11 Evolution of Perioperative Echocardiography
Many characteristics define a cardiac anesthesiologist, and these have evolved over time.1 Some of these include intimate knowledge of cardiac diseases and the interaction of anesthesia with them, knowledge of the special techniques used by cardiac surgeons including, initially, pure moderate hypothermia and, subsequently, cardiopulmonary bypass (CPB), deep hypothermic circulatory arrest, cardioplegia, off-pump coronary artery bypass grafting, and minimally invasive and robotic surgery. But one of the cardinal characteristics of cardiac anesthesiologists has been their adoption and application of special monitoring techniques. Early on this involved electrocardiographic (ECG), electroencephalographic, arterial, central venous, and left atrial pressure monitoring, and use of arterial blood gases and tests of coagulation. In the 1970s, use of the pulmonary artery catheter (PAC) was introduced. Since about 1990, expertise with use of echocardiography has become one of the defining characteristics of the accomplished cardiac anesthesiologist. Today, few anesthesia or cardiac surgical groups would accept an anesthesiologist who wishes to provide anesthesia for cardiac cases if they have not been trained in perioperative echocardiography. Residents seeking fellowship training in cardiothoracic anesthesia view the quality of experience and teaching of echocardiography as critical criteria in selecting a fellowship. The authors believe that the introduction of echocardiography into cardiac surgery and anesthesia has contributed to the improved success and safety of cardiac surgery since the early 1990s.
As Feigenbaum2 notes, the word evolution usually is reserved for changes of natural phenomena, but it is appropriate to use it in reference to the development of diagnostic ultrasound (e.g., echocardiography) because this represents an attempt to mimic a natural phenomenon. Some mammals (e.g., bats and aquatic mammals) use “diagnostic ultrasound” to visualize their environments, a phenomenon that was first recognized by Lazzaro Spallanzani (1729–1799). A number of previous articles and sections of other texts have reviewed the history of medical diagnostic ultrasound and echocardiography.2–15 The authors have relied heavily on these prior works and frequently quote from them. What becomes immediately apparent in reviewing this history is that it has been an international effort with contributions from engineers, scientists, and clinicians from many different nations. The knowledge and experience of the authors of this chapter result in a particular emphasis on developments in North America, and they acknowledge that they may have unintentionally overlooked important contributions and events that have occurred elsewhere.
Early developments leading to the medical use of ultrasound
The Roman architect Vitruvius coined the word echo (Table 11-1). The French friar Marin Mersenne (1588–1648) frequently is referred to as the “father of acoustics” because he first measured the velocity of sound, whereas the English physicist Robert Boyle (1627–1691) recognized that a medium was necessary for the propagation of sound.13 The Italian Lazzaro Spallanzani (1729–1799) is sometimes referred to as the “father of ultrasound” because he deduced that bats must emit ultrasound waves (inaudible to humans) and listen to the echoes to navigate, based on his observations in the 1790s that bats navigate well when blindfolded but not when he plugged their ears. The Austrian mathematician and physicist Christian Johann Doppler (1803–1853) noted that the pitch of sound varied if the source of sound was moving and derived the mathematical relationship between change in pitch (frequency) and the relative motion (velocity) between the source and the