ENVIRONMENTAL EMERGENCIES, RADIOLOGICAL EMERGENCIES, BITES AND STINGS
DESIREE M. SEEYAVE, MBBS AND KATHLEEN M. BROWN, MD
RELATED CHAPTERS
Resuscitation and Stabilization
• Cardiopulmonary Resuscitation: Chapter 4
Signs and Symptoms
Clinical Pathways
Medical, Surgical, and Trauma Emergencies
• Cardiac Emergencies: Chapter 94
• Renal and Electrolyte Emergencies: Chapter 108
DROWNING
Goals of Treatment
Treatment of submersion injuries aims to resuscitate and stabilize those who need it, and prevent further pulmonary and neurologic deterioration.
CLINICAL PEARLS AND PITFALLS
• Outcome of submersion victims depends on duration of submersion, degree of pulmonary damage by aspiration, and effectiveness of initial resuscitative measures.
• Supplemental O2 should be given to all victims of submersion, regardless of neurologic or pulmonary function on presentation.
Current Evidence
Drowning is defined as water submersion with resultant asphyxiation and death within 24 hours; near drowning implies that resuscitation has extended survival beyond 24 hours. Each term is further classified according to whether aspiration has occurred.
Drowning affects all age groups and males two to three times more often than females. Individuals with epilepsy have a 15- to 19-fold higher risk of drowning when compared to the general population. It is the major cause of death following water-related natural disasters such as Hurricane Katrina.
Drowning is the second leading cause of injury death in children 1 to 14 years of age. Older infants and toddlers are disproportionately represented in these accidents, with lower survival rates. They are vulnerable to immersion in household buckets, baths, hot tubs, swimming pools, and other bodies of water near their homes. Young teenagers are also at greater risk because adult supervision decreases and impulsive behavior increases. However, coexisting trauma, drug or alcohol use, and suicidal intent must be considered in each case.
Fresh water aspirated into the lungs is rapidly taken up into the circulation, resulting in a transient rise in circulating blood volume and hemodilution. Salt-water aspiration causes decreased intravascular volume and hemoconcentration. Occasionally massive hemolysis may occur. Electrolyte abnormalities that occur after massive aspiration in laboratory animals rarely achieve clinical significance in human victims.
Even small (1 to 3 mL per kg) quantities of fresh water cause disruption of surfactant, a rise in surface tension in the lungs, and alveolar instability. Capillary and alveolar membrane damage allows fluid to leak into the alveoli, with subsequent pulmonary edema. Aspiration of salt water (osmolality greater than normal saline) does not denature surfactant but creates an osmotic gradient for fluid to accumulate in the lungs, which dilutes surfactant.
Both fresh- and salt-water aspiration decrease pulmonary compliance, increase airway resistance and pulmonary artery pressure, and diminish pulmonary flow. As nonventilated alveoli are perfused, an intrapulmonary shunt develops, leading to a drastic fall in partial pressure of arterial oxygen (Pao2). Tissue hypoxia then leads to severe metabolic acidosis. The victim is usually able to correct a rise in partial pressure of arterial carbon dioxide (Paco2). Aspiration of bacteria, gastric contents, and foreign materials may cause additional trauma to the lungs.
Hypoxemia results in loss of consciousness. If anoxia ensues, irreversible central nervous system (CNS) damage begins after 4 to 6 minutes. Fear or cold may trigger the diving reflex (commonly encountered in infancy), which shunts blood to the brain and heart primarily and affords several minutes of additional perfusion. Cold water is relatively protective of the CNS, but probably only if immersion hypothermia develops very rapidly or before compromise of oxygenation. Hypothermia is more rapid in the victim who is younger (because of greater surface area:volume ratio) or is struggling in or swallowing icy water. If laryngospasm or aspiration occurs before a fall in core body temperature and cerebral metabolic rate, protection is probably minimal.
Cardiovascular effects are primarily those expected with myocardial ischemia, severe systemic acidosis, hypothermia, and intravascular volume changes. After aspiration of fresh water, the transient rise in intravascular volume later contributes to problems of cerebral and pulmonary edema.
Clinical Recognition
In the first moments after rescue, the appearance of the child who has nearly drowned may range from apparently normal to apparently dead. Body temperature is often low, even in warm water. Respiratory efforts may be absent, irregular, or labored, with pallor or cyanosis, retractions, grunting, and cough productive of pink, frothy material. The lungs may be clear, or there may be rales, rhonchi, and wheezing. Infection may develop as a consequence of aspirated mouth flora or organisms in stagnant water, but this is not usually important in the first 24 hours.
Respiratory function may improve spontaneously or deteriorate rapidly as pulmonary edema and small airway dysfunction worsen. Deterioration may also occur slowly over 12 to 24 hours. Intense peripheral vasoconstriction and myocardial depression may produce apparent or actual pulselessness.
The child may be alert and normal or have any level of CNS compromise. Superficial evidence of head trauma may be noted if the submersion episode was a secondary event. Head CT most typically shows diffuse loss of gray–white differentiation and/or bilateral basal ganglia edema/infarction.
Triage Considerations
Outcome depends on the duration of submersion, the degree of pulmonary damage by aspiration, and effectiveness of initial resuscitative measures. Most children are salvageable, and all should receive the benefit of excellent cardiopulmonary resuscitation without delay at the scene. They should all be given 100% oxygen, even if rescued with spontaneous ventilation and minimal or no neurologic dysfunction, to minimize the risk of progressive hypoxemia and acidosis with secondary myocardial and cerebral damage. Physical examination is notoriously insensitive to hypoxemia; a seriously hypoxemic child may be alert and talking. Once the child has arrived at an emergency facility (and cardiovascular stability is achieved), pulmonary and neurologic assessment should guide further treatment.
Initial Assessment
Initial assessment should focus on signs of neurologic, respiratory, and hemodynamic compromise. One prospective study devised a prediction rule for children submerged in nonicy water who presented to the emergency department (ED) in a comatose state: Lack of pupillary light reflex, male gender, and hyperglycemia were variables used to predict unfavorable outcome (vegetative state or death). A retrospective study of children presenting to the ED after warm-water submersion suggested that hemodynamic, rather than neurologic, status was more highly predictive of poor neurologic outcome.
More recent studies indicate that patients with asystole on arrival in the ED have uniformly poor neurologic outcomes. The emergency physician may reasonably discontinue resuscitative efforts after consideration on a case-by-case basis.
Management and Diagnostic Testing
Effective therapy of drowning depends on the reversal of hypoxemia and metabolic acidosis. The pulmonary status is assessed initially with a chest radiograph (Fig. 98.1) and with measurement of arterial oxygen saturation (Sao2) and arterial blood gas (ABG), as in Figure 98.2. If oxygenation is normal on breathing room air, the child can be assumed to have suffered drowning without aspiration. Observation for 6 to 24 hours with repeat Sao2 or ABG determination should be sufficient to assess the possibility of late deterioration in gas exchange.
FIGURE 98.1 Drowning in a 4-month-old girl. A: There is bilateral disseminated alveolar pattern, more on the left than on the right, consistent with the pulmonary edema of drowning. This change may be the result of neurologic pulmonary edema rather than aspirated water. B: Two days later, the patient has been extubated and there is marked improvement in appearance of pulmonary edema secondary to drowning. (Courtesy of Soroosh Mahboubi, MD.)
Other initial laboratory evaluation should include complete blood cell count (CBC), electrolytes, and urinalysis. Patients with abnormalities of gas exchange with normal chest radiographs can usually be managed with supplemental oxygen and pulmonary physiotherapy. Noninvasive ventilatory support, such as high-flow humidified nasal cannula oxygen or CPAP, may be beneficial. Any change in mental status or increase in respiratory distress may reflect arterial hypoxemia and should also prompt a repeat ABG determination. Continuous Sao2 or serial Pao2 measurements will guide the physician to continue conservative treatment or to intensify ventilatory support.
