Electrodiagnosis in Pain Medicine
Nathan J. Rudin
To effectively treat acute and chronic pain, the clinician must understand its causes. Electrodiagnostic testing (EDX) can significantly improve that understanding. It complements the history, physical examination, laboratory testing, and imaging studies by providing quantitative assessments of the health, integrity, and function of muscle and nerve. Thoughtful electrodiagnostic studies can help narrow a differential or confirm a diagnosis. They can clearly identify the extent, severity, and location of nerve injury, muscle damage, or disease of the neuromuscular junction. Serial examinations can track disease progression, provide prognostic information, and measure recovery.
Clinicians should consider EDX whenever they suspect focal or diffuse neuromuscular disease. In these conditions, weakness, numbness, tingling, and pain are the most frequent complaints. The physical examination may include alterations in sensation, strength, reflexes, muscle bulk, muscle tone, and/or coordination. Imaging and laboratory studies may be normal or abnormal, depending on the condition. Common indications for EDX include nerve entrapment, radiculopathy, plexopathy, and traumatic injuries as well as metabolic disorders (e.g., diabetes mellitus), peripheral neuropathies, myopathies, and diseases of the neuromuscular junction. Special tests can assess disorders of cranial, pharyngeal, and perineal structures.
This chapter will familiarize the reader with the basic principles of EDX, provide guidance on interpretation of test reports, and help caregivers make optimal use of EDX when assessing patients in acute and chronic pain.
The Electrodiagnostic Laboratory
A reputable electrodiagnostic laboratory employs consistent techniques and norms to ensure accuracy and reliability. Test data should be interpreted by a trained and experienced physician, and electromyographers should be readily accessible for questions and case discussions from referring providers. To determine abnormal results, the laboratory should compare its results to documented and standardized normal values and should use the same norms consistently. Examiners within a laboratory should use the same techniques so that the same norms apply to all cases.
The laboratory should have good climate control set at a comfortable room temperature, as an excessively cold environment may adversely affect results. Electrical circuitry supplying the room should be properly shielded and grounded to ensure patient safety and to reduce line-current interference from equipment such as fluorescent light fixtures. Commercial electrodiagnostic units have been designed and rigorously tested to ensure safety and reliability.
The electrodiagnostic unit or “EMG machine” is primarily composed of a sensitive differential amplifier (high signal-tonoise ratio) and an electrical stimulator with precise control of stimulus duration and intensity, coupled to a computer for ease of data acquisition, analysis, and storage (Fig. 18.1). An active electrode (G1) is placed over the desired recording location, and a reference electrode (G2) is placed nearby. The amplifier subtracts the reference signal from the active signal to remove elements common to both, leaving only the information unique to the active electrode. The resulting signal (G1 to G2) is amplified, displayed, and stored.
Electrodiagnostic Tests
Most patients referred for EDX will undergo both nerve conduction studies (NCS) and needle electromyography (EMG). The entire procedure (both EMG and NCS) is often referred to as “EMG” when ordering the test or describing it to patients.
NERVE CONDUCTION STUDIES
NCS use electrical stimuli over a selected nerve to produce an action potential, which is recorded using electrodes placed further along the course of the stimulated nerve or the muscle it supplies. The resulting waveforms are analyzed and compared to normal values derived from a database of healthy individuals. The electrical stimulus is usually administered transcutaneously, with the stimulator probe (Fig. 18.2 shows an example)
positioned on the skin overlying the desired nerve. Recording electrodes are generally placed on the skin. In special circumstances (e.g., deep nerves, obesity, edema), stimulation and/or recording may be accomplished using needle electrodes advanced through the skin and placed adjacent to the desired structures. Patients should be warned before testing that the stimulated limb may twitch when the shock is delivered.
positioned on the skin overlying the desired nerve. Recording electrodes are generally placed on the skin. In special circumstances (e.g., deep nerves, obesity, edema), stimulation and/or recording may be accomplished using needle electrodes advanced through the skin and placed adjacent to the desired structures. Patients should be warned before testing that the stimulated limb may twitch when the shock is delivered.
Electrical stimulation should not be performed over or near pacemakers, implantable cardioverter-defibrillators, spinal cord stimulators, or other implanted medical electronics. In most patients, stimulation distant from the implant and its electrodes can be safely accomplished, but if there is any doubt, check with the device manufacturer before proceeding.
Electrical stimulation is generally perceived as a brief (0.1 to 0.2 ms), mildly uncomfortable “static” shock, repeated as stimulus amplitude is gradually increased. Once a maximal response is elicited from the nerve being studied, stimulation is terminated and the action potential is analyzed.