observer. As an indication of the ingenuity of early scientific investigators, Buys Ballot at Utrecht in 1845 confirmed Doppler’s theory and formula experimentally by having trumpeters play notes on railroad cars pulled at different speeds and employed musicians with perfect pitch to identify the frequencies they heard.9 Doppler also predicted (which was later confirmed) that the frequency of light would decrease from a receding object (e.g., stars [“red shift”]) and increase (toward blue) if approaching. Interestingly, manufacturers of color-flow Doppler systems chose to use the opposite convention, using red to color-code flow moving toward the transducer and blue for flow moving away from the transducer (“Red returning, Blue away”).
TABLE 11-1 Early Developments Leading to Medical Use of Ultrasound
Name | Date* | Development |
---|---|---|
Marcus Vitruvius (Roman) | 80–15 BC | Coined word echo |
Marin Mersenne (French) | 1588–1648 | “Father of acoustics” Measured speed of sound |
Robert Boyle (English) | 1627–1691 | Recognized a medium was necessary for propagation of sound |
Lazzaro Spallanzani (Italian) | 1727–1799 | “Father of ultrasound” Demonstrated that bats navigated by echo reflection |
Christian Doppler (Austrian) | 1803–1853 | In 1842, he described Doppler effect and mathematical relations between change in frequency (of sound and light) and relative motion |
Jacque and Pierre Curie (French) | 1880–1881 | Discovered piezoelectric effect |
Lewis F. Richardson (British) | 1912 | British engineer after the Titanic disaster suggested that an echo technique could be used to detect underwater objects |
Paul Langevin (French) | WWI | Developed SONAR; used the piezoelectric effect to develop transmitters and receivers |
Sergei Sokolov (Russian) | 1929–1937 | Used reflection of ultrasound to detect flaws in metal |
Floyd Firestone (American) | 1942 | Flaw detection in metals |
* Lifespan of first five; date of development for last five.
The creation and recording of ultrasound were made possible by the discovery of the piezoelectric effect by the French brothers Jacques and Pierre Curie. In 1880, they discovered that when certain quartz crystals were subjected to mechanical stress (compression), they developed an electrical charge. A year later, in 1881, they observed the converse: When crystals were placed in an alternating electrical field, they would rapidly change shape (vibrate).9
After the Titanic disaster in 1912, the British engineer Lewis F. Richardson suggested that an echo technique could be used to detect underwater objects. In 1917, the Frenchman Paul Langevin (1872–1946) conceived of the idea to use piezoelectric quartz crystal as both transmitter and receiver of ultrasound, which culminated in the development of SONAR (sound navigation and ranging) used to detect enemy submarines. In 1937, the Soviet scientist Sergei Sokolov and, in 1942, the American engineer Floyd Firestone described the use of reflected ultrasound to detect flaws in metals that, as explained later, ultimately led to the medical use of ultrasound in cardiac medicine.11,13,14
Early medical use of ultrasound
The Austrian neurologist Karl T. Dussik (Figure 11-1), sometimes referred to as the “father of diagnostic ultrasound,”11 is credited with being the first to apply ultrasound for medical diagnosis16 (Table 11-2). In 1941, he reported on his use of transmission ultrasound to outline the ventricles of the brain.17 He considered use of echo reflection but abandoned this approach when his idea was ridiculed.11 In the 1940s, the German physicist W. D. Keidel used transmitted ultrasound through the chest (like X-rays) in an attempt to measure cardiac volumes.11,18 Subsequently, both investigators appear to have abandoned their efforts.
Early history of clinical echocardiography

