Fig. 7.1
Placement of electrodes for recording EMG responses from the extraocular muscles (CNs III, IV, VI), facial muscles (CN VII), masseter muscle (CN V), trapezius muscle (CN XI), and the tongue (CN XII). Cranial nerve IX is monitored by either placing needles or an adhesive surface electrode in or on the soft palate. Cranial nerve X can be monitored by placing needle electrodes in the vocal folds, percutaneous needle electrodes in the larynx muscles, or by using a tracheal tube with built-in surface electrodes. Also included are electrodes for recording auditory brainstem responses (ABRs) or brainstem auditory evoked potentials (BAEPs) and visual evoked potentials (VEPs). Also shown are insert earphones for presenting a click stimulus to the inner ear and eliciting the ABRs and a contact lens with light-emitting diodes for stimulating the eye and eliciting the VEPs. As a result, the function of cranial nerves VIII and II can be assessed as well. Reprinted from Moller et al. [28]
Table 7.1
Muscle groups commonly used to assess cranial nerve function based on their innervations
Cranial nerve | Muscle group |
---|---|
III | Medial rectus |
IV | Superior oblique |
V | Masseter, temporalis |
VI | Lateral rectus |
VII | Orbicularis oris, orbicularis oculi |
IX | Soft palate |
X | Vocal cords |
XI | Trapezius |
XII | Tongue |
As below, continuous monitoring of spontaneous or free-running EMG activity provides a means for assessing if an injury may occur to a cranial nerve. Normally, the activity should remain flat or quiet, indicating that the nerve has not become activated as a result of mechanical stimulation. Manipulation of a nerve may result in periods of activation. The length of time a nerve is activated depends on the degree of nerve irritation [4, 29]. Short periods of activation generally correlate with no permanent injury. Frequent or sustained periods of activation have a greater likelihood of being associated with a postoperative neurologic deficit [30]. This type of sustained activation has been given the name “A-train” activity , the occurrence of which has been associated with postoperative paresis in patients operated on for vestibular schwannoma [11, 13, 31] and microvascular decompression for trigeminal neuralgia [12, 27, 28]. The condition of a nerve prior to manipulation also plays a role in determining how it will react to mechanical stimulation. Manipulation of normal healthy nerves will generally produce little or no activation, whereas nerves that are already slightly injured will tend to react more strongly when manipulated and may act as impulse generators of spontaneous EMG activity even when no manipulation is taking place [4, 30]. However, severely injured nerves may not react to mechanical stimulation at all and therefore the absence of EMG activity does not guarantee that a nerve has not sustained an injury. As a result, it is important to use electrical stimulation to validate that an injury has not occurred. If the responses that result have latencies or amplitudes that are prolonged or diminished relative to baseline responses, these are indications that an injury has occurred.
Monitoring Specific Cranial Nerve Function
Cranial Nerves V and VII
Historically, monitoring cranial nerve function began with attempts to assess and preserve the motor function of the facial (VIIth cranial nerve) and trigeminal (Vth cranial nerve) nerves during operations for removal of large acoustic or other skull base tumors [1, 2]. The facial nerve provides innervation to the orbicularis oculi and orbicularis oris muscles, whereas the trigeminal nerve innervates the muscles of mastication (masseter and temporalis muscles). Therefore, if pairs of needle electrodes are placed in these muscles, they provide a means for recording EMG activity during tumor removal and other surgical procedures [12–15, 19, 20, 32]. If each pair is connected to a different recording channel, the recordings provide a means for differentiating between which muscle group is being activated and hence which cranial nerve is being irritated or stimulated. Electrical stimulation of the trigeminal nerve will result in responses with a peak latency less than 6 ms, whereas the responses elicited by facial nerve stimulation will have peak latencies greater than 8 ms [30]. This provides another means for differentiating which cranial nerve is being activated.