Patients with obvious respiratory distress, hypoxemia (Sao2 less than 90% or Pao2 less than 60 on 60% inspired oxygen), and extensive pulmonary edema or infiltration generally require more vigorous treatment. All should be monitored for heart rate, cardiac rhythm, respiratory rate, and blood pressure (BP). Most will require frequent blood gas analysis and may be more easily monitored through arterial cannulation. Intubation, supplemental oxygen, and mechanical ventilation with positive end-expiratory pressure (PEEP; 5 to 15 cm H2O) should be provided, as indicated.
FIGURE 98.2 Algorithm for pulmonary assessment for drowning.
Once BP is stabilized, fluid restriction (to approximately one-half the maintenance rate) and diuretic therapy (e.g., furosemide 0.5 to 1 mg per kg intravenously, usual maximum 20 mg per dose) may improve gas exchange. In the setting of extensive pulmonary damage, pulmonary and cardiovascular components of the disease are intimately entwined. Optimum management requires monitoring of blood gases and systemic arterial pressure.
Patients who have experienced significant hypoxemia and are not able to be aroused, can be further divided into three subcategories according to neurologic findings: (i) Those with decorticate response to pain and Cheyne–Stokes breathing, (ii) those with decerebrate response to pain and central hyperventilation, and (iii) those who are flaccid with fixed, dilated pupils and apneustic breathing or apnea. Again, reversal of hypoxemia and acidosis is critical, as well as fluid resuscitation and avoidance of hyperglycemia. Avoiding hypercapnia and resultant cerebral hyperemia is generally accepted, but hyperventilation, barbiturate coma, and other measures initially believed to provide cerebral protection and prevent or treat elevated intracranial pressure have not been helpful in these patients.
Hypothermia does appear to have some protective effect. Extreme hypothermia should be corrected to at least 32°C (89.6°F) to achieve hemodynamic stability and to minimize the risk of infection. The child should then be allowed to rewarm passively. Although data in humans are limited, animal studies suggest that maintenance of mild brain hypothermia may minimize reperfusion injury. Hyperthermia, a common result of active rewarming, should be avoided.
There is no benefit to prophylactic antibiotics, which should be reserved for strongly suspected or proven bacterial infection. Exceptions are when grossly contaminated water is aspirated or when maximal ventilatory support is required to provide any margin for survival. Bronchoalveolar lavage and steroids have no demonstrated benefit.
Renal function, normal electrolytes, and an adequate hemoglobin level (more than 10 g per 100 mL) should be maintained. If significant hemoglobinuria exists, diuresis is required.
Indications for Discharge or Admission
The patient’s clinical condition in the ED dictates further management and may provide prognostic clues. Patients may be assigned to one of three groups: alert, blunted, or comatose (Table 98.1). Patients in the first group should be observed in the ED (as above). If they maintain normal oxygenation and normal work of breathing they can be discharged to home. Patients in the second group should be admitted for careful monitoring.
Patients in the third group have experienced severe CNS asphyxia. The prognosis for this group includes a much greater risk of death or severe anoxic/ischemic encephalopathy. Risk increases with depth of coma on presentation. Patients in the third subdivision (flaccid with fixed, dilated pupils) rarely survive intact regardless of treatment, although coexistent hypothermia has provided some remarkable exceptions.
SMOKE INHALATION
Goals of Treatment
The goals of emergency care include early intubation (if there are signs of airway compromise), stabilization of respiratory and cardiovascular status, and maintenance of fluid and electrolyte balance. One must recognize concomitant carbon monoxide or cyanide poisoning or other inhalants that contribute to morbidity.
TABLE 98.1
NEUROLOGIC ASSESSMENT AFTER DROWNING
CLINICAL PEARLS AND PITFALLS
• Early intubation should be done with any evidence of airway burns or edema.
• Severe smoke inhalation can occur without cutaneous burns.
• Respiratory status, and not evidence of airway burns or labs, should guide treatment.
Current Evidence
Respiratory complications of smoke inhalation rank with carbon monoxide poisoning as a major cause of early death from fire. Although serious cutaneous injury may occur in the absence of pulmonary involvement, inhalation injury dramatically increases the morbidity and mortality associated with any given percent body surface area burn.
The severity of carbon monoxide inhalation and respiratory problems is related to the duration of exposure, the occurrence in a closed space (more likely in very young or elderly victims), the nature of materials involved, and the presence of products of incomplete combustion. Severe hypovolemic shock, massive tissue destruction, extensive fluid resuscitation, and infection further complicate direct inhalational trauma.
The relatively low heat capacity of dry air and the excellent heat exchange properties of the nasopharynx usually limit direct thermal injury to the upper airway. Dry air above 160°C (320°F) has little effect on the lower airway. The greater heat capacity of steam increases the risk of lower airway damage. In addition, continuing combustion of soot particles carried deeply into the lung may exacerbate thermal injury.
Chemical injury may occur at any level of the respiratory tract. Oxides of sulfur and nitrogen combine with lung water to form corrosive acids. Incomplete combustion of any carbon-containing material, such as wood, may produce carbon monoxide. Combustion of cotton or plastic generates aldehydes that cause protein denaturation and cellular damage. Burning polyurethane may produce cyanide gas. Fire retardants that contain phosphorus may actually produce phosgene gas. The upper airway filters most soot particles, but those carried into the lung may adsorb various substances and cause reflex bronchospasm to further extend chemical damage.
Immediate effects of smoke inhalation on the lower airway include loss of ciliary action, mucosal edema, bronchiolitis, alveolar epithelial damage, and impaired gas exchange, particularly oxygenation. Areas of atelectasis or air trapping, and loss of surfactant activity, worsen ventilation–perfusion mismatch and hypoxemia. Hours later, sloughing of tracheobronchial mucosa and mucopurulent membrane formation increases the degree of obstruction, poor gas exchange, and likelihood of infection. Beyond the first 24 hours, pulmonary pathology that results from smoke inhalation is largely indistinguishable from adult respiratory distress syndrome, which arises from other insults. Children who die from smoke inhalation may sustain serious respiratory damage in the absence of cutaneous injury.
Clinical Recognition
A history of exposure in a closed space should heighten concern for smoke inhalation. Need for CPR at the site implies significant carbon monoxide poisoning and/or hypoxia secondary to decreased ambient oxygen concentration or severe respiratory disease. The physician should also consider the types of material involved to determine the risk of poisoning from carbon monoxide or other toxins.
Physical examination that reveals facial burns, singed nasal hairs, pharyngeal soot, or carbonaceous sputum justifies a presumption of smoke inhalation. Any sign of neurologic dysfunction, including irritability or depression, should be presumed related to tissue hypoxia until proven otherwise. Signs of respiratory dysfunction, including tachypnea, cough, hoarseness, stridor, decreased breath sounds, wheezing, rhonchi, or rales may be detected on presentation or may be delayed for 12 to 24 hours, depending on the severity of the insult.
Auscultatory findings often precede chest radiograph abnormalities by 12 to 24 hours. Radiographic changes may include diffuse interstitial infiltration or local areas of atelectasis and edema (Fig. 98.3). Acute respiratory failure may occur at any point. ABG analysis provides the ultimate assessment of effective respiratory function. Bronchoscopy can document the extent of inhalation injury and help remove debris but may worsen airway edema. In general, it is respiratory function, not the appearance of lesions, that guides supportive care; therefore, most patients can be treated effectively without bronchoscopy.