Edler and Hertz and the Beginning of Clinical Echocardiography (Ultrasound Cardiography) and M-Mode Imaging
It was the collaboration between cardiologist Inge Edler (1911–2001) and physicist Carl Hellmuth Hertz (1920–1990) (Figure 11-2) at Lund University in Sweden in the early 1950s that is commonly accepted as the beginning of clinical echocardiography.13*

Figure 11-2 I. Edler (right) and C. H. Hertz (left) in 1979.
(From Roelandt JR: Seeing the invisible: A short history of cardiac ultrasound. Eur J Echocardiogr 1:8–11, 2000.)
In a search of a better way to evaluate the mitral valve function before closed mitral commissurotomy, Edler considered the use of something like RADAR (see Table 11-2). He was referred to a young physicist at Lund University, C. Hellmuth Hertz. Hertz was the son of a Nobel Prize winner, and, remarkably, his uncle, Heinrich Hertz, had lent his name to the unit of frequency. Hertz thought that ultrasound (frequencies higher than that audible to the human ear) might be the solution to Edler’s needs.14 Hertz borrowed an ultrasonic reflectoscope used in nondestructive testing of metals at the ship-building yard in Malmo for a weekend, and Elder tested it on Hertz’s chest and detected movement in the heart. The Siemens Corporation provided them with a reflectoscope in October 1953. They first studied isolated hearts in the laboratory with A-mode (static amplitude), but then devised a recording technique to display motion versus time (M-mode) by photographing the image off the oscilloscope (Figures 11-3 and 11-4). Their machine could produce ultrasound frequencies of 0.5, 1.0, 2.5, and 5.0 MHz, and Hertz opted to use 2.5 in humans as the optimal compromise between penetration and resolution. They first used this machine in a patient on October 29, 1953; they termed it “ultrasound cardiography” (UCG).19 At first they attributed the reflectors to the posterior wall of the left ventricle and the anterior wall of the left atrium, but, subsequently, Edler demonstrated that the latter came from the anterior leaflet of the mitral valve. He determined this by passing an ice pick in the comparable direction through the chest at the time of an autopsy on a patient he had echoed shortly before death.2 He subsequently used UCG to evaluate pericardial effusions and, in 1956, detected a left atrial myxoma (but this was not published until 1960).12 However, his principal use of UCG was to evaluate mitral stenosis, and he described the value of the E-F slope in quantifying the severity of the stenosis. In the mid-1950s, Edler and Hertz actually experimented with introducing a transducer into the esophagus to overcome the attenuation in lung tissue, but they abandoned this effort because of difficulties in obtaining acoustic coupling between the transducer and the esophageal wall.12 They published the first review article on UCG in Acta Medica Scandinavia in 1961.20 Hertz left the field of cardiac ultrasound fairly early after he developed ink-jet technology, and Edler made few innovations after 1960.2 In 1977, Edler and Hertz were awarded the Lasker Clinical Medicine Research Prize (often referred to as the American Nobel prize in Medicine).

Figure 11-3 The ultrasonoscope initially used by Edler and Hertz for recording their early echocardiograms.
(From Feigenbaum H, Armstrong WF, Ryan T: Feigenbaum’s echocardiography, 6th ed. Philadelphia: Lippincott Williams & Wilkins, 2005.)

Others Enter the Field
In the late 1950s, Schmidt and Braun, and Sven Effert in Germany separately began duplicating Elder and Hertz’s work, and in 1959, Effert published the first report identifying an intra-atrial tumor by echocardiography. Between 1961 and 1965, workers in Shanghai and Wuhan, China began to report their use of echocardiography including fetal echocardiography.13

The American Experience
In 1957, engineers John Wild and John Reid at the University of Minnesota described ultrasonic echocardiographic imaging of excised hearts.21 When Reid went to the University of Pennsylvania, he joined forces with cardiologist Claude Joyner, building an ultrasonoscope that they used to study mitral stenosis. Their report, which appeared in the journal Circulation in 1963, was the first American publication on clinical echocardiography.22 Harvey Feigenbaum in Indianapolis, author of one of the first (first published in 1972) and still published textbooks of echocardiography, first became interested in echocardiography in 1963 (Figure 11-5). He published his first article on use of ultrasound to diagnose pericardial effusions in 1965,23 and as mentioned later, he collaborated with Reggie Eggleton to develop a mechanical two-dimensional (2D) scanner. Dr. Feigenbaum also may have been the first to train nonphysicians to do echocardiograms, leading to the development of cardiac sonographers.13 Feigenbaum believes that Bernie Segal of Philadelphia was the first to use the term echocardiography in print in an article published in 1966.24,25 The senior author (E.A.H.) recalls cardiologists presenting their primitive, indistinct, and difficult to interpret M-mode images of the mitral valve at preoperative case conferences in the late 1960s and thinking that echocardiography did not have much future. How wrong he was!
Between the 1950s and the 1970s, M-mode was the only clinically useful format for echocardiography, and many advances and applications were described as the equipment and the skill of the echocardiographers improved, peaking in the late 1970s. But then its role rapidly declined with the development of 2D instrumentation.9