During operations for removal of large acoustic tumors or during operations in the cerebellopontine angle, the function of the component branches (acoustic and vestibular) of the VIIIth cranial or auditory nerve is clearly at risk. Unlike the other cranial nerves where the monitoring is dependent upon recording EMG activity, the auditory nerve consists of sensory pathways and, as a result, monitoring of auditory nerve function is dependent on assessing sensory pathway function using brainstem ABRs. This monitoring technique is thoroughly discussed in Chap. 3 (“Auditory Evoked Potentials”).
Cranial Nerves III, IV, and VI
Cranial nerves III, IV, and VI innervate the extraocular muscles. Hence, as is the case when monitoring facial and trigeminal nerve function, if needle electrodes are placed in or in close proximity to these muscles, cranial nerve function can be monitored by recording the EMG activity from the muscles that these nerves innervate [27, 28]. Cranial nerve III function can be monitored by recording from the medial rectus muscle. Similarly, cranial nerve IV function is monitored by recording from the superior oblique muscle. Recordings from the lateral rectus muscle are used to monitor cranial nerve VI function [21]. Because of space limitations, only single electrodes are placed in each of these muscles with a reference electrode placed contralateral to the side of surgery.
Cranial Nerves IX, X, XI, and XII
As was the case with monitoring cranial nerve III, IV, and VI function using the EMG activity from extraocular muscles, the function of the IX, X, XI, and XII cranial nerves is assessed by monitoring the EMG activity from muscles innervated by the motor components of these cranial nerves [15–20, 33]. Although these cranial nerves also contain sensory and autonomic components, it is assumed that the EMG activity associated with these nerves represents the condition of the entire nerve and not just the motor component. The motor component of the IXth cranial nerve is monitored by recording the EMG activity from the soft palate using needle electrodes [9, 27]. Although it is possible to monitor the motor function of the Xth cranial nerve (the vagus nerve) using needle electrodes placed in the vocal folds, it is difficult to place these electrodes [9]. As a result, recording of vagal nerve EMG activity is typically done using a tracheal tube with attached electrodes that are able to make contact with the vocal cords. Monitoring of recurrent laryngeal nerve (a branch of the vagus nerve) function during surgery is a common application of this technique [16, 17]. Monitoring the motor function of the spinal accessory or XIth cranial nerve is relatively easy. This is done by placing needle electrodes in the trapezius muscle and recording the ongoing EMG activity. Finally, monitoring of the XIIth cranial or hypoglossal nerve is done by placing needle electrodes in the tongue [9, 28], although other techniques have been shown to be effective as well [34]. The hypoglossal nerve is very small but very important. Therefore, if there is any question regarding its location or identity, electrical stimulation can elicit EMG responses from the tongue or other appropriate muscles. When monitoring the function of all of these cranial nerves, caution must be taken with regard to the stimulation intensity that is used for eliciting EMG responses from the muscles that they innervate. If the intensity is too high, the resulting responses may be excessive and possibly injurious.
Although the amplitude of the EMG responses resulting from electrical stimulation of the various cranial nerves may vary, the responses are typically monitored by viewing their traces on an oscilloscope-type display with each trace dedicated to a particular cranial nerve. In addition, the surgeon may want to receive auditory feedback of the stimulus and any elicited EMG activity each time they stimulate. Therefore, most monitoring equipment comes equipped with a speaker system for this purpose.
However, the use of electrical stimulation to test cranial nerve function is necessarily only intermittent and, in addition, sometimes a nerve will be inaccessible to test because of the size of a tumor. Hence, even when a nerve is available for testing, this method cannot provide the desired ongoing functional assessment. In such cases, the use of multipulse transcranial electrical stimulation provides a means for continuous monitoring of cortibulbar pathway function. The technique involves the use of the same standard scalp stimulation sites and parameters that are routinely utilized to elicit transcranial motor-evoked potentials (tcMEPs) (see Chap. 2, “Transcranial Motor-Evoked Potentials”). However, rather than recording these MEP responses from muscles in the extremities, the recordings are acquired from the same muscles in the face and head that are routinely used to monitor triggered and spontaneous EMG activity when assessing cranial nerve function in the traditional manner as discussed earlier. These responses are known as corticobulbar tract motor-evoked potentials (CBT-MEPs) . The successful use of this corticobulbar technique during cerebellopontine angle (CPA) tumor removal when facial nerve function is at risk or for other skull base surgeries when the function of other cranial nerves is at risk, including the vagal nerve pathways, has been reported [35–38].