FIGURE 98.3 Smoke inhalation in a 9-year-old girl. A: There is bilateral central alveolar process consistent with acute smoke inhalation. B: A day later, the patient has been extubated and there is marked improvement in the appearance of pulmonary edema. (Courtesy of Soroosh Mahboubi, MD.)
Triage Considerations
Initial assessment and resuscitation at the scene should proceed according to the principles outlined in Chapter 1 A General Approach to Ill and Injured Children. Because of the likelihood of carbon monoxide exposure and the difficulty of assessing hypoxemia clinically, all victims should receive the maximum concentration of inspired oxygen possible in transport and in the ED until further evaluation is complete (Table 98.2).
Clinical Assessment
In the ED, assessment of airway and respiratory functions must proceed simultaneously with cardiovascular stabilization. Thermal injury to the nose, mouth, or face, or compromise of the upper airway (stridor, hoarseness, barking cough, retractions, delayed inspiration, or difficulty handling secretions) indicates the need for direct laryngoscopy. The presence of significant pharyngeal, supraglottic, or glottic edema mandates elective endotracheal intubation. Worsening edema over 24 hours may lead to respiratory arrest and a difficult emergency intubation through a distorted airway, and thus early endotracheal intubation is indicated. Elective tracheostomy may be considered if placing or securing the endotracheal tube will further traumatize an edematous airway or severe facial burns. However, in the presence of extensive cutaneous burns, tracheostomy dramatically increases the risk of systemic and pulmonary infection.
TABLE 98.2
MANAGEMENT OF SMOKE INHALATION
Management and Diagnostic Studies
The details of burn therapy are elaborated in Chapter 112 Burns but, in general, the goals are stabilization of cardiovascular function without fluid overload and compromise of gas exchange. Pulse rate and BP should guide administration of fluid volume. Maintenance of urine output of at least 0.5 mL/kg/hr should provide adequate tissue perfusion. Careful monitoring of renal and cardiovascular systems may prevent or minimize acute pulmonary edema and delayed pulmonary dysfunction secondary to late fluid mobilization and infection.
Oxygen saturation and serial blood gas determinations should be obtained to guide oxygen supplementation and to assess adequacy of ventilation. Intubation is indicated if adequate oxygenation (Sao2 greater than 90% or Pao2 greater than 60 mm Hg) cannot be maintained with an inspired oxygen concentration of 40% to 60%, if Paco2 rises above 50 mm Hg, or if the work of breathing appears unsustainable. Spontaneous ventilation with continuous positive airway pressure causes less cardiovascular interference; this patient should receive humidified gas mixtures and be encouraged to take deep breaths and cough frequently. If there is severe CNS depression or severe pulmonary parenchymal damage, mechanical ventilation with PEEP will likely be necessary. Maximally humidified oxygen should be delivered by mask or artificial airway to prevent inspissation of debris and occlusion of the airway. If intubation is necessary, meticulous pulmonary toilette is essential, with frequent suctioning to remove edema fluid, mucus, and sloughed epithelium that may otherwise occlude the endotracheal tube.
High-frequency percussive ventilation (hi-fi) is superior to conventional mechanical ventilation in reducing work of breathing. There is also evidence that extracorporeal membrane oxygenation (ECMO) may be useful in severely burned children.
After the first few hours, diuretic therapy (furosemide 0.5 to 1 mg per kg intravenously), within the limits of cardiovascular stability, may also improve oxygenation and pulmonary compliance, leading to more effective ventilation. Chemical and particulate irritation of upper airway receptors may cause reflex bronchoconstriction and contribute to lower airway obstruction. Bronchodilators such as nebulized albuterol may help reverse bronchospasm, but relief depends mostly on removal of secretions and debris from the respiratory tree.
Studies have not demonstrated a role for steroids or prophylactic antibiotics. When steroids are used, there is evidence that sodium and fluid retention increase, healing is delayed, and bacterial clearance from the lung is decreased.
One study in children has demonstrated some benefit to aerosolized heparin and N-acetylcysteine in decreasing the incidence of atelectasis, reintubation, and overall mortality. Whole-body hypothermia has been shown to be effective in controlling bronchoalveolar damage in animal models.
Indications for Discharge or Admission
Determination of disposition from the ED will depend on the history and clinical status. Patients with significant respiratory distress or decreased neurologic status should be admitted to an intensive care unit (ICU). Patients who appear well on presentation to the ED but have a significant history of exposure or physical evidence of significant smoke inhalation should be monitored closely for at least 6 hours for signs of respiratory distress or significant cough.
CARBON MONOXIDE POISONING
Goals of Treatment
The goals of treatment are immediate removal from the source of contamination, provision of 100% oxygen to foster elimination, and treatment/prevention of tissue hypoxia, metabolic acidosis, and cerebral edema.
CLINICAL PEARL AND PITFALLS
• Carbon monoxide poisoning may occur in spaces with improper ventilation, even with doors and windows open.
• The classically described “cherry red” skin color is unreliable in diagnosis.
• Pao2 and pulse oximetry arterial saturation (Sao2) are likely to be normal in carbon monoxide intoxication.
Current Evidence
Unintentional carbon monoxide poisoning claims approximately 500 lives per year in the United States and is largely responsible for early deaths related to fire. Exposure may occur in a variety of other settings unrelated to accidental fires, including incomplete combustion of any carbon-containing fuel (i.e., propane-powered forklifts), improperly vented wood- or coal-burning stoves, and automobile exhaust in garages, even with doors and windows open. Passengers may be poisoned in vehicles or boats with open backs, or with faulty or blocked exhaust systems.
In the normal person, carboxyhemoglobin levels are less than 1%. In smokers, levels of 5% to 10% are common. Inhaled carbon monoxide has two important effects that cause tissue hypoxia: (i) It binds to hemoglobin with an affinity 200 to 300 times greater than that of oxygen, and (ii) it shifts the oxyhemoglobin dissociation curve to the left and changes the shape from sigmoidal to hyperbolic (Fig. 98.4). The first effect decreases oxygen content of the blood, whereas the second causes oxygen release at lower-than-normal tissue oxygen levels. Other endogenous (anemia) and exogenous (high altitude, or displacement of ambient oxygen during fires) factors contribute further to hypoxia. Although oxygen content of the blood is low, the Pao2 remains normal. Because carotid body receptors respond to Pao2, respiration may not be stimulated until late, when metabolic acidosis activates other centers. Tissue hypoxia increases cerebral blood flow, cerebrospinal fluid pressure, and cerebral capillary permeability, which predispose the patient to cerebral edema.
FIGURE 98.4 Carbon monoxide shifts the oxyhemoglobin dissociation curve to the left and changes its shape, making the unloading of oxygen in the tissues more difficult and provides an inadequate diffusion gradient. (Left curve, P50 >12 mm; right curve, P50 >28 mm.)
Carbon monoxide interacts with several other cellular proteins, including cytochrome oxidase. It appears to interfere with oxidative energy production and may generate free radicals, which exacerbate CNS dysfunction. Neuronal necrosis, as well as apoptosis, is seen in animal models in the frontal cortex, globus pallidus, and cerebellum, likely contributing to delayed cognitive effects such as deficits in learning, memory, and dementia. Carbon monoxide also binds to myoglobin; individuals, particularly with pre-existing coronary disease, may develop cardiac ischemia and/or dysrhythmias.
Clinical Recognition
History provides the most valuable clue to diagnosis. Carbon monoxide poisoning should be suspected in all fire victims and considered in children exposed to other hazards noted earlier. Presence or absence of the classically described “cherry red” skin color is of no diagnostic value. In fact, patients with thermal injury may appear red, whereas those with vasoconstriction may be quite pale. Both color and respiratory rate may be deceptive and may lead the physician away from recognition of severe tissue hypoxia. Pao2 and arterial saturation as determined by pulse oximetry (Sao2) are likely to be normal in carbon monoxide intoxication; low values reflect coexistent pulmonary dysfunction.
