Peripheral Pain Mechanisms and Nociceptor Sensitization
Michael S. Gold
Pain has been categorized by duration (acute vs. chronic), location (superficial or deep; cutaneous, bone/joint, muscle, or viscera), and cause or type (inflammatory, neuropathic, cancer). Generally, activation of and/or ongoing activity in specific subpopulations of primary afferent neurons underlies the experience of pain regardless of how it is categorized. Accordingly, primary afferents are key players in understanding mechanisms of, and managing, pain.
Sir Charles Sherrington anticipated by many decades the existence sensory receptors that respond to noxious stimuli, that he called nociceptors and thereby provided for us the operational definition of stimuli that are noxious (i.e., stimuli that damage or threaten damage of tissue). Two considerations are important to this discussion. First, Sherrington functionally defines the nociceptor by its response to a noxious stimulus (e.g., a nociceptive withdrawal reflex, pain). Second, the definition of an applied stimulus as noxious is based on the response to the stimulus applied to skin and subcutaneous structures.
Sherrington’s definition of a nociceptor continues to the present day. However, the term has undergone change and challenge over the past 100 years and may be nearing the end of its utility in the face of the growing understanding of the heterogeneity in the neurons that may not fit so comfortably under this umbrella term. It is therefore important to consider, within the context of our current knowledge, how a nociceptor is defined, identified, and studied, as the interpretation of this information will directly affect the management of pain.
All would agree that a nociceptor is a sensory receptor which, when activated or active, can contribute to the experience of pain. Nociceptors are present in skin, muscle, joints, and viscera, although the density of innervation (i.e., the number and distribution of sensory endings) varies between and within tissues. As originally described by Sherrington, a nociceptor is the peripheral sensory terminal (i.e., the site of energy transduction—see following section), although commonly the term is used to also include the cell body (in a dorsal root, trigeminal, or nodose ganglion) and its central termination in the spinal cord or brainstem. Beyond this, agreement about important features of nociceptors is less uniform. One of the best examples of why this definition is becoming problematic is the observation that injury-induced changes in the central nervous system underlie the emergence of allodynia, pain in response to normally innocuous stimuli. Allodynia is problematic for this definition of nociceptor because pain is mediated by activity in low-threshold afferents that in the absence of tissue injury would, if anything, contribute to the suppression of pain. That is, these afferents would not be considered nociceptors despite the fact that they contribute to the experience of pain.
Furthermore, because stimuli adequate for activation of nociceptors differ between tissues (e.g., tissue damage is not always required), defining a noxious stimulus has become a challenge. For example, some nociceptors in skin and joints and most nociceptors in the viscera have low thresholds for mechanical activation that do not conform to the condition that stimulus intensity must be either damaging or threaten damage. Further, so-called “silent” or “sleeping” nociceptors are unresponsive to intense mechanical stimulation (and are better denoted as mechanically insensitive nociceptors) but develop spontaneous activity and mechanosensitivity after exposure to inflammatory and other endogenous mediators. These types of nociceptors—low threshold and sleeping—as well as other subpopulations of sensory neurons that may contribute to the sensation of pain following tissue injury are considered further in the discussion of sensitization in the following section.
Functional Characterization of Nociceptors
As suggested earlier, there are many types of nociceptors, our knowledge of which has been advanced by human psychophysical studies while recording from afferent fibers (Box 3.1). In human skin, for example, there exist nociceptors that respond only to mechanical, only to cold thermal, or only to hot thermal stimuli as well as those that are insensitive to both mechanical and heat stimuli (mechanically insensitive or sleeping nociceptors).1,2 The most abundant is the polymodal nociceptor, which responds to mechanical, thermal, and chemical stimuli. In general, nociceptors that innervate skin have the broadest range of modality selectivity, whereas nociceptors innervating deeper structures tend to be less modality-selective and more polymodal in character.3 For example, mechanical sensitivity is a prominent feature of visceral and joint nociceptors because stimuli adequate for their activation include hollow organ distension and overrotation, respectively. Many of these nociceptors also respond to chemical and/or thermal stimuli as well, although the functional significance of thermal sensitivity in deep tissues is uncertain. An important characteristic of polymodal nociceptors, whether the modalities of stimulation to which they respond are two or all three, is that when sensitized (e.g., by an inflammatory insult), responses to the other modality or modalities of stimuli to which it responds are all increased.4 That is, it is not only the mechanosensitive modality, for example, that becomes sensitized, but other modalities to which it responds are sensitized as well.