Two-Dimensional Scanners
Work to develop real-time 2D scanners began in the 1960s, made possible by advances in sonar and radar technology and circuitry. Early pioneering work was done by the previously mentioned Americans Wild and Reid,26 and Howry and Bliss27 in the 1950s. In the later 1960s, Ebina28 in Japan and Asberg29 reported producing ultrasound-generated tomographic images of thoracic structures in humans. Their clinical application was limited because of transducer size.
In 1968, Somer30 constructed the first electronic phased-array scanner based on the wavefront theory formulated in the 17th century by Huygens.11 In 1971, this was followed by the description by Nicholas Bom31 of Rotterdam of an electronic linear scanner (Figure 11-6) that generated a rectangular image and, in 1974, of an electronic phased-array scanner by F. L. Thurstone and O.T. von Ramm at Duke University.11,32 At the same time, J. Griffith and W. Henry, at the National Institutes of Health, introduced a mechanical sector scanner.11,33 This was said to be cumbersome to manipulate, and hence shortly thereafter Reggie Eggleton, working with Feigenbaum in Indianapolis, developed a handheld real-time mechanical 2D scanner34 that was more “user friendly” and commercially successful13 (Figure 11-7). In 1976 and 1977, Kisslo and colleagues35,36 at Duke University reported on the clinical use of the von Ramm and Thurston phased-array scanner for 2D echocardiography (2DE).

Figure 11-6 Twenty-element electronic linear two-dimensional transducer developed by N. Bom in Rotterdam circa 1972.
(From Feigenbaum H, Armstrong WF, Ryan T: Feigenbaum’s echocardiography, 6th ed. Philadelphia: Lippincott Williams & Wilkins, 2005.)

Development of Vascular and Cardiac Doppler
In 1956, Satomura reported the application of the Doppler principle in the use of ultrasound to measure blood flow velocity.11,37 However, in 1961, Franklin, at the University of Washington, was the first to measure Doppler shift of frequency, and hence velocity of flow of blood in vessels using a continuous-wave ultrasonic device.38 Pulse-wave Doppler (in which flow at a defined depth could be analyzed) was nearly simultaneously introduced in 1969 and 1970 by P. N. T. Wells of England, P. A. Peronneau of France, and D. W. Baker in the United States.11 In 1974, F. E. Barber, D. W. Baker, and colleagues at the University of Washington introduced the combination of pulse-wave Doppler with 2D scanning to produce the subsequently widely used “Duplex Scanner.”11,39 Subsequently, in Norway, but at different institutions, J. Holen40 and L. Hatle41 applied the Bernoulli principle to estimate the pressure decline across stenotic mitral and aortic valves, respectively.

Color-Flow (Doppler) Echocardiography
In 1978, the Swiss-born M. A. Brandestini, working at the University of Washington, described a method (based on pulse-wave Doppler) of color encoding flow velocity data and superimposing it on M-mode images.13 His initial observations were extended by Namekawa et al42 in Japan who described, in 1982, color Doppler flow imaging (CFI) utilizing autocorrelation to produce real-time images of flow in 2D sector scans. Bommer and Miller in the United States43 and Omoto working with the Namekawa group44 described clinical use of 2D color Doppler flow imaging.9,11