Parameters recorded during NCS include action potential latency (time between stimulation and initiation or peak of action potential), action potential amplitude, and nerve conduction velocity (NCV). Latency and NCV reflect myelin health; demyelination will prolong the latency and slow the NCV. Amplitude reflects axonal integrity; axonal loss or injury may produce decreased amplitude and temporal dispersion of the action potential. The distribution of abnormalities can distinguish focal, segmental, and diffuse neuropathic processes. Stimulation at multiple points along the nerve allows measurement of NCV across different nerve segments. Where abnormal results are seen, comparison to a contralateral asymptomatic structure, where available, provides a valuable intra-individual control.1 Varieties of NCS are described in detail in the following text along with their specific applications.
Sensory nerve conduction studies (SNCS): SNCS provide detailed assessment of sensory nerve function. Nerve stimulation produces a sensory nerve action potential (SNAP), which is recorded over the same peripheral nerve at a distance from the stimulation point. Figure 18.3 illustrates a standard setup for a median SNCS (Fig. 18.3A) and the resulting normal SNAP (Fig. 18.3B). Because nerves conduct bidirectionally from the point of stimulation, SNCS may be performed orthodromically (in the physiologic direction, stimulating distally, and recording proximally) or, as in this case, antidromically (stimulating proximally and recording distally).
Figure 18.4 shows an orthodromic SNCS comparing distal conduction of the median and ulnar nerves across the wrist (Fig. 18.4A). This study is highly sensitive and specific for the presence of carpal tunnel syndrome (median entrapment neuropathy at the wrist), which is demonstrated here (Fig. 18.4B). The two nerve segments are of equal lengths and should have approximately the same latency and NCV. In this case, the median SNAP latency is appreciably prolonged, indicating conduction delay. Median amplitude is also lower than the laboratory’s norm of 51 µv. Values for the ulnar SNAP are normal.
SNCS results may be altered by cold skin temperatures, potentially leading to incorrect diagnoses. Warming the skin to ≥32°C will minimize this cause of error.
Like electrical cables, peripheral nerves are composed of numerous nerve fibers with varying functions, diameters, and degrees of myelination. The SNAP elicited during SNCS primarily reflects the response of faster conducting, more heavily myelinated afferent fibers. Responses from smaller diameter, less myelinated fibers (e.g., C and Aδ fibers transmitting pain and temperature signals) may not be detected. Standard SNCS therefore lack sensitivity for detecting small-fiber dysfunction in painful conditions.2 Alternate techniques, such as quantitative sensory testing (see the following text) and skin punch biopsy with nerve fiber staining,3 can augment the physical examination to help characterize small-fiber abnormalities.
Motor nerve conduction studies (MNCS): MNCS characterize the health of motor nerve fibers and the neuromuscular junction. Nerve stimulation produces a compound muscle action potential (CMAP), which is recorded over a muscle innervated by the stimulated nerve. Figure 18.5 shows the
setup (Fig. 18.5A) and normal results (Fig. 18.5B) for an ulnar MNCS recording at abductor digiti minimi. The nerve is stimulated at multiple sites along its length; distances between stimulation points are measured and recorded. Onset latencies and CMAP amplitudes are measured. Motor NCV in meter per second (m/s) is calculated by dividing the distance between points (δd, in millimeter) by the change in time between stimulation points (δt, in millisecond).
setup (Fig. 18.5A) and normal results (Fig. 18.5B) for an ulnar MNCS recording at abductor digiti minimi. The nerve is stimulated at multiple sites along its length; distances between stimulation points are measured and recorded. Onset latencies and CMAP amplitudes are measured. Motor NCV in meter per second (m/s) is calculated by dividing the distance between points (δd, in millimeter) by the change in time between stimulation points (δt, in millisecond).
Repetitive nerve stimulation studies (RNS): RNS are a variant of MNCS that can identify disorders of the neuromuscular junction. Rapid repetitive stimulation (usually 2 to 3 Hz) is performed, as illustrated in Figure 18.6A. A decrement in CMAP amplitude during RNS is abnormal and suggests a disorder of the neuromuscular junction. CMAP decrement with RNS is illustrated in Figure 18.6B. The response of CMAP amplitude to exercise can differentiate between neuromuscular disorders.