Triage Considerations
Most important is removal of the victim from the contaminated environment. Resuscitation should proceed according to general principles. As soon as possible, the patient suspected of suffering carbon monoxide poisoning should be provided 100% oxygen to hasten elimination.
Clinical Assessment
Determination of blood levels of carboxyhemoglobin may help aid in diagnosis and prognosis. Spectrophotometric methods are most widely used clinically. Venous blood may be used because of the high affinity of carbon monoxide for hemoglobin, but an arterial sample provides more precise information about acid–base balance and adequacy of ventilation. The level of hemoglobin should also be determined.
Levels of carboxyhemoglobin as low as 5% in nonsmokers may impair judgment and fine motor skills. Mild intoxication (20% carboxyhemoglobin) produces headache, mild dyspnea, visual changes, and confusion. Moderate poisoning (20% to 40%) produces drowsiness, faintness, nausea and vomiting, tachycardia, dulled sensation, and decreased awareness of danger. At lower levels, these symptoms are noted only with exertion, but as the fraction approaches 40%, they are present at rest. Between 40% and 60%, weakness, incoordination, and loss of recent memory occur, and cardiovascular and neurologic collapse is imminent. Above 60%, coma, convulsions, and death are almost certain. Although carboxyhemoglobin levels and symptoms tend to follow the pattern just described, individual patients may be more or less symptomatic than predicted. An important caveat is that blood carboxyhemoglobin levels will fall rapidly with time and may not reflect cellular dysfunction, especially in high-demand tissues of the heart and CNS.
Patients with severe poisoning are vulnerable to pressure trauma to skin, subcutaneous tissue, and muscle, especially at sites that support body weight or that are pinned under fallen objects. The history may suggest which sites are most vulnerable, and pain is an early symptom. Muscle breakdown and myoglobin deposition in renal tubular cells may precipitate acute renal failure.
A syndrome of delayed neuropsychologic sequelae (DNS) has been described in patients after exposure to carboxyhemoglobin. These patients develop neurologic symptoms acutely, appear to recover with treatment, and then exhibit a broad spectrum of neurologic and psychiatric abnormalities days to weeks after the exposure. Studies of DNS, many of which are methodologically flawed, have elucidated neither an exact mechanism nor a consensus on prevention and treatment.
Management and Diagnostic Studies
After removal from the contaminated environment, 100% oxygen should be administered (Table 98.3). If the patient is breathing spontaneously, this can be accomplished with a non-rebreathing face mask. The half-life of carboxyhemoglobin is approximately 4 hours in a patient breathing room air at sea level and approximately 1 hour if pure oxygen is inspired. The half-life is further reduced to less than 30 minutes if the patient has access to hyperbaric oxygen (HBO) at 2 to 3 atmospheres of pressure. There is no widespread agreement on indications for HBO, and transfer to a hyperbaric chamber should not jeopardize cardiopulmonary stabilization. However, HBO administration may have effects beyond the mere reduction in carboxyhemoglobin half-life. Some studies in adults suggest a role for HBO in reducing the incidence of mortality and DNS, but there are no studies in children addressing its effectiveness. Early consultation with a poison control center or an HBO facility should be considered while the patient is receiving 100% oxygen (Table 98.4).
TABLE 98.3
MANAGEMENT OF CARBON MONOXIDE POISONING
Severe metabolic acidosis in the context of normal carboxyhemoglobin and methemoglobin should suggest the possibility of coexistent cyanide poisoning in patients involved in closed-space fires (especially where nitrogen-containing synthetic materials have burned). Cyanide has high mortality but a short half-life (approximately 1 hour), so empiric cyanide levels on patients who have survived the scene are not recommended generally unless confirmation is needed. If cyanide poisoning is strongly suspected in an early presenting patient, the cyanide antidote kit (formerly known as the Lilly kit) may be considered. This two-step kit must be used with caution because the nitrite-containing first step induces methemoglobinemia. In case of doubt, the thiosulfate-containing second step, which is able to scavenge cyanide without significant additional toxicity, may be given alone. Anemia (hemoglobin less than 10 g per 100 mL) must be corrected to maximize oxygen-carrying capacity. Hydroxocobalamin (a synthetic form of vitamin B12) was approved by the FDA in 2006 for use in cyanide poisoning. Its mechanism of action is that the hydroxyl group of the vitamin binds to free cyanide, forming the nontoxic cyanocobalamin. The dose is 70 mg per kg (max 5 g) given over 15 minutes, with a repeat dose if necessary. The minimal side effects of hydroxocobalamin make this an attractive alternative to the traditional nitrite therapy.
TABLE 98.4
CONSIDERATIONS FOR HYPERBARIC OXYGEN THERAPYa
If myoglobinemia or myoglobinuria is present, vigorous hydration and diuresis with furosemide (1 mg per kg intravenously) and/or mannitol (0.25 to 1 g per kg intravenously) with close attention to urine output may preserve renal function. If hydration and diuresis are ineffective, renal failure should be considered and fluids restricted accordingly (see Chapter 108 Renal and Electrolyte Emergencies).
Indications for Discharge or Admission
Determination of disposition from the ED will depend on the history and clinical status. Patients with significant respiratory distress or decreased neurologic status should be admitted to an ICU. Patients who appear well on presentation to the ED but have a significant history (loss of consciousness at the scene) or elevated CO levels should be admitted for monitoring for other sequelae of smoke inhalation.
ENVIRONMENTAL AND EXERTIONAL HEAT ILLNESS
Goals of Treatment
Treatment of heat stroke involves rapid reversal of hyperpyrexia, cardiovascular support, and correction of electrolyte imbalances.
CLINICAL PEARLS AND PITFALLS
• Heat cramps may mimic an acute abdomen.
• Heat stroke is differentiated from heat exhaustion by the presence of circulatory failure and/or severe CNS dysfunction.
• Active cooling may be achieved effectively using evaporation with fans after spraying with cool water.
Current Evidence
Environmental and exertional heat illness occurs with excessive heat generation and storage. These conditions arise when high ambient temperature prevents heat dissipation by radiation or convection, and humidity limits cooling by sweat evaporation. The spectrum of illness is broad, including heat cramps, heat exhaustion, and heat stroke; the latter is an acute medical emergency with significant associated morbidity and mortality.
The elderly are most vulnerable (more than 80% of cases occur in people older than 50 years), but heat illness is also significant among healthy young people. Of the weather-related deaths noted previously for which the age of the decedent was recorded, 164 (4%) occurred in children younger than 15 years. Children with increased risk include those with cystic fibrosis or congenital absence of sweat glands, children receiving medications that cause oligohidrosis, infants left in automobiles on hot days, and young athletes. Heat stroke remains the third most common cause (after head injury and cardiac disorders) of exercise-related mortality among U.S. high school athletes, despite the fact that survival following acute heat stroke has improved over the last century from an estimated 20% to more than 90%.
Heat-sensitive centers of the posterior hypothalamus control sympathetic tone. This tone regulates vasoconstriction of arterioles and subcutaneous arteriovenous anastomoses, which, in turn, controls heat conduction from the body core to the skin. Flow through these areas may represent 0% to 30% of total cardiac output. High flow provides efficient heat transfer from the body core to the skin, which is an effective radiator. Low flow to the skin prevents radiation and allows only inefficient diffusion through the insulating skin and subcutaneous tissues. When body temperature rises, blood in the preoptic area of the anterior hypothalamus is warmer than optimal. Impulses from this area increase and are conducted through autonomic pathways to the spinal cord and then, through cholinergic fibers to the sweat glands, where sweat is released. Exercise and certain emotional states release circulating epinephrine and norepinephrine to increase sweat production.