Prelude to Perioperative Transesophageal Echocardiography
The first reported use of intraoperative echocardiography (IOE) was in 1972 when Johnson and his colleagues45 at the University of Colorado reported on the use of intraoperative epicardial M-mode echocardiography to evaluate the results after open mitral commissurotomy. In 1976, Wexler and Pohost, of Massachusetts General Hospital, wrote a review article in the journal Anesthesiology on noninvasive techniques for hemodynamic monitoring, in which they described the basic principles and the potential application to anesthesia of the relatively new technique of “echocardiography.”46 At that time, this was limited to M-mode technology, but they anticipated that in the future 2D sector scanning would overcome some of the limitations of M-mode scanning. In that same year, at the annual meeting of the American Society of Anesthesiologists (ASA) in October in San Francisco, Rathod and colleagues,47 at Cook County Hospital and Loyola University in Chicago, and Paul Barash and colleagues,48 at Yale University, described use of transthoracic M-mode echocardiography to monitor the effects of anesthetics on cardiac function during anesthesia in 20 adults and 13 children, respectively. They concluded that this technique was a useful method to assess cardiac function, and Barash et al48 predicted that it “has the potential to be a useful tool for clinical anesthesiology that may supplant the invasive monitors currently available.”
In 1978, Strom and colleagues at Albert Einstein College of Medicine in Bronx, New York, published their experience with use of intraoperative epicardial M-Mode scanning during cardiac surgery,49 which led to their investigations of transesophageal echocardiography (TEE; see later).
When superior handheld 2D transducers became available, they began to be used epicardially during cardiac surgery after gas sterilization or wrapping them in sterile sheaths. In the early 1980s, Spotnitz and colleagues,51 at Columbia University College of Medicine in New York City (NYC), reported use of intraoperative epicardial 2D scanning after CPB to detect intracardiac air50 and to assess left ventricular ejection fraction before and after cardiac surgery. In 1984, Goldman and colleagues, at Mount Sinai Medical Center in NYC, reported the use of intraoperative epicardial contrast 2DE to evaluate regurgitation after mitral valve surgery52 and to assess myocardial perfusion during cardiac surgery.53
Transesophageal echocardiography
TEE has had a profound effect on the practice of cardiac anesthesiology starting in the mid 1980s, and conversely, at least in North America, anesthesiologists played a key role in the introduction of this modality into the practice of cardiac anesthesia and cardiology8 (Table 11-3).
TABLE 11-3 Notable Developments in Transesophageal Echocardiography
Investigator (Reference) | Year | Contribution |
---|---|---|
Side and Gosling60 | 1971 | Continuous-wave Doppler of the thoracic aorta mounted on a standard gastroscope |
Duck61 | 1974 | Pulse-wave Doppler of thoracic aortic blood flow with an esophageal probe |
Daigle62 | 1975 | Pulse-wave Doppler of thoracic aortic blood flow with an esophageal probe |
Frazin63 | 1976 | M-mode of heart via a cable-mounted transducer |
Matsumoto, Oka, et al64,65 | 1979–1980 | Transesophageal M-mode monitoring during cardiac surgery |
Hisanaga66 | 1977–1980 | Mechanical 2D scanner mounted on gastroscope |
DiMagna67 | 1980 | Electronic linear phased-array 2D scanner mounted on an endoscope mainly used to examine the gastrointestinal tract |
Souquet68 | 1980–1982 | Electronic phased-array 2D scanner mounted on gastroscope |
Schulter, Hanrath, Sorquet69 | 1980–1982 | Clinical evaluation of Sorquet’s TEE probe |
Goldman et al93 | 1976 | TEE with color-flow Doppler |
Takamoto et al94 | 1987 | TEE with color-flow Doppler |
deBruijn et al95 | 1987 | TEE with color-flow Doppler |
Omoto98; and others | ~1989 | Biplane probe |
Roelandt100; and others | ~1992 | Multiplane probe |
2D, two-dimensional; TEE, transesophageal echocardiography.

Intravascular Ultrasound
The development of TEE had its roots in the search for alternative ultrasound windows because of difficulties encountered in obtaining good ultrasound signals through the chest using the early insensitive transthoracic transducers.7 In the early 1960s, this led to the investigation of intravascular probes. In 1960, Cieszynski54 inserted a single-element transducer on a catheter into the jugular vein of dogs. In 1963, Omoto et al55 reported obtaining static cross-sectional images in patients by slowly rotating a single-element transducer inserted into the right atrium,7 and a year later, Kimoto et al56 reported obtaining C-scans of the atrial septum in humans from an intravascular catheter.9 In 1968, Carleton and Clark57 reported similar studies. 1970, Reggie Eggleton58 mounted four elements on a catheter and created the first cross-sectional images of intracardiac structures by computer reconstruction of images obtained by slow rotation and ECG triggering,5 and in 1972, Nicholas Bom,59 in Rotterdam, described a real-time intra- cardiac scanner using a 32-element circular electronically phased-array transducer placed at the tip of a 9 F-catheter. Thereafter, interest faded as sophisticated TEE probes became available, only to re-emerge in the late 1980s (see later).