H-reflex studies: H-reflex studies are modified MNCS that help to identify some radiculopathies and peripheral neuropathies. The H-reflex is an analog of the muscle stretch reflex. Most commonly, the tibial nerve is electrically stimulated at the popliteal region (analogous to an Achilles tendon tap) and the reflex response (H-reflex) in the soleus muscle is measured. A unilaterally absent or delayed tibial H-reflex may reflect an S1 radiculopathy, tibial neuropathy, or sciatic neuropathy. Bilateral abnormalities may be seen in peripheral neuropathies and in older individuals. H-reflexes may also be found by stimulating the median nerve at the elbow and recording at flexor carpi radialis. They are more widespread in hyperreflexic conditions (e.g., myelopathy) and in infants.1
Another modified MNCS, the F-wave study, allows indirect observation of nerve conduction between the dorsal root ganglion (DRG) and the spinal cord, permitting sensitive evaluation of polyneuropathies including Guillain-Barré syndrome (GBS), radiculopathies, and proximal nerve dysfunction.4 Upon peripheral nerve stimulation, the stimulus travels bidirectionally, producing (1) a CMAP and (2) a small, later potential (F-wave) from antidromic activation of a small pool of alpha-motoneurons. Their appearance and latency are variable (Fig. 18.7).
Cranial nerve studies: The trigeminal nerve reflexes (blink reflex, jaw jerk, and masseter inhibitory reflex) can be studied using special EDX,5 providing valuable clues to lesions of cranial nerves and/or the brain stem.6 The blink reflex (corneal reflex) is the most often studied and can provide information regarding the integrity of the ophthalmic division (V1) of the trigeminal nerve, the facial nerve (efferent limb), and the brain stem. In the normal blink reflex study, electrical stimulation of the supraorbital nerve unilaterally is followed by a facial nerve blink response bilaterally.
Sacral nerve studies: Special techniques are used to examine sacral sensory and motor innervation, including studies of the bulbocavernosus and clitorocavernosus reflexes (pudendal and perineal nerves). Along with EMG of pelvic floor muscles, these studies can help diagnose sacral nerve dysfunction due to cauda equina syndrome, peripheral neuropathy, focal neuropathies, pelvic pain syndromes, neurogenic erectile dysfunction,7 and Tarlov cysts.8 They may, however, be quite uncomfortable for the patient, so they are reserved for cases where the findings are crucial for treatment decisions.
NEEDLE ELECTROMYOGRAPHY
Needle EMG uses needle electrodes to directly assess the electrical activity of motor units, providing extensive information about their integrity, innervation, function, and recruitment. A skilled electromyographer can identify a broad range of disorders causing denervation, inflammation or other destruction, or failure of neuromuscular transmission.
EMG is indicated whenever muscular dysfunction is suspected. It can assist with the diagnosis of neuropathies, radiculopathies, or plexopathies; myopathies (inflammatory, congenital, or other); disorders of the neuromuscular junction; and motor neuron diseases. Where NCS has indicated abnormalities, EMG can help clarify their distribution and severity; also, EMG often detects significant abnormalities when NCS are normal (radiculopathy is a common example). Special electrodes and techniques permit the assessment of single muscle fibers, which can clarify diagnosis in some conditions, particularly neuromuscular junction disorders.9
The electromyographer chooses which nerves and muscles to examine, and which techniques to employ, based on the history and physical examination. A strong command of neuroanatomy is required. The electrodiagnostic exam will ideally screen for most conditions on the differential diagnosis. For example, when pathology in a nerve structure is suspected, the electromyographer will perform the appropriate NCS and then will use EMG to assess multiple muscles supplied by that structure. The choice of nerves and muscles will vary with suspicion of peripheral nerve, plexus, nerve root, muscle, or other neuromuscular pathology. Findings on earlier tests often change the choice of tests performed later as the differential is narrowed or widened.
Where it is important to diagnose a nerve lesion early, electrodiagnostic studies within 7 to 10 days of injury can localize
nerve lesions and quantify the severity of nerve injury. Studies performed 3 to 4 weeks after injury provide information on muscle denervation and can help with surgical planning. Studies done 2 to 6 months after injury can quantify healing and provide prognostic information.10 In chronic illnesses such as motor neuron disease and peripheral neuropathies, serial studies document disease course, progression, and prognosis.
nerve lesions and quantify the severity of nerve injury. Studies performed 3 to 4 weeks after injury provide information on muscle denervation and can help with surgical planning. Studies done 2 to 6 months after injury can quantify healing and provide prognostic information.10 In chronic illnesses such as motor neuron disease and peripheral neuropathies, serial studies document disease course, progression, and prognosis.