Clinical Recognition
Three types of heat illnesses are recognized and represent different physiologic disturbances (Table 98.5). Heat cramps refer to the sudden onset of brief, intermittent, and excruciating cramps in muscles after they have been subjected to severe work stress. Cramps tend to occur after the work is done, on relaxing, or on taking a cool shower. Occasionally, abdominal muscle cramps may simulate an acute abdomen. The usual victim is highly conditioned and acclimatized. Typically, these individuals can produce sweat in large quantities and provide themselves with adequate fluid replacement but inadequate salt replacement. Electrolyte depletion is probably the cause of cramps.
TABLE 98.5
CHARACTERISTICS OF HEAT ILLNESS
Most spasms last less than a minute, but some persist for several minutes, during which a rock-hard mass may be palpated in the affected muscle. Cramps often occur in clusters. Rapid voluntary contraction of a muscle, contact with cold air or water, or passive extension of a flexed limb may reproduce a cramp. Laboratory investigation reveals hyponatremia and hypochloremia and virtually absent urine sodium. The blood urea nitrogen (BUN) level is usually normal but may be mildly elevated.
Heat exhaustion is less clearly demarcated from heat stroke than are heat cramps. In most cases, water depletion predominates because individuals who live and work in a hot environment do not always voluntarily replace their total water deficit. Progressive lethargy, intense thirst, and inability to work or play progress to headache, vomiting, CNS dysfunction (including hyperventilation, paresthesias, agitation, incoordination, or actual psychosis), hypotension, and tachycardia. Hemoconcentration, hypernatremia, hyperchloremia, and urinary concentration are typical. Body temperature may rise but rarely to higher than 39°C (102.2°F). If unattended, heat exhaustion may progress to frank heat stroke.
Heat exhaustion may also occur secondary to predominant salt depletion. As in heat cramps, water losses are replaced but without adequate electrolyte supplementation. Symptoms include profound weakness and fatigue, frontal headache, anorexia, nausea, vomiting, diarrhea, and severe muscle cramps. Tachycardia and orthostatic hypotension may be noted.
Hyponatremia, hemoconcentration, and significantly diminished urine sodium are consistent findings. Children with cystic fibrosis, particularly those who are young and unable to meet increased salt requirements, are at risk for electrolyte depletion because salt losses in their sweat apparently do not respond to acclimatization and aldosterone stimulation of the sweat gland.
Heat stroke (Table 98.5) is a life-threatening emergency. Classic signs are hyperpyrexia (41°C [105.8°F] or higher); hot, dry skin that is pink or ashen, depending on the circulatory state; and severe CNS dysfunction. Often sweating ceases before the onset of heat stroke.
The onset of the CNS disturbance may be abrupt, with sudden loss of consciousness. Often, however, premonitory signs and symptoms exist. These include a sense of impending doom, headache, dizziness, weakness, confusion, euphoria, gait disturbance, and combativeness. Posturing, incontinence, seizures, hemiparesis, and pupillary changes may occur. Any level of coma may be noted. Cerebrospinal fluid findings are usually normal. The extent of damage to the CNS is related to the time and extent of hyperpyrexia and to the adequacy of circulation. In severe cases, coma may persist even after the body temperature is lowered.
Patients able to maintain cardiac output adequate to meet the enormously elevated circulatory demand are most likely to survive. Initially, the pulse is rapid and full, with an increased pulse pressure. Total peripheral vascular resistance falls as a result of vasodilation in the skin and muscle beds, and splanchnic flow diminishes. If hyperpyrexia is not corrected, ashen cyanosis and a thin, rapid pulse herald a falling cardiac output. The cause may be either direct thermal damage to the myocardium or significant pulmonary hypertension with secondary right ventricular failure. Even after body temperature is returned to normal, cardiac output remains elevated and peripheral vascular resistance remains low for several hours, resembling the compensatory hyperemia after ischemia noted in posttrauma, postshock, and postseptic states. Persistently circulating vasoactive substances probably account for this phenomenon.
Severe dehydration is not a necessary component of heat stroke but may play a role if prolonged sweating has occurred. Electrolyte abnormalities may occur, especially in the unacclimatized victim, if NaCl has not been replaced. In acclimatized persons, NaCl is conserved but often at the expense of a severe potassium deficit. Polyuria is sometimes noted, often vasopressin resistant and possibly related to hypokalemia. Acute tubular necrosis may be seen in as many as 35% of cases and probably reflects combined thermal, ischemic, and circulating pigment damage. Hypoglycemia may also be noted.
Nontraumatic rhabdomyolysis and acute renal failure have been described as consequences of various insults, including hyperthermia and strenuous exercise in unconditioned persons. Clinically, there may or may not be musculoskeletal pain, tenderness, swelling, or weakness. Laboratory evidence includes elevated serum creatinine phosphokinase (CPK) (300 to 120,000 units) and urinalysis that is orthotolidine (Hematest)-positive without red blood cells and shows red-gold granular casts. Typically, serum potassium and creatinine levels rise rapidly relative to BUN. An initial hypocalcemia, possibly a consequence of deposition into damaged muscle, progresses to hypercalcemia during the diuretic phase a few days to 2 weeks later.
Management and Diagnostic Studies
Heat Cramps
Most cases of heat cramps are mild and do not require specific therapy except for rest and increased oral salt intake. In severe cases with prolonged or frequent cramps, IV infusion of normal saline is effective. Approximately 5 to 10 mL per kg over 15 to 20 minutes should be adequate to relieve cramping. Oral intake of fluids and salted foods can then complete restoration of salt and water balance.
Heat exhaustion
Heat exhaustion as a result of predominant water depletion is treated with rehydration and rest in a cooled or well-ventilated place. If the child is able to eat, he or she should be encouraged to drink cool liquids and be allowed unrestricted dietary sodium. If weakness or impaired consciousness precludes oral correction, IV fluids are given as in any hypernatremic dehydration.
Heat exhaustion caused by predominant salt depletion also requires rest in a cool environment. Alert, reasonably strong children can be given relatively salty drinks, such as tomato juice, and should be encouraged to salt solid foods. Hypotonic fluids (e.g., water, Kool-Aid) should be avoided until salt repletion has begun. Patients with CNS symptoms or gastrointestinal (GI) dysfunction may be rehydrated with IV isotonic saline or Ringer lactate. Initial rapid administration of 20 mL per kg over 20 minutes should improve intravascular volume with return of BP and pulse toward normal. Further correction of salt and water stores should be achieved over 12 to 24 hours. In especially severe cases with intractable seizures or muscle cramps, hypertonic saline solutions may be used. The initial dose of 3% saline solution is 5 mL per kg by IV over 15 minutes for seizures, more slowly over 30 to 60 minutes for cramping. An additional 5 mL per kg should be infused over the next 4 to 6 hours.
Heat Stroke
Treatment centers on two priorities: (i) Immediate elimination of hyperpyrexia and (ii) support of the cardiovascular system (Table 98.6). Clothing should be removed and patients should be cooled actively. They should be transported to an emergency facility in open or air-conditioned vehicles. Ice packs may be placed at the neck, groin, and axilla. Although immersion in ice water may be a more efficient means of lowering body temperature, it may complicate other support and monitoring. Among the most efficient but invasive and rarely used methods is iced peritoneal lavage. Iced peritoneal lavage is contraindicated in the pregnant patient and those with a history of abdominal surgery. In addition, a canine model of heat stroke suggested that an evaporative technique in which fans blew room air over subjects sprayed with 15°C (59°F) tap water was equally efficient. Temperature should be monitored continuously with a rectal probe, and active cooling should be discontinued when rectal temperature falls to approximately 38.5°C (101.3°F). Sedation and paralysis of the patient can greatly augment the cooling process.