Transesophageal Ultrasound (Doppler and M-Mode)
Side and Gosling60 were the first to use transesophageal (continuous-wave) Doppler (transducer mounted on a steerable gastroscope) to assess flow in the heart and aorta in 1971. This was followed by similar reports by Olson and Shelton in 1972. In 1974 and 1975, Duck et al61 and Daigle et al62 used transesophageal pulsed Doppler to measure flow in the thoracic aorta.7 However, true TEE is said to have its embryonic beginnings in 1976 when Lee Frazin (a Chicago cardiologist) et al63 reported recordings of M-mode echocardiograms of the aortic root and valve, mitral valve, and left atrium from the esophagus by attaching a nonfocused 3.5-mHz transducer attached to a 3-mm coaxial cable (Figure 11-8). They reported superior recordings compared with the transthoracic approach in 38 patients.63 Following this lead, Matsumoto and Oka,64 at Albert Einstein College of Medicine, fabricated a stiff transesophageal probe supporting an M-mode transducer (Figure 11-9). They first used this in 1979 to measure ventricular dimensions and volume during mitral valve surgery in a 65-year-old woman.64 They subsequently reported intraoperative transesophageal M-mode monitoring in 21 patients undergoing cardiac surgery.65 At that time, M-mode TEE was difficult to interpret except by the extremely sophisticated clinician and hence was not widely adopted. However, the development of a new generation of gastroscopes with steerable tips in the late 1960s, onto which echo transducers could be mounted, had a significant positive impact on the development of TEE by facilitating direct contact with the wall of the esophagus,7 overcoming the problem encountered by Edler and Hertz a decade earlier.

Transesophageal Two-Dimensional Imaging
Although some primitive 2D TEE mechanical scanners and linear were described between 1977 and 1980 (e.g., Hisanaga et al66 and DiMagno et al67), it was not until Jacques Souquet68 (Figure 11-10), working in conjunction with the Varian Corporation, developed their phased-array transducer (Figure 11-11) mounted on the end of a gastroscope for their 2D sector scanner system (Figures 11-12 and 11-13) that TEE became a practical reality. These new TEE probes were evaluated clinically by cardiologists Michael Schluter and Peter Hanrath in Hamburg, Germany,69 and their preliminary results were highlighted at an international conference held in Hamburg in 1981.8 The early subsequent use of TEE took different directions in the United States and Europe. Cardiologists in Germany and the Netherlands rapidly began using TEE in awake patients to aid in the diagnosis of a variety of cardiac pathologies. In the United States, cardiologists seemed reluctant to adopt this new technology.6 In the early 1980s, Peter Hanrath sent prototypes (first M-mode transducers and then with the 2D transducers mounted on gastroscopes) to cardiologists James Seward at Mayo Clinic and Nelson Schiller (Figure 11-14) at the University of California in San Francisco (UCSF), who passed them on to anesthesiologists. Schiller70 spoke with William Hamilton, Chair of the Department of Anesthesiology at UCSF, who put him in contact with two young faculty members, Michael Cahalan and Michael Roizen. At that time (1981–1983), a cardiology fellow from Hanrath’s group in Germany, Peter Kremer (Figure 11-15), came to UCSF and collaborated with Cahalan (Figure 11-16) and Roizen to investigate these new TEE instruments. At the 1982 annual meeting of the ASA, they started the American TEE “revolution” when they presented their results in monitoring cardiac and vascular surgery patients with this new TEE probe, displaying the high-quality recordings and images obtained (Figures 11-17 and 11-18), and describing its usefulness in assessing filling and function of the left ventricle, and in detecting myocardial ischemia and intracardiac air.71,72 Subsequently, they reported its superiority to PAC in assessing filling in the operating room73 and usefulness in evaluating the hemodynamic changes during anaphylaxis74 and during surgery for pheochromocytoma.75 Topol and colleagues at Johns Hopkins University demonstrated its usefulness in determining the cause of hypotension immediately after CPB76 and documented improvement in myocardial dysfunction immediately after coronary revascularization.77 Cucchiara et al,78 at the Mayo Clinic, described its usefulness in detecting air embolism during neurosurgery, whereas the UCSF group described its value in monitoring during vascular surgery79,80 and its superiority over ECG in detecting myocardial ischemia.81 In 1984, the Hamburg group and Cahalan published an anatomic analysis of six standard TEE views,82 which was expanded on a few years later by the Mayo Clinic group.83 Meanwhile, the group at Mount Sinai Medical Center in NYC (Martin Goldman, Joel Kaplan, Daniel Thys, Zaharia Hillel, and Steven Konstadt) was quick to adopt and evaluate perioperative TEE, following the experience of Kaplan working with the early Diasonic TEE probes in the early 1980s.84–88 In 1987, Cahalan et al89 and Clements and deBruijn90 at Duke University wrote review articles on the use of TEE in anesthesiology, whereas the group at Mayo Clinic reported on the reproducibility of measurements obtained during intraoperative TEE.91 In that same year (1987), the second edition of this text edited by Kaplan on Cardiac Anesthesia contained for the first time a 63-page thoroughly referenced chapter on Intraoperative Echocardiography.92

