Introduction
Perioperative electrolyte and endocrine disorders are dealt with by anesthesiologists on a daily basis. With the rising incidence of diabetes and obesity and their relation to increased morbidity in the perioperative period, it is imperative that clinicians are skilled in the management of these disorders. This chapter discusses the management of these conditions and other common endocrinological problems such as thyroid disease and the controversy surrounding perioperative steroid supplementation. Finally, this chapter discusses the etiology and management of some of the more commonly encountered electrolyte disturbances.
Endocrine Dysfunction
Diabetes Mellitus
Diabetes mellitus is a metabolic disease characterized by an absolute or relative lack of insulin from the pancreas. There are three distinguished types. Although it can occur at any age, Type I diabetes usually occurs in adolescence. It is believed to result from the autoimmune destruction of insulin-producing cells in the pancreatic islets. There is an absolute deficiency in insulin production and these patients are dependent on exogenous insulin administration for survival. Type II diabetes is caused by insulin resistance, thus resulting in a relative deficiency of insulin. Its onset is typically in adulthood, although recent epidemiologic data suggest that the mean age of onset is decreasing. Type II diabetes results from a heterogeneous group of causes but is usually associated with obesity and abnormal insulin levels. Like many diseases today, there may also be a genetic component.
Gestational diabetes is defined as carbohydrate intolerance beginning in or first recognized during pregnancy. It is believed to occur in 2%–9% of all pregnancies and is associated with substantial rates of maternal and perinatal complications, such as shoulder dystocia, bone fractures, nerve palsies, and hypoglycemia. Long-term adverse health outcomes reported among these infants include sustained glucose intolerance, obesity, and impaired intellectual performance. Gestational diabetes is a strong risk factor for the subsequent development of diabetes in the mother.
Diabetes is marked by long-term complications involving the microvasculature and macrovasculature. This vascular disease is manifested by retinopathy, neuropathy, and nephropathy and constitutes a major risk factor of heart disease, stroke, kidney disease, blindness and nontraumatic amputation. The etiology of these complications is multifactorial and includes glycosylation of proteins and sorbitol synthesis as a result of glucose reduction, which functions as a tissue toxin.
With respect to management of the perioperative patient, diabetes itself is not as important an issue as the degree of end-organ dysfunction that it causes. Several major trials have shown that over the long term, tight glycemic control, tight blood pressure control, and regular physical activity can postpone the microvascular complications in both types of diabetic patients. What is less clear is the benefit of glycemic control in the perioperative period.
In 1878, Claude Bernard described hyperglycemia in response to hemorrhagic shock. It is now well known that injury and stress can cause hyperglycemia as a result of the secretion of glucagon, insulin resistance, and glucose intolerance. This so-called “stress hyperglycemia” is evolutionally preserved and provides the body with a rapid source of energy in times of stress. For a long period of time, stress hyperglycemia was tolerated as it was deemed to be an adaptive evolutionary response to stress. It was not until several studies in both intensive care unit (ICU) and non-ICU patients suggested a link between hyperglycemia and adverse outcome that clinicians began treating hyperglycemia.
There is evidence that perioperative hyperglycemia impairs wound healing. Inadequate insulin action in the early postoperative period leads to impaired hydroxyproline incorporation into the structure of healing wounds. Laboratory studies in diabetic animals have demonstrated that, in insulin-treated animals, repaired tissue could withstand up to three times the mechanical force that separated the incision in non-insulin treated animals. An additional study in a murine diabetic model revealed that blocking insulin activity inhibited DNA and protein synthesis in wounds, thus resulting in decreased fibroblast activation and collagen synthesis and a reduction in capillary proliferation. There is now greater recognition that glycemic control is crucial in maintaining healthy tissue repair and regeneration in the perioperative period.
Diabetic patients are also at higher risk of postoperative wound infections. High glucose levels hinder immune function by increasing neutrophil adhesiveness, decreasing phagocytic activity, and decreasing microbicidal function. Glycemic control is vital in the immediate postoperative period when the patient is particularly vulnerable to infection. Raissas et al. prospectively studied diabetic patients undergoing cardiac surgery. These patients were randomized to an aggressive insulin infusion or standard therapy. Tight glucose control during cardiac surgery was found to improve leukocyte function significantly.
There are several clinical studies supporting short-term hyperglycemia and perioperative infection risk. One investigation involving diabetic patients undergoing major vascular surgery or major abdominal procedures revealed that diabetic patients had higher nosocomial infection rates when their serum glucose values exceeded 220 mg/dL. Zerr and colleagues found an association between infection risk and the mean concentration of blood glucose on the first postoperative day. In that study, the incidence of postoperative wound infection was 1.3% among patients with glucose levels between 100 and 150 mg/dL versus 6.7% among patients with glucose levels between 250 and 300 mg/dL. In a prospective cohort of diabetic patients undergoing coronary artery bypass graft (CABG) surgery, those patients with mean glucose concentrations in excess of 200 mg/dL within the first 36 postoperative hours were more likely to develop infectious complications including infections of their leg and chest wounds. In a similar prospective study involving 2467 diabetic patients undergoing cardiac surgery, a continuous insulin infusion resulted in decreases in perioperative serum glucose levels consistently less than 200 mg/dL, which led to a significant reduction in the incidence of wound infection.
Numerous studies on glucose control have been conducted with patient populations ranging from the critically ill, pregnant diabetics, diabetics undergoing cardiac surgery with cardiopulmonary bypass, and in those with brain injury and global central nervous system (CNS) ischemia. Although some of these studies have demonstrated benefit with tight glucose control, there is little evidence to indicate that tight glycemic control may be of benefit to any other group.
In 2001, Van Den Berghe and colleagues published their seminal study on “Intensive Insulin Therapy in Critically Ill Patients.” This shifted the paradigm from tolerating or normalizing glucose levels to attempting to maintain “tight glucose control” (blood glucose concentration between 80 and 110 mg/dL). Although the mortality reduction in this single center study was impressive, 14 subsequent studies demonstrated no benefit and the suggestion of harm with this tight glucose control as a result of extremely high rates of hypoglycemia.