TABLE 98.6
MANAGEMENT OF HEAT STROKE
The severity of the patient’s presentation determines the degree of cardiovascular support. If the skin is flushed and BP adequate, lowering body temperature with close attention to heart rate and BP may be sufficient. Although severe dehydration and electrolyte disturbances are uncommon, these should be assessed and corrected if necessary. Fluids cooled to 4°C (39.2°F) hasten temperature correction but may precipitate arrhythmias on contact with an already stressed myocardium. Adult patients rarely have required more than 20 mL per kg over the first 4 hours, but determinations of electrolytes, hematocrit, and urine output, and clinical assessment of central vascular volume should guide precise titration of fluids and electrolytes.
Inotropic support may be required after a fluid challenge (see Chapter 5 Shock). Dobutamine is probably most appropriate: Its β-agonist properties increase myocardial contractility and maintain peripheral vasodilation. Isoproterenol has been used successfully in the past but may cause myocardial oxygen consumption to exceed oxygen delivery. Additional fluid resuscitation may be necessary with the initiation of either dobutamine or isoproterenol to fill the effectively increased vascular space. Normal saline or albumin should be given to maintain the arterial BP in the normal range. Dopamine may also be effective, infused at rates compatible with inotropic support without vasoconstriction (i.e., 5 to 15 mcg/kg/min). In cases of extreme hemodynamic instability, extracorporeal circulation may provide both circulatory support and a means of rapid temperature correction. Agents with α-agonist characteristics (epinephrine and norepinephrine) are not recommended for initial management; they cause peripheral vasoconstriction, interfere with heat dissipation, and may compromise hepatic and renal flow further. Atropine and other anticholinergic drugs that inhibit sweating should be avoided.
Renal function should be monitored carefully, especially in patients who have been hypotensive or in whom vigorous exercise precipitated heat stroke. In general, BUN, creatinine, electrolytes, calcium, and urinalysis for protein and myoglobin should be obtained. Once the patient’s vascular volume has been restored and arterial pressure normalized, hourly urine output should be monitored. If urine output is inadequate (less than 0.5 mL/kg/hr) in the face of normovolemia and adequate cardiac output, furosemide (0.5 to 1 mg per kg by IV) and/or mannitol (0.25 to 1 g per kg by IV) should be given. If the response is poor, acute renal failure should be suspected, and fluids should be restricted accordingly. Rapidly rising BUN or potassium should prompt consideration of early dialysis.
Indications for Admission and Discharge
Patients with heat cramps can generally be discharged for the ED after resolution of symptoms. Children with heat exhaustion may require admission for ongoing fluid and electrolyte replacement and serial testing. Children with heat stroke require admission to the ICU. Patients with ashen skin, tachycardia, and hypotension demonstrate cardiac output insufficient to meet circulatory demand and are in imminent danger of death. Monitoring of the electrocardiogram (ECG) and arterial BP (with an indwelling arterial line) should determine support.
ACCIDENTAL HYPOTHERMIA
Goals of Treatment
The goals of treatment include general supportive measures, cardiopulmonary resuscitation, and rewarming.
CLINICAL PEARLS AND PITFALLS
• A high index of suspicion is needed to recognize hypothermia.
• Hypothermia can mimic death, hence rewarming must be done before pronouncing death.
Current Evidence
Hypothermia was responsible for nearly 14,000 deaths in the United States from 1979 to 1998. Neonates, with large surface:volume ratios and small amounts of subcutaneous fat, conserve heat poorly and are unable to produce heat by shivering. Therefore, minor deviations in the thermal environment may produce hypothermia in neonates. The capacity for nonshivering thermogenesis—primarily metabolism of brown fat—is intact, but oxygen consumption is significantly increased. This may result in metabolic acidosis, hypoglycemia, and hypocalcemia. Adolescents are psychologically less likely to conserve energy and to take preventive or corrective measures, thus increasing their risk of hypothermia. Physical disability, especially immobilizing conditions, and drug or alcohol ingestion increase risk at any age. Healthy young people who work or play to exhaustion in a cold environment are also at risk. The rising popularity of cold weather sports is producing more cases of accidental hypothermia. However, environmental conditions need not be extreme, and the diagnosis should be considered even in temperate climates.
When core temperature begins to fall to less than 37°C (98.6°F), physiologic mechanisms that produce and conserve heat are activated. Cooled blood stimulates the hypothalamus to increase muscle tone and metabolism (oxidative phosphorylation and high-energy phosphate production) and to augment heat production by 50% during nonshivering thermogenesis. When muscle tone reaches a critical level, shivering begins, and heat production increases two to four times basal levels.
Although the surface temperature of the body, especially of the extremities, may drop to nearly the environmental temperature, several mechanisms work to conserve heat and to protect blood and core structures from ambient air temperature, humidity, and wind. Sweating is abolished, decreasing heat loss by evaporation (unless there is external moisture), whereas vasoconstriction of cutaneous and subcutaneous vessels reduce losses further. Piloerection, which in many animal species traps an insulating layer of air next to the skin, occurs but is ineffective in humans.
Once homeostatic mechanisms fail and core temperature falls, predictable physiologic changes take place. If shivering does not occur, basal metabolic rate decreases steadily, reaching 50% of normal at 28°C (82.4°F). As a result, oxygen consumption and carbon dioxide production decline. The oxygen–hemoglobin dissociation curve shifts to the left.
Although respiratory depression occurs late, impaired mental status and cold-induced bronchorrhea predispose the patient to airway obstruction and aspiration. Acid–base balance follows no predictable pattern. Respiratory acidosis occurs, but tissue hypoxia, increased lactic acid production, and decreased lactate clearance by the liver produce metabolic acidosis.
Decreased heart rate contributes primarily to a drop in cardiac output. Peripheral vasoconstriction and an early increase in central vascular volume cause a transient rise in BP, which later falls to become clinically significant at less than 25°C (77°F). A variety of cardiac conduction abnormalities arise, including decreased sinus rate, T-wave inversion, prolongation of ECG intervals, and the appearance of pathognomonic J waves (Fig. 98.5), which may provide the first clue to the diagnosis. Atrial fibrillation may occur at temperatures less than 33°C (91.4°F) but is usually not hemodynamically significant. At less than 28°C (82.4°F), myocardial irritability increases dramatically, and ventricular fibrillation becomes likely.
FIGURE 98.5 J wave (Osborn wave), pathognomonic of hypothermia. Rounded contour distinguishes it from an RSR′. It may also be confused with a T wave with a short Q-T interval. (Reprinted from Welton D, Mattox K, Miller R, et al. Treatment of profound hypothermia. JAMA 1978;240:2291–2292, with permission.)
Cold-induced vasoconstriction and elevated central blood volume and pressure contribute to a diuresis, which subsequently diminishes intravascular volume. At lower temperatures, tubular dysfunction allows salt and water loss. Acidosis causes potassium to shift from cells to the urine, where it is eliminated. Increased capillary permeability results in loss of fluid into the extracellular space.
Hematologic abnormalities may also occur. Plasma loss causes an increased hematocrit level, whereas splenic sequestration may be responsible for a fall in white blood cell and platelet counts. Disseminated intravascular coagulation is sometimes seen.