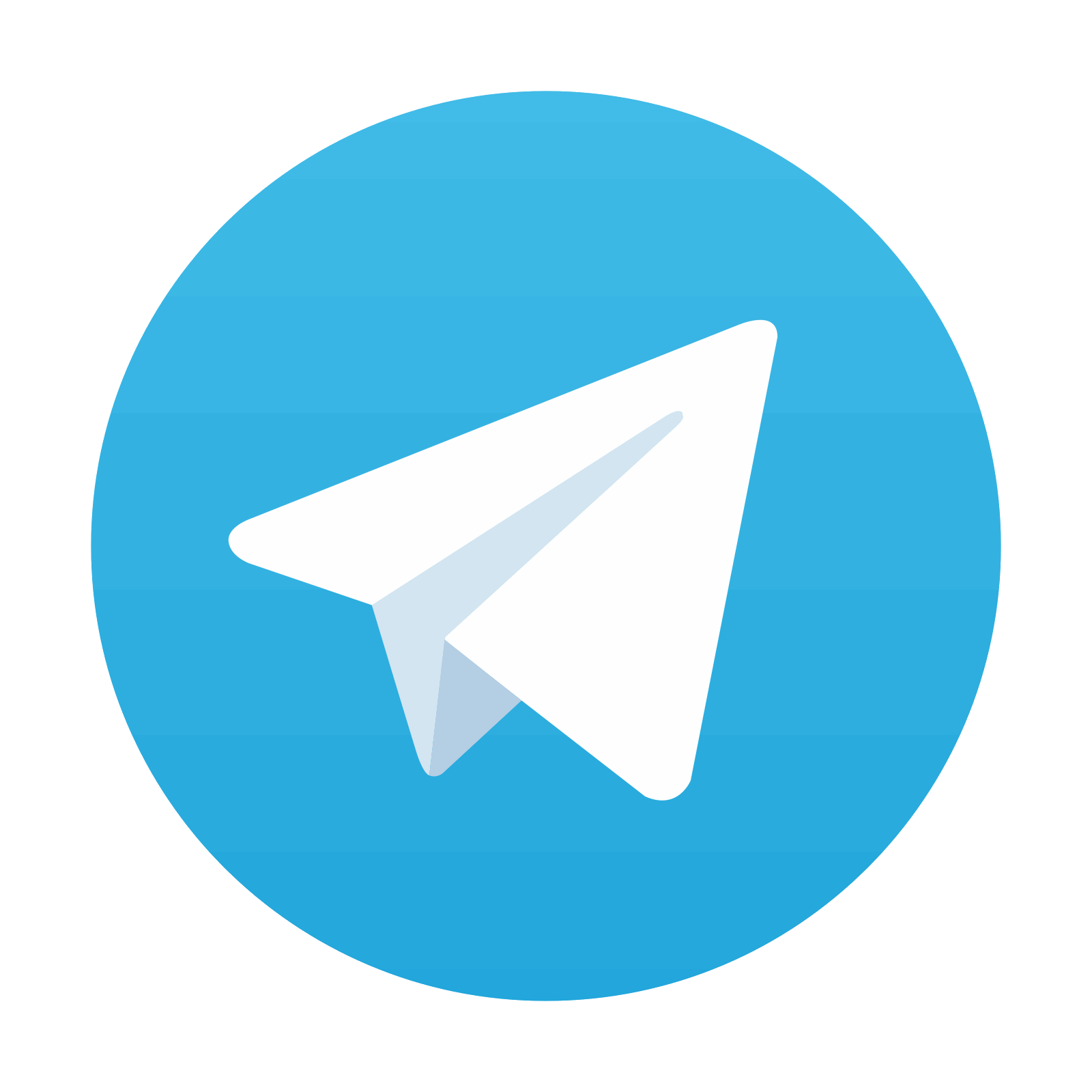
Stay updated, free articles. Join our Telegram channel

Full access? Get Clinical Tree
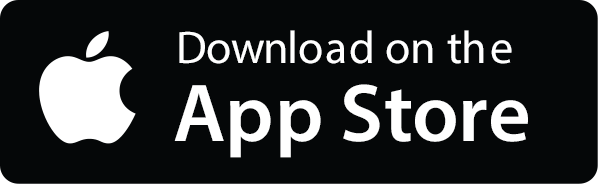
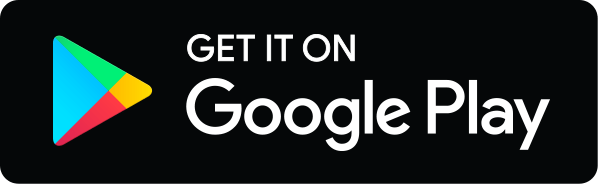