There is a lack of data on the intraoperative management of hyperglycemia in diabetic patients. Based on the ICU studies on intensive insulin therapy, the safety and efficacy of this therapy has been trialed in operative patients. The majority of these studies have been undertaken on cardiac surgical patients because of the benefit of glucose-insulin-potassium infusions on mortality seen in acute myocardial infarction (although this association has recently been questioned).
A systematic review and meta-analysis of the studies of tight glucose control demonstrated an almost 50% decrease in the odds of mortality when compared with conventional glucose control (< 220 mg/dL). This result was driven almost entirely by one paper. The difficulty with interpreting the evidence regarding the benefit of tight glucose control and cardiac surgery is the limited number of well conducted clinical trials, the significant variability in the target glucose levels, and reporting of outcome measures. Cardiac surgery is plagued with intense periods of surgical stress and therefore wide swings in glucose levels. Considering the intense fluctuation in glucose levels and the question of harm in the ICU studies of tight glucose control, it would seem prudent to avoid tight glucose control in this population and aim for normoglycemia (blood glucose concentration < 180 mg/dL).
In diabetic patients undergoing cardiac surgery with cardiopulmonary bypass, there are sufficient data to recommend maintaining a perioperative blood glucose level below 180 mg/dL. In nondiabetic patients the data are not as compelling and most anesthesiologists are satisfied with a serum glucose level below 200 mg/dL especially in view of a study that revealed that nondiabetic patients experienced hypoglycemia after cardiopulmonary bypass when continuous insulin infusions where used intraoperatively to maintain normoglycemia. Fig. 40.1 presents an algorithm for perioperative glucose management in a both cardiac and noncardiac surgery patients.

Medically Significant Obesity
Obesity is defined by an abnormal accumulation of fat in proportion to body size. Overweight persons, although still technically obese, have a body-fat proportion that is intermediate between normal and obese. The most recently published United States data reveal that approximately 65% of adults are above normal weight: about 30% are overweight (BMI 25 to 30), 30% are obese (BMI 30 to 40), and 5% are extremely obese (BMI ≥ 40). Minority populations have seen a rise in the prevalence of obesity, with the highest rates found in Native Americans, Hispanics, and African Americans, and the lowest rates found in those of Asian ancestry. Prevalence rates for obesity are also highest in populations with less education and lower income levels. Internationally, obesity rates are generally lower than those in the United States, but even societies that traditionally had the lowest prevalence of obesity are starting to observe rates of weight gain that are beginning to meet or exceed those of Western societies. Obesity has now become a major burden on health-care systems and it has surpassed alcohol and tobacco abuse in terms of health-care costs and resource usage.
Men aged 25–34 years with extreme obesity have a 12-fold higher mortality and men aged 35–44 years with extreme obesity have a sixfold higher mortality then non-obese men in the same age group. In addition, obese adults are at increased risk of other comorbidities such as hypertension, diabetes mellitus, coronary artery disease, and a variety of neoplastic diseases.
Preoperative Evaluation
Obesity and its complications present major challenges for the anesthesiologist. For the morbidly obese patient, the main concern for the anesthesiologist is the airway and the cardiopulmonary system. Abundant soft tissue in the upper airway makes the obese patient more susceptible to obstructive sleep apnea and the development of hypoxemia and hypercapnia. Obesity also significantly increases the risk of a difficult tracheal intubation.
Morbid obesity is the most common risk factor for obstructive sleep apnea (OSA). Every increase in BMI of two units increases the likelihood of coexisting OSA by 4%. The prevalence of OSA is 3% to 25% in morbidly obese men and 40% to 78% in morbidly obese women, as opposed to 2% in non-obese women and 4% in non-obese men in the general US population.
It is difficult to predict the presence of OSA based solely on a clinical examination. The definitive diagnostic test for OSA is polysomnography. It is, however, impractical to screen all suspected OSA patients with polysomnography and this has led to the development of clinical predictive tools.
A good example is the STOP-BANG questionnaire developed by Chung et al., which has been shown to have consistently high sensitivity for detecting OSA at different severity levels (84% in mild OSA or above, 93% in moderate or above, and 100% in severe). It consists of questions on Snoring, Tiredness, Observed apnea, and high blood Pressure and is combined with BMI > 35, Age > 50, Neck circumference > 40 cm, and male Gender. Like many screening tools, the high sensitivity of the STOP-BANG score allows users to rule out moderate to severe OSA if the scoring tool ranks them as low risk, i.e., the negative predictive value is good.
A subgroup of obese patients are susceptible to a syndrome called the obesity-hypoventilation syndrome (OHS). This is characterized by chronic daytime hypoventilation and chronic daytime hypoxemia (PO 2 < 65 mmHg) and is easily detected by using room air pulse-oximetry. Sustained hypercapnia (PCO 2 > 45 mmHg) in an obese patient without significant chronic obstructive pulmonary disease (COPD) is also diagnostic for this syndrome. These patients often have BMI > 40 kg/m and the likelihood of OHS increases as the BMI increases. The underlying pathophysiology of this syndrome is unclear and patients on the extreme end of this spectrum who have signs of cor pulmonale are called “Pickwickian.” Pickwickian conditions are associated with increased perioperative morbidity and mortality and these patients may require more extensive preoperative testing and postoperative management.
Because most clinically severe obese patients do not undergo polysomnographic testing, the diagnosis and severity of OSA in a preoperative patient are most commonly gleaned from patient history. For example, reports of constant snoring, interrupted breathing during sleep, morning headache, and daytime somnolence may be indicative of OSA. Systemic hypertension, a BMI > 35, and a neck circumference > 40 to 42 cm at the cricoid cartilage are consistent with the diagnosis of OSA. At present polysomnographic testing is indicated in severely obese patients who continually snore, have daytime somnolence, or have observed periods of interrupted breathing during sleep. Unfortunately, there are no studies to suggest that this approach is correct. In the setting of morbid obesity and OSA without symptoms or signs of underlying pulmonary pathology, tests for room air, blood gases, and pulmonary function have no predictive value in optimizing postoperative outcomes in severely obese patients. Fig. 40.2 presents an algorithm providing guidance on the preoperative evaluation of patients with morbid obesity.