Brainstem Mapping
Brainstem mapping is a neurophysiologic technique for locating the cranial nerve motor nuclei (CMN) on the floor of the fourth ventricle. This technique has proven to be valuable for preventing damage to the CMN during surgery for the removal of tumors and other pathologies located in and around the brainstem [20, 39–43]. Occasionally, the surgeon is confronted with having to make a decision regarding the safest approach to reach structures below the brainstem surface. When this approach involves the floor of the fourth ventricle, stimulation of the floor at various locations using a handheld stimulation probe can result in EMG responses from various muscle groups that are innervated by cranial motor nerves of the head. These muscle responses can provide information regarding the location of various CMN and also safe entry points to the brainstem. The motor nuclei are generally located near specific anatomic landmarks such as the facial colliculus and striae medullares. However, even in normal patients, visualization of these landmarks is not always apparent; when these landmarks become distorted due to the presence of a tumor, the locations of these nuclei become even more problematic. This is when this technique is of particular value. However, because it is only used intermittently during tumor resection to localize and confirm the location of CMN, it is only a mapping and not a monitoring technique. Unlike monitoring techniques, it is not used continuously to assess function and validate the integrity of neural pathways. Therefore, any neural damage that might occur during brainstem tumor resection would not be preventable using this technique.
Mapping Technique
Stimulation of the floor of the fourth ventricle is performed using a handheld monopolar stimulation probe with a tip that allows for very focal stimulation. The anode or return electrode is generally placed at Fz, near the front of the scalp. Stimulation consists of 0.2-ms pulses presented at a frequency of 4 Hz. The stimulation intensity is kept low and generally begins at 1.5–2.0 mA. Once muscle responses are obtained, the intensity is gradually reduced in order to establish stimulation thresholds. This is generally between 0.3 and 2.0 mA [43].
As is the case with cranial nerve monitoring, recording electrodes are inserted into the appropriate muscle groups. These are placed in the extraocular muscles for mapping the cranial nerve III, IV, and VI motor nuclei. For the CMN VII, they are placed into the orbicularis oris and oculi muscles. For mapping the cranial nerve IX and X motor nuclei, the electrodes are inserted into the soft palate and posterior pharyngeal wall using direct laryngoscopy. Alternatively, a tracheal tube with attached electrodes can be used. For the CMN XI, electrodes are placed in the trapezius muscle, and for the CMN XII, electrodes are inserted into the lateral aspect of the tongue. The EMG responses that result from stimulation of these nuclei are typically several hundred microvolts in amplitude and can be recorded using a time base of 20 ms and a filter bandwidth between 50 and 2000 Hz.
Anesthetic Management
Because the EMG responses used for mapping purposes result from the activation of lower motor neurons, the anesthetics used for general anesthesia have little or no effect on these responses. Because lower motor neurons are being stimulated via the motor nuclei of cranial nerves or their intramedullary roots, as long as muscle relaxants are not utilized during the mapping and monitoring period, any type of anesthetic management is compatible with brain stem mapping.
Motor Strip Mapping
Identification of the motor cortex is typically performed by placing a recording grid of electrodes directly on the cortical surface of the pre- and post-central gyri. The contralateral median or ulnar nerves are then stimulated and the resulting somatosensory-evoked potentials (SSEPs) can be recorded from each of the grid electrodes. At the transition between the sensory and motor cortex, the elicited responses reverse polarity or undergo a phase reversal. In this way, in most instances, the location of the motor cortex can easily be identified. However, with distortion of the cortical anatomy that can occur as a result of the presence of a brain tumor, these results can become unreliable. Phase reversal may not be obtained and other techniques may have to be used. One of these techniques utilizes direct electrical stimulation of the cortical surface to elicit motor responses that can be visualized or to elicit EMG responses that can be recorded from various muscle groups of the limbs, face, or trunk [44]. Location of the lesion within or adjacent to the motor cortex will dictate what muscle groups to focus on. Subdermal needle electrodes are then placed within the appropriate muscles.
