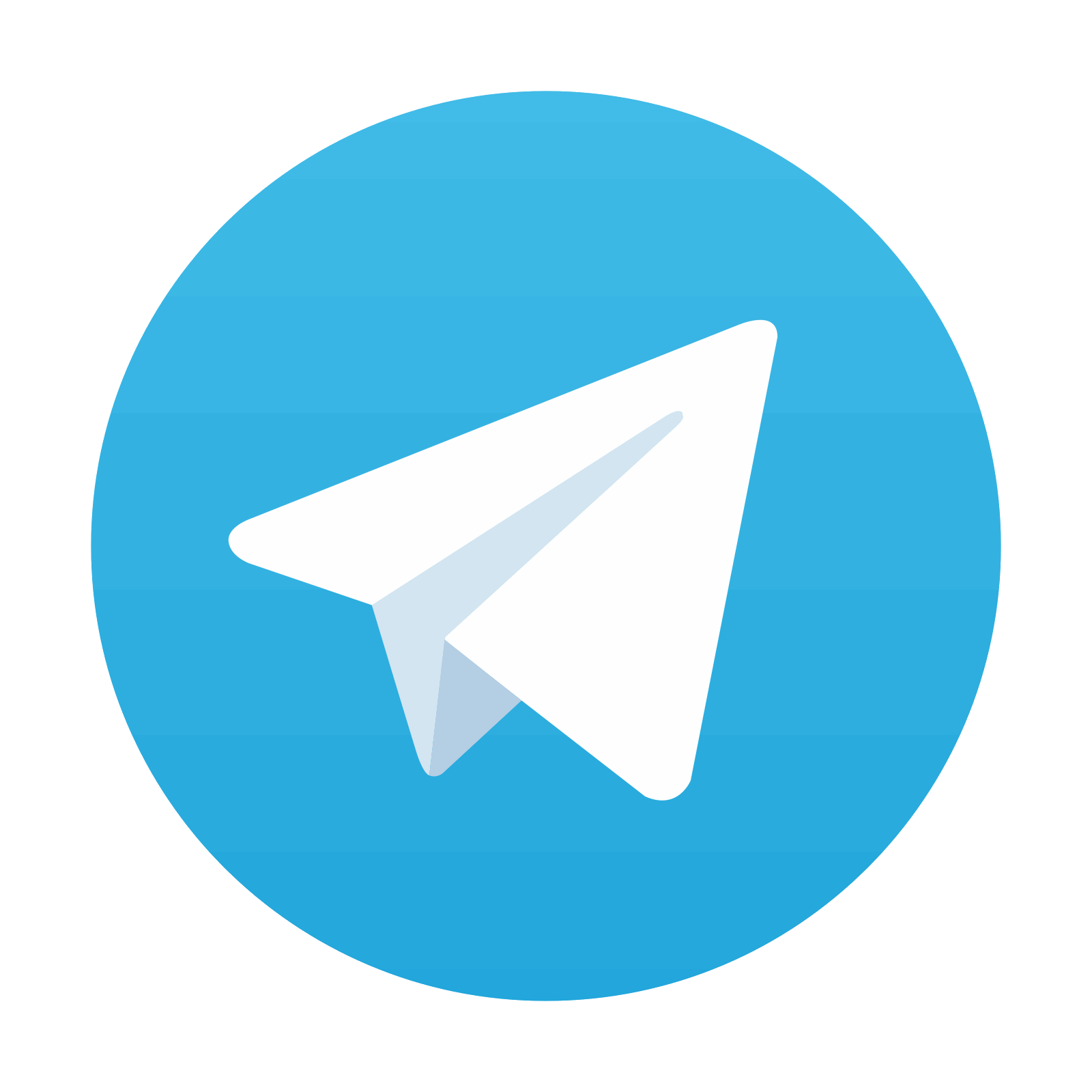
Stay updated, free articles. Join our Telegram channel

Full access? Get Clinical Tree
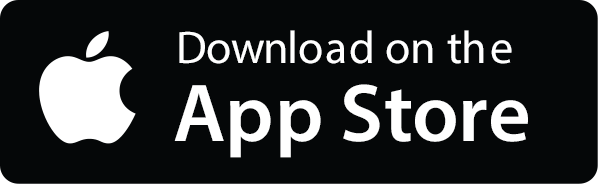
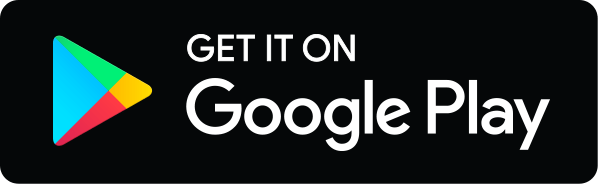