Treatment with Noninvasive Ventilation
Continuous positive airway pressure (CPAP) can reduce or eliminate signs of sleep apnea. To be successful, the use of CPAP requires experienced respiratory care practitioners and patient cooperation. Hence, it is not surprising that use of CPAP results in failure postoperatively because it is often performed in sleepy and uncooperative patients who have not been formally diagnosed with OSA. In addition, it is also provided by practitioners who are not skilled in CPAP titration. It is important to point out that patients require a period of adaptation to the mask and device and not all patients can tolerate this treatment.
The amount of CPAP required varies depending upon whether the sleep disorder involves rapid eye movement (REM) or non-rapid eye movement (NREM) sleep. For example, a CPAP level that eliminates obstructive and hypoxic symptomology during naps may not be effective for nighttime sleep when REM occurs. Unfortunately, compliance with CPAP is in the range of 50% to 80%. Therefore, it is important for the anesthesiologist to make inquiries of each patient on an individual basis regarding the use of their device.
The use of CPAP to reduce complications associated with OSA has recently come into question with the publication of a large randomized controlled trial that did not demonstrate any benefit to CPAP therapy on reducing the rate of death from cardiovascular causes, myocardial infarction, stroke, or hospitalization for unstable angina, heart failure, or transient ischemic attack.
In the preoperative setting it remains unclear whether surgery should be delayed to optimize airway status and oxygenation with CPAP therapy. One study failed to observe any major postoperative respiratory complications in 14 patients treated with nasal CPAP for a period of 3 weeks before surgery. However, in addition to a small sample size this study suffered from the lack of a control group. Therefore, it could not be determined whether these complications would have occurred without the intervention. Other studies have shown that 3 weeks of CPAP therapy before bariatric surgery improved the left ventricular ejection fraction and afterload in patients with severe obesity and heart failure. Eight weeks of preoperative CPAP may be necessary to treat hypertension (HTN) secondary to OSA. In the postoperative setting, one study revealed that CPAP used on the first night after surgery failed to improve oxygenation. The authors believed that this may have been because the REM rebound does not occur until several days after surgery.
Bilateral positive airway pressure (BiPAP) provides positive pressure to the airway during both inspiration and expiration. With this modality spontaneous ventilation is further assisted with increases in airway pressure and flow. BiPAP may have its greatest use in patients who require a significant amount of CPAP or in those who have a severe underlying airway disease such as COPD. The effectiveness of BiPAP in the general sleep apnea population remains unknown.
Cardiovascular Complications of Obesity
Morbid obesity is associated with a higher frequency of cardiovascular disease, including essential hypertension, pulmonary hypertension, left ventricular hypertrophy, congestive heart failure, and ischemic heart disease. Therefore, many patients with morbid obesity will require a thorough cardiac workup. Preoperative patients can be assigned to a risk group based primarily on history and physical examination and the extent of surgery as per the American College of Cardiology/American Heart Association (ACC/AHA) guidelines.
Cardiac output must increase 0.01 L/min to perfuse each kilogram of adipose tissue adequately. Not surprisingly, obese patients often have hypertension, which can lead to cardiomegaly and left ventricular failure. The mechanism for the development of hypertension may be caused by repeated episodes of desaturation and sleep arousals resulting in the activation of the sympathetic nervous system. Although treatment of sleep apnea may result in improvement of HTN, most often treatment cannot be initiated in time for the planned surgery. Treatment of hypertension should be based on current recommended guidelines for patients without sleep apnea.
The association between obesity, sleep apnea, and atherosclerotic cardiovascular disease remains incompletely understood. Episodes of myocardial ischemia are not temporally related to apneic episodes despite sleep studies demonstrating myocardial ischemia in high risk individuals. Myocardial ischemia in this setting could be caused by increased activation of the sympathetic nervous system, resulting in increased myocardial work and increased oxygen demand. Oxygen demand can easily outstrip delivery in this setting because of hemoglobin desaturation. OSA also predisposes patients to congestive heart failure, arrhythmias, and diastolic heart dysfunction.
Chronic daytime hypoxemia can also result in pulmonary hypertension, right ventricular hypertrophy, or right ventricular failure. Anesthesiologists should consider the use of echocardiography to evaluate right heart failure or pulmonary hypertension in selected patients with clinically severe obesity and OSA. However, data are lacking to support the widespread use of this modality at this time.
Deep Vein Thrombosis
Obesity is also an independent risk factor for deep vein thrombosis (DVT) and pulmonary embolus. The estimated incidence of DVT and pulmonary embolism in the postoperative period after bariatric surgery is 2.6% and 0.95% respectively. Venous emboli migrating to the pulmonary circulation is a significant contributor to the 1% to 2% 30-day mortality after bariatric surgery. Most mortality in the 30-day period after bariatric surgery is a result of pulmonary embolism, and this cause of mortality is threefold higher than anastomotic leak and subsequent sepsis. Unfractionated heparin in a dosage of 5000 units administered subcutaneously before surgery and then two times a day until the patient is ambulatory has been shown to reduce the risk of DVT. Enoxaparin in a dosage of 40 mg twice a day has also been shown to decrease the incidence of complications arising from postoperative DVT.
Intraoperative Concerns
In obese patients, the expiratory reserve volume (ERV) and functional residual capacity are reduced to 60% and 80% of normal respectively. ERV is the primary source of oxygen reserve during apnea. Decreased apneic reserve and possibility of difficult mask ventilation has led some to suggest that awake intubation is the safest way to secure the airway in severely obese patients, indicating the potential for difficult intubation. Because of the higher risk of aspiration in the severely obese patient, most practitioners perform a rapid sequence induction to secure the airway, but there is little data to support this practice.
It has been shown that drugs used for analgesia and neuromuscular blockade have increased volume of distribution and prolonged elimination times in severely obese patients. In one study involving eight obese patients (mean weight 94 kg), the elimination half-life of sufentanil was 208 minutes versus 135 minutes for eight control patients (mean weight 70 kg). In a study examining twitch recovery times of vecuronium, the twitch recovery time was 38.4 minutes in obese patients (mean weight 93 kg) versus 17.6 minutes for non-obese patients (mean weight 61 kg).