The surgeon uses a handheld stimulator to excite the cortex. The electrical stimulation technique relies on the activation of the cortical circuitry by means of a train of bipolar, biphasic short-duration (1 ms) rectangular electrical pulses. These pulses are applied at a rate of 50–60 Hz. The intensity of the stimulus is normally kept low (3–5 mA) and is gradually increased in 1- to 2-mA steps until motor responses are elicited. The duration of the stimulus train is typically applied for a few seconds in order to detect any elicited motor responses in the spontaneous EMG activity that is being observed. The surgeon notes the location of these occurrences and in this way is able to determine what tissue can be removed while still preserving function. Chapter 9 (“Cortical Mapping”) provides a more comprehensive discussion of this topic.
Techniques for Assessing Nerve Root Function and Pedicle Screw Placement
The use of pedicle screws for spinal stabilization is becoming increasingly more common. However, proper placement of pedicle screws such that they do not irritate or injure nerve roots requires that the surgeon doing the screw placement be very experienced and knowledgeable about the anatomic characteristics of all aspects of the spine. Although a surgeon will rely on anatomic landmarks and fluoroscopy for accurate placement, the placement is still largely done blindly. Ideally, screw placement should result in the screws being placed within the pedicles with about 1 mm of bone between the lateral and medial walls of the pedicle and with no breaches of the pedicle walls. However, when significant deformity is present, even a skilled surgeon can misplace screws. Nerve roots tend to position themselves near the medial and inferior aspects of the pedicles as they exit the spinal canal through the spinal foramen. If screws are misplaced such that they protrude from the pedicle wall in either of these areas, they can cause nerve root irritation or injury. The current literature indicates that the incidence of screw placements that result in cortical perforations of the pedicle wall ranges between 5.4 and 40 % [45]. Such events might go undetected because most surgeons are reluctant to visualize and validate screw placements unless such actions are warranted. To do so would require multiple laminotomies, which is time-consuming; in addition, these actions by themselves could affect postoperative outcomes. As a result, fluoroscopy has largely been relied on to detect misplaced screws. The incidence of screws associated with neurologic functional impairment has been reported to range from 1 % to more than 11 % [46–49]. Improved imaging technology, including intraoperative computed axial tomography (CAT) scans and stereotactic imaging technology may help to reduce the incidence of misplaced screws. However, in the meantime, electrophysiologic techniques have evolved for monitoring and assessing screw placements that rely on the use of both spontaneous and triggered EMG activity.