CNS abnormalities are progressive. Each fall of 1°C produces a 6% to 7% decline in cerebral blood flow. Plasma loss increases blood viscosity, which further contributes to impaired cerebral microcirculation and mentation. Peripheral nerve conduction slows, and deep tendon reflexes decrease. Pupils dilate and react sluggishly, if at all, at less than 30°C (86°F). The electroencephalogram deteriorates progressively with falling temperature, from high-voltage slow waves, to burst suppression patterns, to electrical silence at 20°C (68°F).
GI motility decreases at less than 34°C (93.2°F). The liver’s capacity for detoxification or conjugation of drugs and products of metabolism is poor. Insulin release abates, and serum glucose rises. Frank pancreatic necrosis may also occur, producing clinical evidence of pancreatitis.
Clinical Recognition
Elevated body temperature is a routine concern for most physicians, especially pediatricians. However, hypothermia, defined as core temperature at or less than 35°C (95°F), is often overlooked. Reduced body temperature may be a consequence or cause of many disorders but is diagnosed only if healthcare providers maintain a high index of suspicion. Special thermometers may be required to detect hypothermia.
Triage Considerations
A history of sudden immersion in icy water or prolonged exposure to low environmental temperatures provides the obvious clue, but significantly low core temperatures may occur under much less suggestive circumstances. Examples include trauma victims found unconscious or immobile on a wet, windy, summer day; infants who are from inadequately heated homes or who are left exposed during prolonged medical evaluation; adolescents with anorexia nervosa; and patients with sepsis or burns. Severe hypothermia, coma, and cardiac arrest may present as the sudden infant death syndrome.
Hypothermia may go undetected if the patient’s temperature falls below the lower limit of the thermometer in use or if the thermometer is not shaken down adequately. Low-recording thermometers should be available in EDs and ICUs. This diagnosis should be kept in mind for any patient with a suggestive history or coma of uncertain cause.
Initial Assessment
Physical examination reveals a pale or cyanotic patient. At mild levels of hypothermia, mental status may be normal, but CNS function is progressively impaired with falling temperature until frank coma occurs at approximately 27°C (80.6°F). BP also falls steadily at less than 33°C (91.4°F) and may be undetectable. Heart rate slows gradually unless atrial or ventricular fibrillation occurs. Intense peripheral vasoconstriction and bradycardia may render the pulse unapparent or absent. At less than 32°C (89.6°F), shivering ceases, but muscle rigidity may mimic rigor mortis. Pupils may be dilated and may not react. Deep tendon reflexes are depressed or absent. Evidence of head trauma or other injury, drug ingestion, and frostbite should be sought (Figs. 98.6 and 98.7).
Severe hypothermia mimics death. However, the significant decrease in oxygen consumption may allow life to be sustained for long periods, even after cessation of cardiac function. Signs usually associated with certain death (i.e., dilated pupils or rigor mortis) have little prognostic value. If the patient’s history suggests that hypothermia is the primary event and not a consequence of death, resuscitation should be attempted and death redefined as failure to revive with rewarming.
FIGURE 98.6 Frostbite of toes. Note the line of demarcation and ulcerative lesion.
FIGURE 98.7 Swollen fingers of a child with cold exposure.
Initial laboratory tests should include CBC, platelet count, clotting studies, electrolytes, BUN and creatinine, glucose, serum amylase, and ABGs corrected for temperature (Table 98.7). Urine should be sent for drug screening.
Management and Diagnostic Studies
Therapy for hypothermia can be divided into two parts: general supportive measures and specific rewarming techniques (Table 98.8). Once hypothermia is diagnosed, temperature must be monitored continuously as treatment progresses.
All patients should be given supplemental oxygen. Patients with profuse secretions, respiratory depression, or impaired mental status should be intubated and mechanically ventilated. Intubation should be performed as gently as possible to minimize the risk of arrhythmias.
A decreased metabolic rate produces less carbon dioxide, and usual minute ventilation would produce respiratory alkalosis, increasing the risk of dangerous arrhythmias. Therefore, ventilation should begin at approximately one-half the normal minute ventilation.
Assessment of acid–base status and ventilation in the hypothermic patient is the subject of considerable confusion. Blood gas machines heat the patient’s blood sample to 37°C (98.6°F) before measuring pH and gas partial pressures (thus providing theoretical values if the patient were 37°C [98.6°F]). If the patient’s actual temperature is provided with the sample, the machine can correct the values according to the nomogram of Kelman and Nunn. (Table 98.7 shows one set of guidelines for appropriate correction.) However, it is important to understand two concepts. The first is the ectothermic principle, which relies on the following aspect of physiology: Dissociation of ions and partial pressures of gases are decreased in cooled blood. In hypothermia, therefore, neutral pH is higher, whereas “normal” Pco2 is lower than is encountered at 37°C (98.6°F). For example, hypoventilation of the hypothermic patient with a pH of 7.5 would actually induce an undesirable respiratory acidosis. A second, more practical concept is that if the patient’s blood volume is restored and oxygenation maintained, acidosis will be corrected spontaneously as the patient is warmed.
TABLE 98.7
EFFECT OF BODY TEMPERATURE ON ARTERIAL BLOOD GASES MEASURED AT 37°C (98.6°F)
TABLE 98.8
MANAGEMENT OF HYPOTHERMIA
Heart rate and rhythm should be monitored continuously and the patient handled gently to avoid precipitation of life-threatening arrhythmias in an irritable myocardium. Sinus bradycardia, atrial flutter, and atrial fibrillation are common but rarely of hemodynamic significance. Spontaneous reversion to sinus rhythm is the rule when temperature is corrected. It may be difficult to detect pulses in the hypothermic patient; therefore, it is important to provide chest compressions until pulseless electrical activity has been ruled out by echocardiography or arterial BP monitoring. Ventricular fibrillation may occur spontaneously or with trivial stimulation, especially at temperatures less than 28° to 29°C (82.4° to 84.2°F). Electrical defibrillation is warranted but frequently is ineffective until core temperature rises. Closed chest massage should be initiated and maintained until the temperature is higher than 30°C (86°F), when defibrillation is more likely to be effective. Drug therapy is rarely effective and fraught with hazards associated with decreased hepatic and renal metabolism.
Fluid replacement is essential. Relatively little plasma loss occurs in acute hypothermia but losses may be great in hypothermia of longer duration. Normal saline or lactated Ringer solution, warmed to about 43°C (109.4°F) in a blood-warming coil, is appropriate initially. Electrolyte determinations should guide further replacement. If clotting abnormalities occur, fresh-frozen plasma (10 mL per kg) is a useful choice for volume expansion (see Chapter 101 Hematologic Emergencies). As temperature rises and peripheral vasoconstriction diminishes, hypovolemia is expected. Fluid volume should be sufficient to maintain an adequate arterial BP.
Hypoglycemia, if present, is treated with glucose (0.5 to 1 g per kg by IV). Hyperglycemia, which may result from impaired insulin release in the hypothermic pancreas, should be tolerated to avoid severe hypoglycemia with rewarming.
A number of rewarming strategies exist (Fig. 98.8). Passive rewarming implies removal of the patient from a cold environment and use of blankets to maximize the effect of basal heat production. For patients with mild hypothermia (temperature higher than 32°C [89.6°F]), this may be adequate. As shown in the algorithm, the adequacy of perfusion and the degree of hypothermia are the major factors in the selection of rewarming strategies. For patients with an adequate pulse, passive rewarming is used as the initial strategy if the temperature is greater than 32°C and active core rewarming if the temperature is less than 32°C. Those with poor perfusion require active rewarming with a temperature greater than 32°C and ECMO, if available, with temperature less than 32°C.