Postoperative Concerns
It is absolutely essential postoperatively that obese patients receive some form of continuous oxygen supplementation and be maintained in the semi-recumbent or upright position. If oxygen therapy alone is insufficient to maintain adequate arterial oxygenation, it is recommended that CPAP or BiPAP therapy be instituted. The issue of postoperative disposition and monitoring of OSA patients is controversial. Although it is known that patients with OSA are at higher risk of complications, there is no evidence that higher levels of postoperative monitoring reduce these risks, and the latest guidelines from the American Society of Anesthesiology do not have specific recommendations for postoperative monitoring of these patients.
Recommendations
Data from well-designed randomized trials are lacking in the perioperative care of morbidly obese patients. In particular, there are no data to suggest that outcomes are improved if coexisting conditions such as OSA are diagnosed and treated prior to surgery. In addition, there are no data to reveal whether the type of anesthetic administered has an impact on perioperative outcomes. We do recommend polysomnographic testing with CPAP titration in selected patients, and more extensive cardiac testing in selected patients prior to surgery. We also recommend that DVT prophylaxis begin prior to anesthetic induction and early ambulation be encouraged in the postoperative period. In addition to oxygen supplementation, a low threshold for initiating non-invasive ventilation in the postoperative period is recommended for patients who are hypoxemic. Fig. 40.3 presents an algorithm that provides guidance on the perioperative airway and disposition planning for patients with clinically significant obesity.

Corticosteroid Supplementation
Since their introduction in 1949, corticosteroids have been used for a myriad of clinical purposes and have revolutionized the treatment of inflammatory diseases. In fact, it was not long after their initial use that Fraser and colleagues reported the first case of adrenal insufficiency secondary to withdrawal from glucocorticoid therapy. The following sections review (1) the normal physiologic secretion of glucocorticoids and the response to stress; (2) the alterations in the hypothalamic-pituitary-adrenal axis (HPA) with chronic steroid supplementation; (3) the incidence and diagnosis of adrenal insufficiency; (4) current recommendations for identifying and treating patients who need perioperative steroid supplementation; and (5) a review of corticosteroid use in the treatment of sepsis and septic shock.
Normal Physiology
The zona fasciculata of the adrenal gland secretes approximately 5 mg/m of cortisol per day. For the average sized person, this means the total normal cortisol production is about 8.5 mg/day. Glucocorticoid receptors are present on many different tissue types and play a key role in homeostasis. Specifically, glucocorticoids are necessary for lipid, carbohydrate, and protein metabolism. Glucocorticoids also facilitate catecholamine production and the maintenance of normal blood pressure and affect cardiovascular homeostasis. In healthy subjects, glucocorticoid secretion has a diurnal variation with peak levels occurring between 4 am and 8 am . The nadir of their secretion is between 2 am and 4 am . Interestingly, this diurnal variation is lost in critically ill patients.
With stressors such as critical illness, trauma, and surgery, the normal physiologic response is an increase in the level of adrenocorticotrophic hormone (ACTH), which stimulates the adrenal gland to secrete cortisol. However, there is considerable interindividual variation in the HPA response to these stresses. Some of the variation is thought to be the effect of anesthetics, endogenous and exogenous opioids, age, and sleep. Of particular interest is emerging data suggesting that a significant proportion of opioid treated chronic pain patients display a degree of adrenal suppression. Furthermore, the amount of glucocorticoid secreted depends on the amount of surgical stress. Minor surgical procedures result in an adrenal secretion of approximately 50 mg of cortisol per day, whereas major surgical procedures produce between 75 and 150 mg of cortisol per day. To put these levels in perspective, patients with Cushing syndrome secrete an average of 36 mg/day of cortisol. The daily production of cortisol during periods of stress seldom exceeds 200 mg/day in normal subjects. This suggests that this is the maximum dosage that should be administered to patients thought to be at risk of postoperative adrenal insufficiency.
Effect of Chronic Steroid Supplementation on the HPA Axis
Adrenal insufficiency (AI) can be classified as either primary (resulting from the destruction of the adrenal gland by tumor, infection, or hemorrhage), secondary (resulting from pituitary dysfunction), or tertiary (resulting from the therapeutic administration of glucocorticoids). By far, the most common cause of AI is tertiary. Exogenous administration of glucocorticoids results in the inhibition of the hypothalamic and pituitary glands, which results in the atrophy of the adrenal gland over time. Precisely how long it takes for the adrenal gland to recover its function from the exogenous administration of glucocorticoids is the subject of much debate. Studies have shown that the adrenal gland can take as little as 2 to 5 days to recover its normal function or as long as 9 months to a year.
Diagnosis of Adrenal Insufficiency
The diagnosis of tertiary AI in a postoperative patient is difficult and subject to error. Both clinical and physical examination findings need to be supplemented with biochemical testing to confirm the diagnosis. The typical history of fatigue, anorexia, postural hypotension, and weight loss seen in adrenally insufficient patients in the outpatient setting is not relevant in the postoperative state. Surgical patients most often present with an Addisonian crisis that includes fever and hypotension unresponsive to fluid or vasopressor support. The diagnosis is thus confounded by typical postoperative mimics such as hypovolemic shock or sepsis.
Biochemically, the classic findings of adrenal insufficiency include hyponatremia and hyperkalemia. Hyponatremia might be masked by the administration of perioperative fluids. Hyperkalemia is often not present because of intact mineralocorticoid secretion thanks to the renin-angiotensin-aldosterone system. The limitations of the physical examination and baseline biochemical data therefore argue for a more focused interrogation of the HPA axis either with an ACTH stimulation test or with a random cortisol level.
Determining an adequate cortisol response in a postoperative patient suspected of having adrenal disease is made challenging by the previously mentioned interindividual and procedural variability in cortisol levels. Ideally, a threshold cortisol level could be defined, below which the diagnosis of adrenal insufficiency could be made with a high degree of certainty, and therapeutic replacement could be initiated. There are currently no clear consensus values used in the diagnosis of postoperative or critical illness related adrenal insufficiency. A random cortisol value of < 10 μg/dL has appeared in older guidelines as a value below which a diagnosis of adrenal insufficiency can be made. Patients whose cortisol level is above 34 μg/dL are unlikely to have AI and need not be treated.
Further testing of the HPA axis can be accomplished with the ACTH stimulation test. This involves the administration of 250 μg of intravenous cosyntropin (a synthetic ACTH analog) and the measurement of plasma cortisol levels at baseline and at 30 and 60 minutes after administration. Current guidelines suggest that a cortisol value of less than 18 μg/dL (assay specific) recorded at any of the time points are needed to confirm adrenal insufficiency. These guidelines, however, are written for the diagnosis of primary adrenal insufficiency and not AI related to critical illness or perioperative stress.