Methodology
As indicated earlier, the purpose of monitoring during surgical procedures involving pedicle screw placements is to protect and preserve nerve root function. Because all nerve roots consist of both sensory and motor fibers, the monitoring techniques that can be used to assess nerve root function can involve the acquisition of either sensory or motor responses. The sensory responses that are mediated by a single nerve root are known as dermatomal evoked responses and can be elicited by electrically stimulating a specific body surface area known as a dermatome. These responses are mediated by the dorsal column pathways and can be recorded from the scalp, much like SSEPs [50]. Conversely, the responses that are mediated by the motor components of a single nerve root result from either direct or indirect mechanical or electrical stimulation of these motor components and consist of EMG activity from a group of muscles known as a myotome. Myotomes are the motor complement to dermatomes and myotomal distributions as with dermatomal distributions can be quite variable between individuals. Whereas a myotome is a group of muscles that receives innervation from a specific spinal nerve root, most muscles receive their efferent innervation from several nerve roots. Like the afferent innervation for dermatomes, the amount and type of efferent innervation to a muscle will vary between individuals. Typically, during pedicle screw placements, EMG activity is recorded from several muscle groups using either surface or subdermal needle electrodes placed over or into these muscles. The selection of what muscle groups to monitor is dictated by which spinal nerve roots are at risk for irritation or injury. Although muscles typically receive their innervation from nerve roots associated with more than one spinal level, one spinal level generally predominates. The selection of what muscles to use for monitoring purposes is based on knowledge of this innervation. In most cases, recordings are made from muscles of the lower extremities because screw placement is generally done in the lumbosacral area of the spine where lumbar or sacral nerve roots are at risk. However, the mechanical advantages of pedicle fixation are not restricted to the lumbosacral spine. As a result, the use of pedicle screw placement in both the thoracic [51–60] and cervical [18, 61] regions of the spine has gained popularity. This has occurred despite the known risks to the spinal cord, nerve roots, and major blood vessels in these regions. In such cases, myogenic activity can be recorded from muscles innervated by cervical and/or thoracic nerve roots. Using this approach, monitoring of thoracic screw placement was first reported in 2001 [62]. However, this type of monitoring has had mixed success for thoracic screw placements [51, 54, 55, 57–59, 62]. In particular, the ability to detect medially misplaced thoracic screws has been very variable. As with the placement of lumbosacral instrumentation, nerve root injury can occur as a result of inferior, superior, or lateral pedicle screw placement. However, a thoracic screw that is misplaced too medially can result in a spinal cord injury. To avoid such occurrences, a technique has been developed that utilizes multipulse stimulation of the spinal cord, in particular the corticospinal tract, to elicit EMG responses from lower extremity musculature when a medial breach occurs [63]. This technique has been shown to successfully detect such occurrences [64, 65].
Because a single nerve root typically innervates more than one muscle, a choice between several muscles is possible when selecting a muscle for monitoring purposes. A list of those muscles that are commonly used for recording purposes and their innervation appears in Table 7.2. As with monitoring cranial nerve function, EMG monitoring for pedicle screw placement and for surgical procedures in the region of the cauda equina consists of detecting two types of activity: spontaneous or free-running activity and triggered EMG activity. The activity of interest in both cases will consist of compound muscle action potentials (CMAPs) , which are elicited either as a result of mechanical or electrical stimulation and are recorded from appropriate muscle groups (muscles that are innervated by nerve roots at risk for injury) using pairs of needle or surface electrodes. However, it has been reported that intramuscular electrodes are preferred over surface electrodes for obtaining these recordings [66]. When monitoring both types of activity, it is assumed that the muscles are sufficiently recovered from any muscle relaxants used so that activity can be elicited when stimulation occurs. For spontaneous activity, CMAP activity can generally be detected when train-of-four (TOF) testing produces only one twitch but having more than one twitch is desirable, because the amplitude of the CMAPS is reduced by muscle relaxants and small CMAPs may be missed if a patient is too relaxed. In most cases, nerve root irritation is an infrequent occurrence. As a result, the tracings of spontaneous EMG activity are generally flat and consist of little or no CMAP activity. When activity is present, it is generally associated with nerve root decompression (Fig. 7.2). Depending on the activated nerve root, the muscle groups being monitored, and the placement of the recording electrodes on or in these muscle groups will determine how many muscle groups are activated when stimulation occurs. This is true for triggered EMG activity as well.