Active rewarming is divided into external and core rewarming techniques. Electric blankets, hot-water bottles, overhead warmers, and thermal mattresses are simple, easily available sources of external heat. Immersion in warm-water baths is also possible but complicates monitoring or response to arrhythmias. These methods, however, cause early warming of the skin and extremities with peripheral vasodilation and shunting of cold, acidemic blood to the core. This causes the well-known phenomenon of “afterdrop” of core temperature. Severe hypotension may also occur in chronic cases as vasodilation increases the effective vascular space. External rewarming techniques limited to the head and trunk may minimize vasodilation and afterdrop. In acute hypothermia, active external rewarming is appropriate, but there is some evidence that in chronic cases (more than 24 hours), mortality is higher if active external rewarming is used instead of simple passive techniques.
Core rewarming techniques are almost certainly more rapid and less likely to be associated with afterdrop, dangerous arrhythmias, or significant hypotension. These methods are especially valuable in the setting of severe chronic hypothermia (temperature less than 32°C [89.6°F]), where fluid shifts are most likely to occur. A nonshivering human model of severe hypothermia indicated that inhalation rewarming offered no rewarming advantage, whereas forced air warming (approximately 200 W) allowed a 6- to 10-fold increase in rewarming rate over controls. A canine study of experimental hypothermia found that heated aerosol inhalation alone contributed less heat than endogenous metabolism, but peritoneal lavage and pleural lavage had similar effect on rewarming (6°C/hr/m2). In humans, peritoneal dialysis with dialysate warmed to 43°C (109.4°F) is effective and requires only equipment routinely available in most hospitals. Gastric or colonic irrigation has also been advocated, but placement of the intragastric balloon may precipitate dysrhythmias. Hemodialysis, extracorporeal blood rewarming, and mediastinal irrigation are effective but require mobilization of sophisticated equipment and personnel. Thus, new endovascular warming catheters, introduced after cannulization of the femoral vein and advancement to the inferior vena cava, use closed-loop circuitry to maintain the patient’s temperature. In one case report, a woman’s temperature increased by 2.8°C per hour, with a temperature of 37°C (98.6°F) achieved after 5 hours.
FIGURE 98.8 Algorithm for rewarming. (Adapted from Danzl DF, Pozos RS. Accidental hypothermia. N Engl J Med 1994;331(26):1756–1760.)
Indications for Admission and Discharge
In patients with mild temperature depression (greater than 32°C [89.6°F]), external rewarming techniques and supportive care based on vital signs, ABGs, and metabolic parameters such as glucose and calcium levels, should result in prompt recovery. Patients with mild hypothermia due to environmental exposure who improve with passive rewarming may be discharged after observation in the ED. However, it should be noted that other causes of hypothermia should be ruled out prior to discharge.
Children who present with a temperature less than 32°C (89.6°F), and especially those in whom hypothermia developed over 24 hours or more, require meticulous attention to continuously changing vital signs and metabolic needs. More elaborate core rewarming techniques are appropriate.
FROST BITE INJURY
CLINICAL PEARLS AND PITFALLS
• Care should be taken not to rub or apply pressure to the affected areas.
• Rewarming is painful and analgesics should be provided.
Clinical Recognition
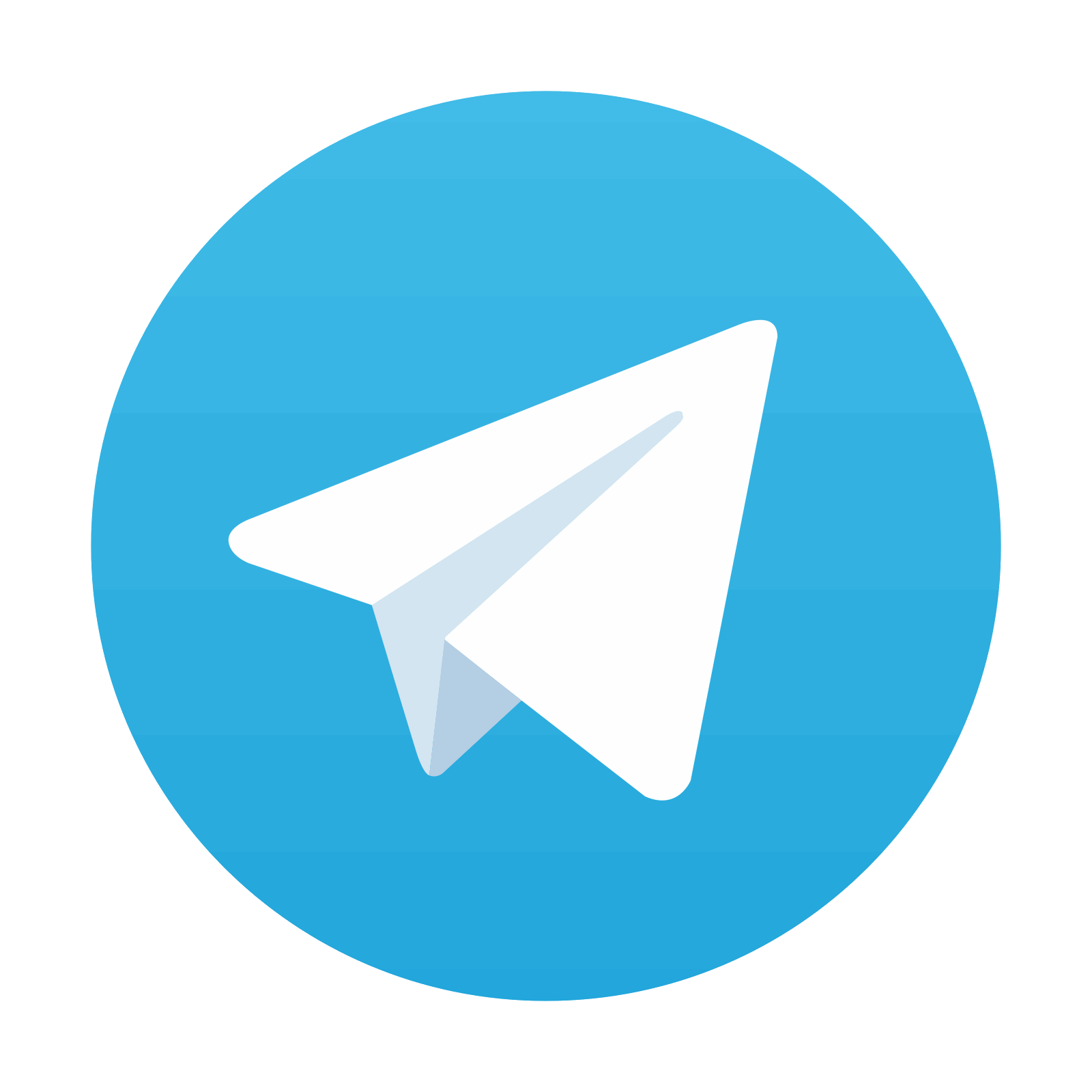
Stay updated, free articles. Join our Telegram channel

Full access? Get Clinical Tree
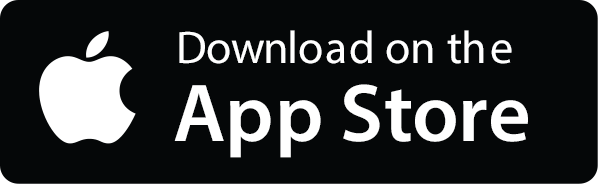
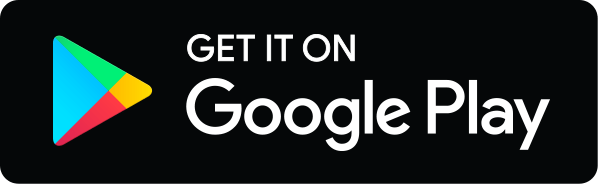