Unfortunately, anesthesiologists often do not have the luxury of waiting for the results of specialized biochemical tests when faced with a hypotensive patient in the operating room or the recovery room who might have AI. One management option, should this situation arise, would be to administer 8 mg of dexamethasone to the patient, as this will adequately provide the “stress dose” required without interfering with any subsequent testing of the HPA axis. Concurrently, a random cortisol level should be drawn to check for the presence of AI.
Incidence of Adrenal Insufficiency
The reported incidence of tertiary AI in at risk surgical patients ranges from 0.1 to 1 in 1000. Whether this represents an actual low incidence, under-reporting, or poor recognition of this condition is unclear. Many patients undergo major surgical procedures without perioperative glucocorticoid coverage, or even without their baseline therapeutic dosage, without sequelae. The likely explanation for this is that these patients have normal HPA axis function in response to stress.
A collection of small studies has examined the outcomes of omitting additional corticosteroid administration in times of stress amongst patients who would be considered to have corticosteroid induced impaired HPA axis. Bromberg and colleagues prospectively studied 40 renal transplant patients on long-term steroids for immunosuppression who required hospital admission for surgical procedures or acute illness. Despite no stress doses being administered they found no clinically significant cases of adrenal insufficiency.
Glowniak and Loriaux studied patients taking at least 7.5 mg of glucocorticoid per day who underwent major surgical procedures. There were no cases of clinically significant adrenal insufficiency found in either the treatment (stress dosed) or control arm. In 2012 and 2014 Zaghiyan et al. completed two studies involving steroid-treated patients with inflammatory bowel disease requiring bowel surgery. Based on these two studies they concluded that continuing only daily home dose steroids perioperatively was not more likely to yield additional cases of adrenal insufficiency than if patients were administered stress doses. Further, within these studies patients who had been treated with steroids but were not actively taking them did not display AI features despite no perioperative steroid being given.
Despite this, adrenal crisis remains a rare yet feared and potentially fatal complication in the perioperative period and patients taking exogenous steroids are at risk. In light of the rarity of adrenal insufficiency and the small size of studies refuting the need for stress dose steroids, many practicing anesthesiologists retain a conservative approach.
Identifying patients at risk for adrenal insufficiency
The commonly agreed upon scenarios for which perioperative stress dose steroids are indicated include patients who take > 20 mg prednisone equivalent per day for > 3 weeks or have clinical features of Cushing syndrome. Patients who take less than 5 mg/day prednisone equivalent or who have taken any dose for less than 3 weeks are considered to have intact HPA axes and do not require stress dose steroids. Patients who take topical or inhaled steroids are at extremely low risk of developing tertiary AI.
A number of patient groups fall outside of these categories. Such patients form an intermediate group for whom there is concern for HPA axis suppression but limited, if any, evidence to support the practice of administering stress dose steroids. For these patients the risk of harm with excess steroid is a significant concern. Patients who fall into this group include those who: (1) take between 5 and 20 mg prednisone equivalent per day, (2) have received epidural or intra-articular steroid injections in the past 90 days, and (3) were treated but have stopped corticosteroid use within the past year.
Determining which of these patients, if any, need stress dosing is a topic of much debate. No clear guidelines exist. An attractive and much discussed approach is to perform a 250-μg ACTH stimulation test preoperatively although its use has not been shown to predict accurately which patients will develop tertiary AI. It is also time-consuming and costly, making it frequently impractical in the preoperative setting. An alternative approach is to omit stress dosing for these patients and administer a rescue dose of hydrocortisone only if features of AI develop.
Perioperative Steroid Supplementation
Once the decision to administer stress dose steroids has been made, the question then turns to dosage and duration. Although several regimes exist in the literature, all are in agreement that patients should take their usual daily dose throughout the perioperative period and that stress dosing should reflect the magnitude of surgical stress. In 2017 a thorough review of the literature on this topic by Liu included a suggested dosing schedule. This is presented in Table 40.1 .
Type of Surgery | Stress Dose |
---|---|
Minor or moderate | Hydrocortisone 50 mg IV before incision + 25 mg IV q8h × 24 h |
Major | Hydrocortisone 100 mg IV before incision + 50 mg IV q8h × 24 h then taper dose by half per day until usual daily dose reached |
As mentioned previously, identifying patients at risk of tertiary AI by history alone is fraught with difficulties. Nonetheless, focused questioning of patients with inflammatory conditions (such as rheumatoid arthritis or inflammatory bowel disease) for steroid use is simple, and the yield is potentially high. Clinicians should also inquire about any administration of epidural steroids in the previous 90 days, because this has also been shown to suppress the adrenal axis. Patients who take topical or inhaled steroids are at extremely low risk of developing tertiary AI.
The salient questions, however, are what dosage, by what route, and what duration of therapy is sufficient in patients at risk of tertiary AI. Previous recommendations of quadrupling the amount of steroid patients were receiving for stress are: (i) antiquated, (ii) based only on case reports, and (iii) have the potential for increasing morbidity such as wound infections, gastrointestinal ulceration, altered glucose tolerance, and dysphoria. A rational approach to supplementation takes into account the magnitude of the insult as well as the patient’s baseline steroid dose.
For all procedures, patients should receive their daily dose of steroid preoperatively, either orally or parenterally. Patients who are receiving 5 mg of prednisone per day or less do not require steroid supplementation because they continue to have an intact HPA axis. For minor surgical stresses ( Box 40.1 ), based on daily cortisol secretion, a target of 25 mg hydrocortisone equivalent ( Table 40.1 ) is sufficient to overcome the surgical stress. If the postoperative course is uncomplicated, the patient may return to their usual steroid dose on postoperative day 1.
For moderate surgical stress, cortisol production rates suggest that a target of 50 to 75 mg/day of hydrocortisone or its equivalent should be administered for 1 to 2 days. After this period, patients may return to their usual oral regimen if they are tolerating and absorbing oral medications.
For major surgical stresses, 100 to 150 mg of hydrocortisone equivalent per day for 2 to 3 days is required to avoid tertiary AI. This amount can then be discontinued on postoperative day 3 with a return to the baseline regimen.