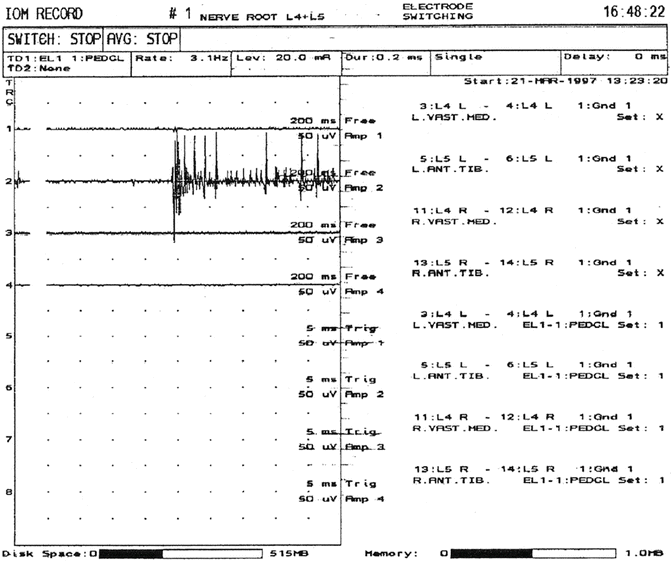
Table 7.2
Muscle groups commonly used to assess pedicle screw placements based on their innervation
Spinal region | Nerve root innervation | Muscle groups |
---|---|---|
Cervical | C2, C3, C4 | Trapezius, sternocleidomastoid |
C5, C6 | Biceps, deltoid | |
C6, C7 | Triceps, flexor carpi radialis | |
C8, T1 | Abductor pollicis brevis, Abductor digiti minimi | |
Thoracic | T1, T2, T3, T4 | Intercostals |
T5, T6 | Upper rectus abdominis, Intercostals | |
T7, T8 | Middle rectus abdominis, Intercostals | |
T9, T10, T11 | Lower rectus abdominis, Intercostals | |
T12 | Inferior rectus abdominis, Intercostals | |
Lumbar | L1 | Psoas |
L2, L3 | Adductor magnus | |
L3, L4 | Vastus medialis | |
L4, L5 | Anterior tibialis | |
L5, S1 | Peroneus longus | |
Sacral | S1, S2 | Medial gastrocnemius |
S2, S3, S4 | External anal sphincter |
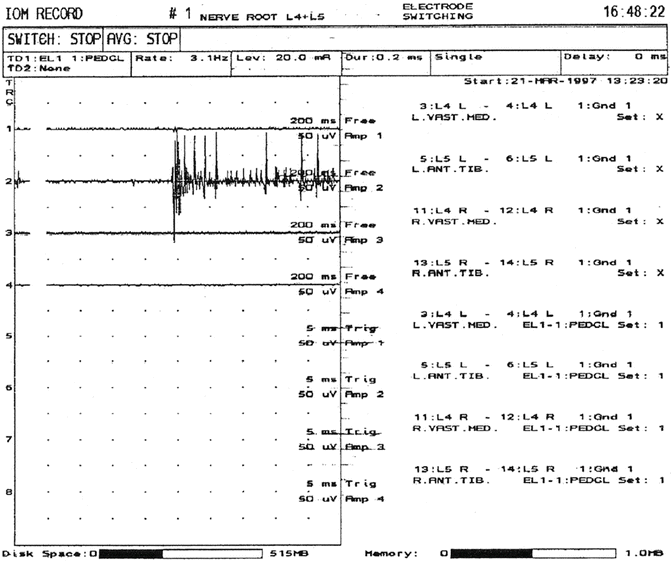
Fig. 7.2
Spontaneous EMG activity elicited from the left anterior tibialis muscle as a result of mechanical irritation of the left L5 nerve root. If the activity is of short duration (<1 s), it is generally considered not to be significant and rarely results in postoperative sequelae. However, if the activity is of a longer duration, it may be caused either by mechanical irritation of a nerve root that is already irritated, or a nerve root injury. Such activity warrants notification of the surgeon and should be avoided in order to minimize any chance of a postoperative deficit. Reprinted from Toleikis et al. [67]; with permission
Not all nerve roots react in the same manner when irritation occurs. Normal nerve roots and irritated or regenerating nerves in continuity react differently to mechanical forces. When such forces are statically or rapidly applied to normal nerve roots, they result in no elicited activity or only short-duration trains of CMAP activity [68]. However, when similar forces are applied to nerve roots that are already irritated or injured, they result in periods of firing of long duration. In cases of preexisting nerve root irritation, recordings of the activity will often consist of low-amplitude low-frequency activity even prior to mechanical irritation. Short aperiodic bursts of activity are common. Although attention should be paid to these, they are rarely indicative of neural injury. As indicated earlier, they are often associated with nerve root decompression and result from tugging and displacement, irrigation, electrocautery, metal-to-metal contact, or application of soaked pledgets. However, long trains of activity may be indicative of neural injury and are causes for concern and alarm. They are commonly related to sustained traction and compression and the more sustained the activity, the greater the likelihood of nerve root damage. When such activity occurs, it is imperative that the surgeon be notified so that corrective measures can immediately be taken. The presence of sustained spontaneous activity during both spinal and intracranial tumor removal surgeries has been reported to correlate with postoperative motor deficits [69, 70]. The presence of continuous activity has been used as a means for determining where spinal decompression is needed [71] and even the adequacy of decompression [72]. However, the presence of continuous EMG activity in patients undergoing surgery for tethered cord syndrome has been reported to