(From Image: A Conversation with…Jacques Souquet, PhD. Available at: http://www.rt-image.com/A_Conversation_with_Jacques_Souquet_PhD_The_diagnostic_capabilities_of_ShearWave/content=8504J05E48BE588440B6967644A0B0441.)

Figure 11-16 Michael K. Cahalan, Professor Anesthesiology, University of Utah.
(Photograph courtesy M. K. Cahalan.)
In 1986, Goldman and colleagues,93 at Mount Sinai Medical Center in NYC, reported their experience with a new 2D TEE probe that also provided Doppler color-flow imaging (Aloka and Irex), as did a Japanese group.94 That same year, the Hewlett-Packard Corporation also introduced a color-flow Doppler TEE probe; and in 1987, deBruijn and Clements, together with pioneering echocardiographer, Joseph Kisslo at Duke University, reviewed their early experience with this new technology.95 In that same year, pulse-wave Doppler was added to 2D TEE probes,96 although several years earlier the Hamburg group had described results of use of a TEE probe that combined pulse-wave Doppler with M-mode TEE.97 In 1989, biplane probes (Figure 11-19) first became available,98 although prototypes had been described earlier,99 and soon thereafter, multiplane single-transducer TEE probes100 and smaller diameter probes suitable for pediatric use101 appeared. In 1992, Pandian and colleagues, at Tufts-New England Medical Center, reviewed their early clinical experience with a 5-MHz phased-array multiplane TEE probe (“OmniPlane”).102