There are no data to support the contention that patients who are receiving maintenance doses of steroids that exceed the estimated stress requirements need higher perioperative doses. That is, a patient who is receiving a maintenance dose higher than the previously estimated stress requirement will not need extra coverage during the postoperative period. These recommended regimens do not require a long tapering because there is little or no evidence to support the short-term (i.e., less than 48 hours) suppression of the adrenal gland.
Corticosteroids and Sepsis
Although more commonly encountered by intensivists, anesthesiologists are often involved in the care of patients with sepsis and septic shock and much controversy has surrounded the use of corticosteroids in this patient population. High-dose methylprednisolone (30 mg/kg) had previously been used but was subsequently linked to increased mortality and morbidity and ultimately abandoned as a practice about 25 years ago. Since that time, the use of low-dose corticosteroid (< 400 mg/day) has gained traction as a treatment option for sepsis and septic shock, but results of clinical trials have produced conflicting results.
Four randomized controlled trials have been carried out in this patient population with conflicting results. In 2002, Annane found that treatment with low-dose hydrocortisone and fludrocortisone conferred a significant mortality benefit among septic shock patients with demonstrated adrenal insufficiency. In 2008 a study by Sprung found no mortality benefit with low-dose corticosteroid treatment in patients with sepsis and septic shock. A propensity matching study by Funk in 2014 on this topic found a small mortality benefit amongst the most ill patients. In 2018 two large independent trials addressing the issue of corticosteroid use in septic shock were published. Venkatesh found no mortality benefit with the use of low-dose corticosteroid and Annane did find a significant mortality benefit with the use of corticosteroid and fludrocortisone.
Two current guidelines address the issue of corticosteroid treatment in sepsis and septic shock. The Society of Critical Care Medicine 2017 recommend using low-dose hydrocortisone (< 400 mg/day) for at least 3 days only in patients with volume and vasopressor unresponsive septic shock; however, this recommendation is labeled conditional and supported by only low quality evidence. The 2012 Surviving Sepsis Campaign Guidelines similarly suggest using hydrocortisone at a dose of 200 mg/day for volume and vasopressor refractory septic shock, although they also acknowledge that the quality of evidence supporting this recommendation is low.
In conclusion, although evidence remains overall conflicting and weak, there appears to be agreement that treatment with low-dose corticosteroid in severely ill septic shock patients may have mortality benefit and is reasonable. The role of fludrocortisone appears to have some promise but is not yet fully researched.
Of particular interest to anesthesiologists, evidence sources agree that the use of etomidate should continue to be avoided in patients with septic shock.
Thyroid Disease and Anesthesia
Anesthesiologists are frequently faced with patients who have preexisting hyperthyroidism or hypothyroidism. The management of these patients during elective surgery is straightforward and does not necessitate any change in anesthetic technique. Problems arise, however, when patients who are floridly hyperthyroid or hypothyroid present for surgery. Patients who are not in a euthyroid state should only be operated on for life-threatening illnesses. The postoperative complications that arise in patients who are not clinically euthyroid make elective surgery a dangerous adventure. This section will address some of the concerns of anesthetizing patients with untreated or uncontrolled thyroid disease. Unfortunately, evidence-based guidelines are not available because of the low incidence of patients with acute thyroid dysfunction presenting for surgery and the lack of controlled trials on management strategies.
Hypothyroidism
Hypothyroidism is a relatively common condition that occurs in 1% of all patients and in approximately 5% off those over the age of 50 years. Females represent the vast majority of patients. The most common (noniatrogenic) cause of hypothyroidism is Hashimoto thyroiditis, which is an autoimmune disorder caused by antibodies to thyroid tissue. Patients with this condition should be screened for other autoimmune diseases because they are often present. The diagnosis and clinical management of these patients has been reviewed recently.
Some of the manifestations of overt hypothyroidism of concern to the anesthesiologist are a decrease in the ventilatory response to hypercarbia and hypoxia, impaired gastric emptying, decrease in free water clearance, altered ability to thermoregulate, and decreased cardiac output and bradycardia. These patients have a slightly decreased anesthetic requirement and opiates, induction agents, and volatile anesthetic agents should therefore be titrated accordingly.
Myxedema coma is the most extreme form of hypothyroidism and anesthesiologists may encounter these patients in the perioperative setting. Common precipitants of myxedema coma include trauma, burns, stroke, sepsis, amiodarone, and the postoperative state. The mortality from myxedema coma is high (greater than 60%), but fortunately this condition is exceedingly rare.
Clinical manifestations of this condition include a decreased level of consciousness, coma, decreased cardiac output, hyponatremia, hypoventilation, and hypothermia. Myxedema coma is an endocrinological emergency and attention should be focused on securing the patient’s airway, control of ventilation, and circulatory support, and these patients should be transferred to an intensive care unit. Despite an increase in total body water, these patients are hypovolemic and subject to profound hypotension should they become vasodilated. Thus external rewarming is ill-advised because the resultant vasodilation that occurs with rewarming could result in cardiovascular collapse. Normothermia will be achieved with normalization of thyroid hormone levels. Hypotension should be treated with the administration of warmed intravenous fluids. Vasoactive agents should be used judiciously because they may precipitate arrhythmia. Hypoglycemia, which is often present, responds well to glucose infusions. With the lack of a febrile response or leukocytosis, broad spectrum antibiotics should be initiated in patients without an obvious precipitant for myxedema coma after appropriate cultures have been obtained.
Definitive management of myxedema coma relies on the administration of intravenous thyroid hormone. The proper initiation of thyroid hormone replacement is controversial with regard to the selection and dose of thyroid hormone (either T4 or T3). In theory, T3 is the logical choice, because it is the form of thyroid hormone active at the cellular level. Further, T4 is converted to the active T3 by a deiodinase enzyme, levels of which are depressed in myxedema coma. This has led several investigators to suggest T3 as the logical replacement choice. T3, however, is expensive, difficult to obtain, and may precipitate arrhythmia. There are also no controlled trials demonstrating improved outcome with T3. Thus most investigators recommend the use of intravenous T4 as the sole replacement hormone. A loading dose of 200–500 μg IV followed by 50–100 μg IV per day afterward is suggested. Concomitant glucocorticoid therapy (hydrocortisone 50 mg IV every 6 hours) should also be initiated, as the presence of AI in these patients is high. Cortisol levels should be drawn prior to initiation of therapy and if random levels greater than 25 μg/dL are found, glucocorticoid therapy can be discontinued.