be a poor predictor of postoperative outcome, with a sensitivity of 100 % but only a specificity of 19 %, and suggests that continuous EMG monitoring and the understanding of its significance remains an evolving technique [73]. When the significance of EMG activity is questionable, the combined acquisition of MEPs and spontaneous EMG activity has been reported to provide a means for corroborating the significance of the EMG activity. The presence of sustained or the sudden loss of EMG activity is an indication for acquiring an MEP [74]. The complementary use of both of these monitoring modalities has been shown to be a means for detecting and minimizing both nerve root and spinal cord injuries [74–80].
During spinal fusion procedures involving pedicle screws, triggered as well as spontaneous EMG activity are utilized for monitoring purposes. Triggered activity is elicited in two ways: either through direct stimulation of nerve roots at risk for threshold determination purposes [45] or via indirect nerve root stimulation when pedicle screws are stimulated in order to assess their placement [47, 49, 52, 53]. Ideally, these two stimulation techniques should be used in conjunction with one another. Direct stimulation is used to determine if a nerve root has an elevated stimulation threshold; generally, the result of chronic nerve root compression as is the case when a radiculopathy is present. The indirect stimulation technique relies on data obtained from the stimulation of healthy, normal functioning nerve roots and is used for assessing the placement of pedicle screws [47]. These data indicate that normal nerve roots have an average stimulation threshold of about 2 mA and that under these circumstances, an indirect stimulation warning threshold of about 10 mA is adequate for detecting misplaced pedicle screws [47]. However, if a direct stimulation threshold is elevated (above 2 mA), the indirect stimulation detection threshold needs to be elevated as well in order to compensate. It has been reported that the direct stimulation thresholds for some chronically compressed nerve roots are as high as 20 mA [81]. If such elevated thresholds are not taken into account, they can lead to false-negative findings, e.g., a screw that is reported to be adequately placed when in fact it is not. The physiologic factors that can contribute to false-negative findings largely pertain to the health status of the nerve roots that are involved because the criteria for assessing pedicle screw placements are based on results involving healthy nerve roots. In addition, stimulation thresholds may be elevated when assessing patients with metabolic disorders such as diabetes. The obvious way to avoid such findings is to directly stimulate each nerve root at risk in order to ensure that it is functioning normally before pedicle screw stimulation. If decompression is already being performed, this may seem like a reasonable thing to do. However, if this is not the case, routine laminotomies to explore each nerve root are time-consuming and not without some degree of risk. Therefore, most surgeons choose not to directly stimulate each nerve root at risk prior to pedicle screw stimulation testing. In cases when patients do exhibit signs of nerve root malfunction, it is strongly recommended that direct nerve root stimulation be utilized in order to establish stimulation thresholds.