A survey conducted of Society of Cardiovascular Anesthesiologists (SCA) members in early 1988 provides a “snap-shot” of the state of practice of IOE at that time103; 20% of the responders reported that IOE studies were being performed at their institutions. The majority of responders were practicing in large teaching hospitals. Anesthesiologists were involved 41% of the time and cardiologists in 33%, although in one third of the former group, cardiologists always assisted. TEE technique was used in 51%, epicardial in 37%, and transthoracic in 22% of cases. At the time of the survey, six manufactures made 2D TEE transducers—Hewlett-Packard, Aloka Toshiba, Diasonics, General Electric, Acusonics, and Hoffrel, but only the first three provide color-flow TEE. Most responders reported using either Diasonics (46%) or Hewlett-Packard (42%); 90% used the 2D mode, 75% M-mode, 60% pulsed-Doppler, 58% color-flow Doppler, and only 23% continuous-wave Doppler, whereas 97% believed that IOE had been or could have been helpful. The authors of the survey noted that few academic medical centers offered formal instruction and certification in IOE, and that a definite need for training had been identified.
Along this same line, in an editorial published in 1989, Kaplan104 urged caution and restraint in the adoption of this new and complex technology that had the potential to cause numerous complications, and emphasized the need for anesthesiologists who aspire to be echocardiographers to obtain a high level of training.
In the late 1980s, the group at Duke University demonstrated the utility of routine epicardial echocardiography during surgery for congenital heart disease.105
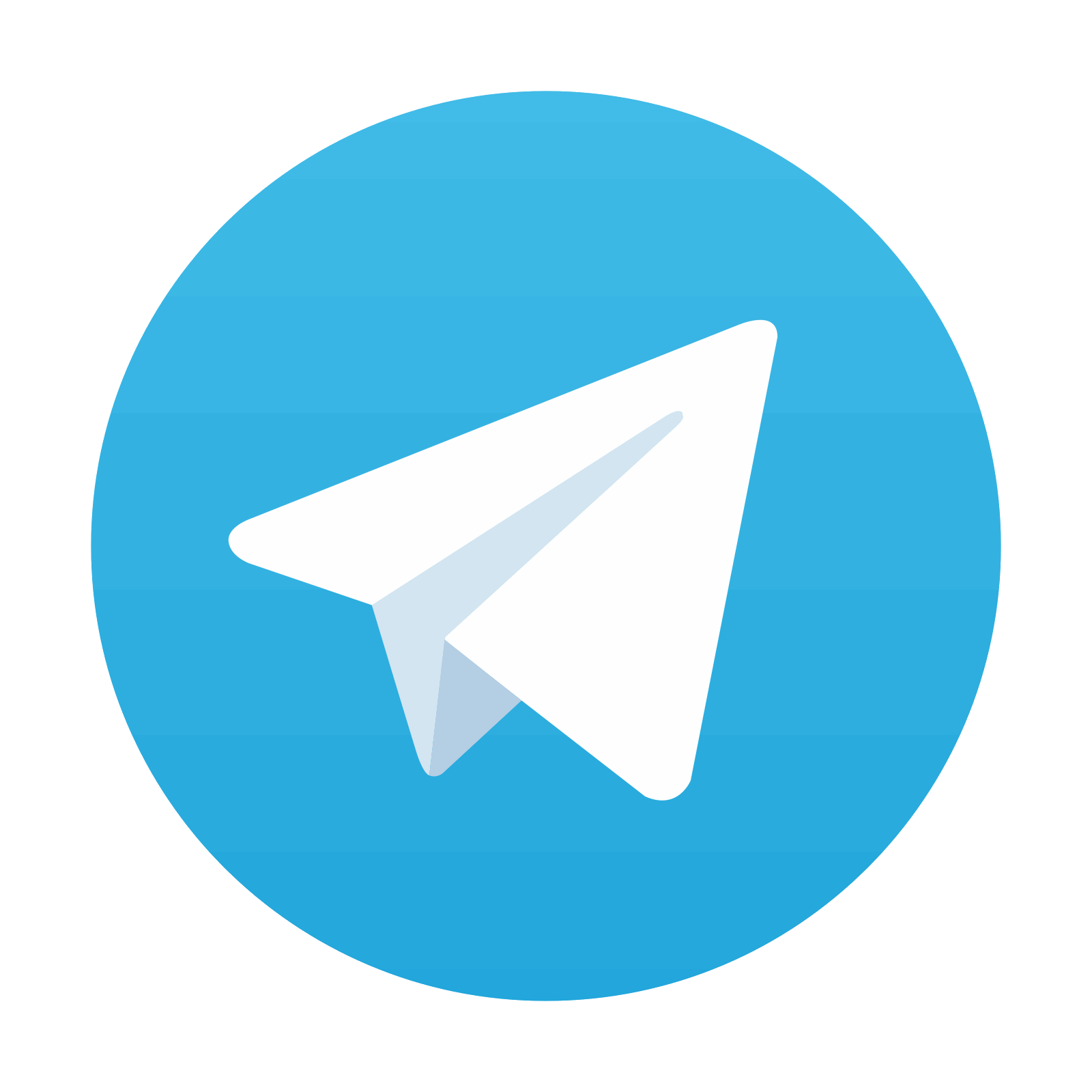
Stay updated, free articles. Join our Telegram channel

Full access? Get Clinical Tree
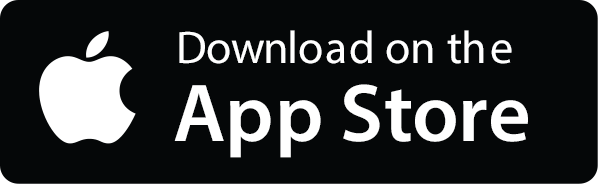
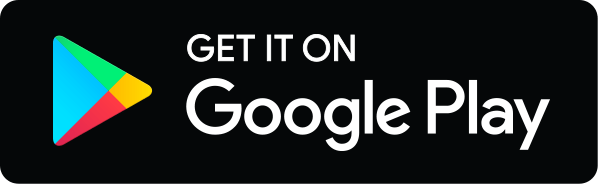