Hyperthyroidism
Graves’ disease or thyrotoxicosis is the most common cause of hyperthyroidism and is caused by the presence of autoimmune antibodies directed against the thyroid hormone receptor. These autoantibodies cause stimulation of the TSH receptor and result in excessive production of thyroid hormone. Clinically, overt hyperthyroidism is manifest by tachycardia, tremor, diarrhea, ophthalmopathy, and goiter. The diarrhea might be severe enough to cause hypovolemia, acid base abnormalities, and electrolyte disturbances. Anesthesiologists should pay particular attention to the size of the goiter as it may be large enough to cause airway obstruction upon induction of anesthesia. Historical features, such as shortness of breath in a recumbent position, and supplemental tests, such as postural flow volume loops and computerized tomography scans, should be evaluated prior to anesthesia. An awake intubation with an armored tube placed distal to the obstruction should be performed with a large goiter. Extubation should occur in the operating room or the ICU with optimal circumstances (complete reversal of neuromuscular blockade and optimal level of consciousness). Postoperatively after thyroidectomy for a large goiter, the possibility of tracheomalacia and tracheal collapse secondary to weakened tracheal rings warrants close observation.
The progression of thyrotoxicosis to thyroid storm is also a rare event. Most often, thyroid storm is caused by a thyroid event such as thyroid surgery, iodinated radiocontrast dye administration (Jod-Basedow effect), and withdrawal of antithyroid drugs. Other common precipitants include surgery, trauma, stroke, parturition, and severe infections. Physical manifestations of thyroid storm result from sympathetic stimulation and include hyperthermia, altered mental status, tachycardia, high cardiac output (that might develop into heart failure), hypertension with a widened pulse pressure, and arrhythmia.
The treatment of thyroid storm requires admission to an intensive care unit and involves general support of the patient, blocking the peripheral effects of thyroid hormone, blocking synthesis of thyroid hormone, and treatment of precipitating causes. Initial management involves inhibition of iodine organification with the thioureas, propothiouracil (PTU), or methimazole. PTU has the added advantage of inhibiting the peripheral conversion of T4 to T3. PTU is administered in a 600–1000 mg loading dose, followed by 1200 mg/day in four divided doses. If the oral route is unavailable, PTU is also absorbed rectally. The next step is to inhibit the secretion of preformed thyroid hormone with iodine. PTU and methimazole only inhibit the formation of new thyroid hormone and have no effect on the release of preformed hormone. Several hours should elapse between the administration of the thioureas and iodine. If iodine is given before organification blockade, it may precipitate a substantial release of thyroid hormone (Jod-Basedow effect). Stress dose steroids (hydrocortisone 100 mg IV three times daily) can also block the release of thyroid hormone.
Simultaneous with the inhibition of thyroid hormone release and synthesis, the peripheral effects of thyroid hormone should be antagonized with the use of beta blockers. Propranolol has been the most commonly used beta blocker for this, but other more selective beta blockers such as metoprolol and esmolol can also be used. Beta blockers have the additional effect of blocking the peripheral conversion of T4 to its active form T3.
Thyroid Hormone and Cardiac Surgery
The effects of thyroid hormone on the heart have long been known. Furthermore, it is also well known that a low T3 state occurs after cardiopulmonary bypass resulting in a low cardiac output and high systemic vascular resistance state. Studies that have shown thyroid hormone to be a positive inotrope and a vasodilator have led to clinical studies that assess its role in the treatment of this post-bypass abnormality.
In adults undergoing coronary artery bypass grafting (CABG), T3 levels decrease between 50% and 75% from baseline and remain depressed for the first 1–4 postoperative days. The precise reason why this occurs is not well known. Proposed mechanisms include hypothermia and increased IL-6 levels, both of which may cause decreased conversion of T4 to T3, or a decreased half-life of T3.
Initial clinical studies suggested that the administration of T3 to patients undergoing CABG improved hemodynamics and overall outcome. These initial studies were, however, small in nature and based mostly on anecdotal experience. These initial studies led to larger randomized placebo-controlled trials. Most of them showed a decrease in the need for inotropic support, but overall outcome was the same between groups. Interestingly, in some of the studies a lower incidence of atrial fibrillation was found. This is unusual because this is one of the more common dysrhythmias in patients with hyperthyroidism. Of note, none of the studies performed to date show an increase risk of adverse events in the treatment group. Despite the lack of clear outcome benefit, a potential role for thyroid hormone in CABG patients does exist and warrants further study.
Alteration in the HPA axis resulting in hypothyroidism is also commonly present in organ donors who have suffered brain death. Studies where a protocol for hemodynamic support included replacement with thyroid hormone have shown a decreased need for vasopressors and a substantial increase in the number of organs transplanted. Thus, thyroid hormone replacement should be considered in any hemodynamically unstable donor patient who is receiving vasopressor therapy.
Sodium
Hyponatremia
Hyponatremia is defined as a serum sodium concentration less than 135 mEq/L and it can occur in a hypotonic, hypertonic, or isotonic state. Hypertonic hyponatremia (increased plasma osmolality) is caused primarily by solutions such as glucose or by mannitol, as seen in transurethral resection of the prostate (TURP).
The most common type of hyponatremia seen in the perioperative setting or in the critically ill is hypotonic hyponatremia, which can occur in the isovolemic, hypervolemic, or hypovolemic state. All three involve an impairment in the excretion of renal water along with continued intake of dilute fluid.
Isovolemic hyponatremia occurs with retention of water in the absence of sodium, and there are no clinical signs of edema. The most common postoperative cause of this condition is syndrome of inappropriate antidiuretic hormone (SIADH), which can be caused by pulmonary or cranial neoplasms or infections, postoperative pain (secondary to sympathetic activation), and drugs such as tricyclic antidepressants or opiates.
Hypovolemic hyponatremia is commonly caused by gastrointestinal losses, third-space losses, or adrenal insufficiency.