Although most surgeons prefer to use indirect stimulation for testing the placements of pedicle screws, the technique can also be used to test for breaches in the pedicle wall by stimulating the markers that are placed in the spinal pedicles. Fluoroscopy is generally used by surgeons to identify the potential trajectory of pedicle screws, the taps, and/or the pedicle screw holes prior to screw placements. Stimulation is typically performed using a ball-tipped probe with a needle electrode used as an anode to complete the return current path (Fig. 7.3). The same assessment criteria are used to determine if the pedicle wall has been breached regardless of whether a marker, tap, screw, or hole is stimulated. Although the technique is generally the same regardless of who is providing monitoring services, there is some variability in the stimulation parameters used [47–49, 51–53, 67, 81–86]. Stimulation rates have ranged from 1 to 5 Hz with pulse durations of 50–300 μs. Typically, the stimulation intensity is gradually increased starting at or near zero until either CMAPs are elicited from one or more monitored muscle groups or a preset intensity limit is reached. The lack of elicited CMAP responses at intensities of 20–30 mA is probably a sufficient indication that a breach has not occurred even if nerve roots have elevated stimulation thresholds due to factors such as chronic nerve root compression. If EMG responses are elicited at stimulation intensities lower than a predetermined “warning threshold,” the course of action is to advise the surgeon to examine the pedicle hole or the pedicle screw placement. Just as the stimulation parameters may vary among groups, so too do the “warning thresholds.” Some have used stimulus intensities of 10 mA or greater, whereas others have used intensities of 8 mA or less as “warning thresholds” [84, 87–90]. As indicated earlier, normal nerve roots have a stimulation threshold of about 2 mA [47]. This threshold depends on both the amplitude of the pulsatile stimulus as well as the stimulus pulse width. Therefore, different “warning thresholds ” may result from different stimulus parameters. As indicated in Fig. 7.3, stimulation current can take many pathways but ultimately it will follow those that provide the least resistance. When a pedicle is intact, the pathway through bone is generally one of high resistance depending on bone density. However, when there is a breach of a medial or inferior pedicle wall, the fluid and tissue outside of the wall is likely to provide a path of least resistance. Now the distance from a stimulation probe or a screw becomes a factor. The intensity of the current that is present to excite a proximal nerve root will obey Coulomb’s Law (E = K[Q/r 2]), where E = stimulating current present at a nerve root, K = a constant, Q = applied stimulating current, and r = pedicle screw to nerve root distance, and will depend on the inverse square of the distance between the nerve root and the pedicle screw [91]. When testing a screw placement, the current intensity that is needed to elicit a CMAP response simply provides an indication of whether a breach is likely to have occurred and if it has, whether the position of the screw could potentially cause nerve root injury. A “warning threshold” of 10 mA is often used to assess whether such a breach has occurred; it is then verified either visually, or via palpation or fluoroscopy [47, 67]. In one study [67], it was found that on visual inspection by the operating surgeon, screws that require more than 7 mA of stimulation to elicit a CMAP response were associated with no breach, a cracked pedicle, or a slight medial exposure of one or two threads of the pedicle screw. In the surgeons’ judgment, in most cases, such findings posed no threat to nerve root functional integrity or of neural injury and the screws were generally left in place. It was only those screws with stimulation thresholds of 5 mA or less that were typically removed or redirected. Those screws with stimulation thresholds in the 5–7 mA range were equally likely to be left in place or removed. It is noteworthy that none of the screws that were left in place (except one) resulted in new postoperative deficits, including two screws with thresholds of 4 and 5 mA—an indication that low stimulation thresholds, unless they are far below a “warning threshold,” are unlikely to be associated with new postoperative neurologic findings. Therefore, a “warning threshold ” is only that and is meant to indicate when a screw has breached a pedicle wall such that its placement should not be ignored. The lower this “warning threshold” is set, the greater the likelihood that a screw with a lower stimulation threshold is misplaced and consideration should be given to having it removed. The results of other studies have suggested that a “warning threshold” should be set to at least 8 mA. However, it is more likely that only screws with stimulus threshold intensities less than 5 mA pose a real danger for nerve root injury [67, 88, 90, 92, 93]. Even in cases when radiographs are suggestive of adequate screw placement, low stimulation thresholds with resulting visual inspection or a postoperative CT have revealed that screw placements needed to be revised (Fig. 7.4).