Hypervolemic hyponatremia is characterized by edematous states such as congestive heart failure, cirrhosis, and renal failure. This state is characterized by sodium retention with disproportionately larger amounts of water.
Management of Hyponatremia
When the serum sodium level remains greater than 125 mEq/L, patients are usually asymptomatic. Lower values usually result in symptoms, especially if the hyponatremia has developed quickly. Nausea, vomiting, visual disturbances, depressed level of consciousness, altered mental status, seizures, disordered reflexes, loss of thermoregulatory control, muscle cramps, and weakness can be seen. Cerebral edema begins to manifest at a sodium concentration of 123 mEq/L and, if it progresses rapidly, it may lead to transtentorial herniation. Cardiac symptoms occur at levels of less than 100 mEq/L and can result in pulmonary edema, hypertension, and heart failure.
It is recommended that the rate of correction of asymptomatic hyponatremia be 0.6 to 1 mmol/L/h until the sodium concentration is 125 mEq/L, using hypertonic saline. Then, one half of the deficit should be administered over 8 hours and the remaining half over 1 to 3 days. Concurrent treatment with furosemide is also recommended to avoid volume overload. A further advantage to this combination is that furosemide treatment is equivalent to the administration of one-half normal saline and thus aids in the correction. Patients who have symptomatic hyponatremia should have their serum sodium concentration raised by 3 to 7 mmol/L. Overly rapid correction of hyponatremia places patients at risk of osmotic demyelination. This usually occurs when serum sodium is increased by more than 12 mmol in a 24-hour period.
The sodium dosage for deficit correction can be based on the following formula:
Dosage=bodyweight×140−Na×0.6
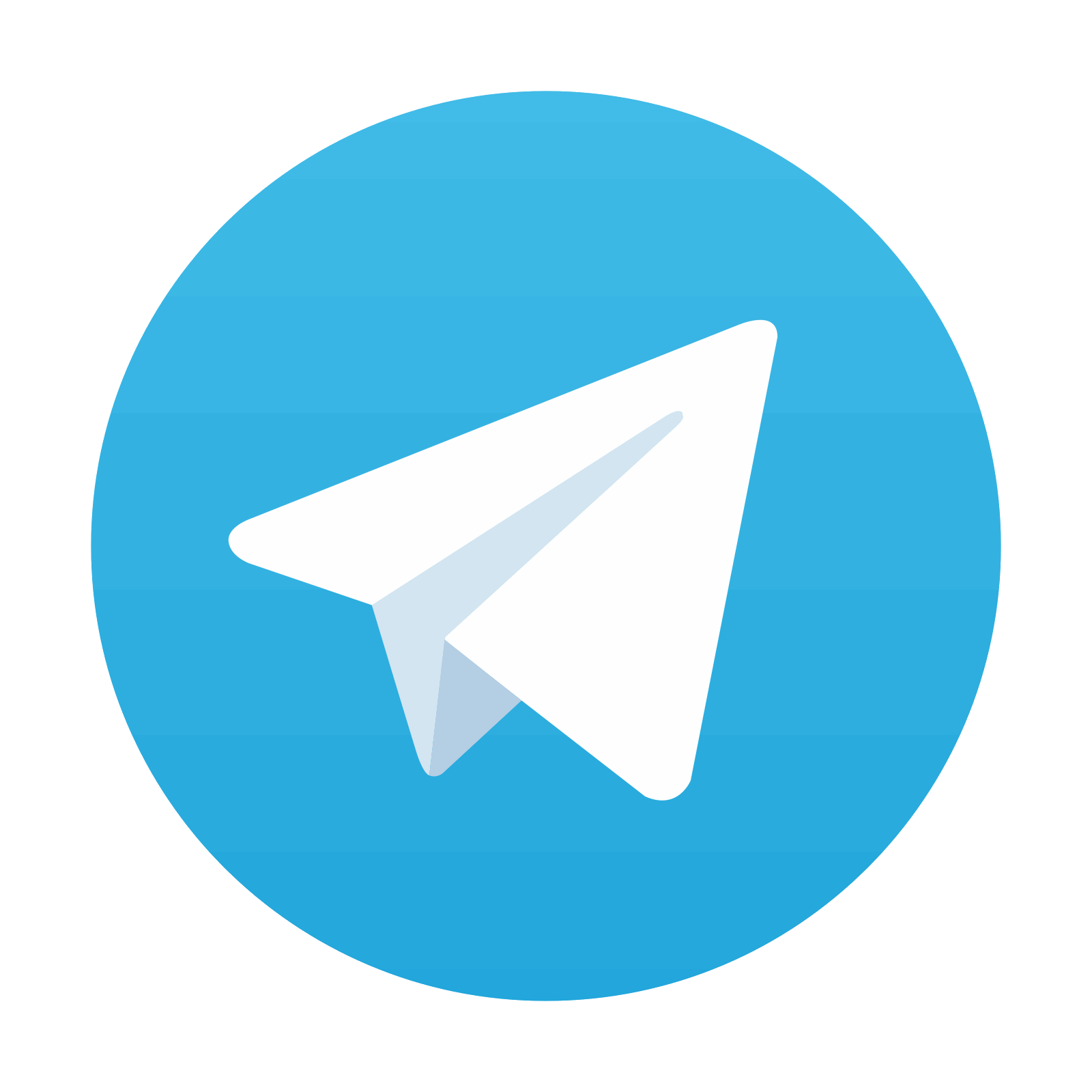
Stay updated, free articles. Join our Telegram channel

Full access? Get Clinical Tree
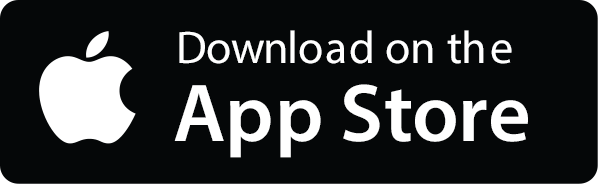
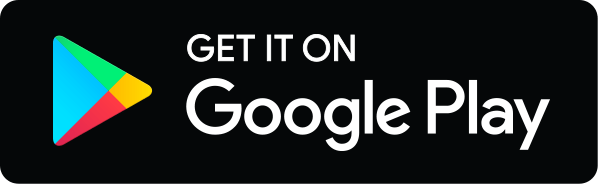