With respect to mechanosensitivity, nociceptors at the opposite extremes of sensitivity are most illustrative of the limitations of even a functional definition of a nociceptor. Nociceptors with low mechanical thresholds for response and those with very high mechanical thresholds for response (i.e., sleeping nociceptors) are both clinically important. Mechanosensitive sensory neurons with low thresholds for response have long been classified as non-nociceptors (because it was considered that nociceptors had to have response thresholds in the noxious range). Some mechanosensitive skin, joint, and many visceral sensory neurons have low thresholds for response (i.e., in the nonnoxious range) but possess characteristics that suggest an important role in pain. First, they encode stimulus intensity well into the noxious range and, moreover, typically give greater responses to all intensities of stimulation than do nociceptors with high mechanical thresholds for response. Second, they sensitize after tissue insult. Unlike nociceptors with low mechanical thresholds, mechanically insensitive or sleeping nociceptors normally provide no information to the central nervous system but after tissue insult become spontaneously active and mechanosensitive.5
BOX 3.1 Microneurography
The development of a method to record from human nerve fibers in situ,191,192 termed microneurography, provided an unparalleled opportunity to expand our knowledge about peripheral sensory receptors, including nociceptors. The method involves percutaneous insertion of the tip of a sharp, insulated metal microelectrode into a nerve (e.g., peroneal or radial nerve) and the application of search stimuli to sites distal to the electrode. In earlier work, mechanical search stimuli (e.g., von Frey filaments) were used, and accordingly, only mechanosensitive afferents were studied. An electrical search stimulus (surface electrode), however, has become favored because the electrical stimulus identifies afferent fibers independent of sensitivity to natural stimulation. After an afferent fiber is isolated, the innervation territory can be drawn on the skin and the adequate, natural stimulus/stimuli determined.
Because microneurography can be easily coupled with a psychophysical approach, human subjects are able to describe stimulus-produced experiences (e.g., pain) while recording from single afferent fibers. Microneurography also has been expanded to include intraneural electrical stimulation of the fiber through the recording electrode, providing additional insight into the qualities of sensation produced, for example, by low- and high-frequency stimulation in addition to qualities associated with natural stimulation. Microneurography has confirmed in psychophysical experiments sensations associated with activation of rapidly adapting (flutter, vibration) and slowly adapting (pressure) cutaneous mechanoreceptors, Aδ-mechanonociceptors (AM[mechano]; sharp pain), C-polymodal nociceptors (CM[mechano]H[heat]; dull, burning [heat] pain), and group IV muscle nociceptors (cramping pain).
Electrical search strategies have revealed a wider range of nociceptors, including190 A-mechanoheat (AMH), which have similar heat thresholds as CMH (C-polymodal) fibers and also typically respond to chemical stimuli; C-mechanonociceptors (CM), C-heat (CH); C-mechano- and heat-insensitive (CMiHi, or sleeping nociceptors); and C-mechanoinsensitive-histamine responsive (CMiHis+, or itch fibers193). Microneurography has also been extended to psychophysical study of pathologic pain states in humans. In a study of patients suffering from erythromelalgia, a condition characterized by painful, red, and hot extremities, a proportion of CMiHi fibers were found to be spontaneously active or sensitized to mechanical stimuli. Because CMiHi fibers also mediate the axon flare reflex, their hyperexcitability was considered to contribute to the patients’ ongoing pain and tenderness as well as the redness and warming in this pain syndrome. In patients with painful peripheral neuropathy, Ochoa et al.194 reported hyperexcitability in CMH and CMiHi fibers. Signs of hyperexcitability included reduced thresholds to mechanical and heat stimuli, spontaneous activity, and increased responses to stimulation. In diabetic neuropathic pain patients, Ørstavik et al.195 found that the ratio of CMH to CMiHi fibers was reduced by about 50%, apparently due to loss of mechanical and heat responsiveness in CMH fibers.
These and future studies will help to understand which nociceptors (and non-nociceptors) in which conditions contribute to spontaneous, ongoing pain as well as stimulus-evoked pain and what therapeutic strategies are most effective.
Identification of Putative Nociceptors
As indicated earlier, nociceptors are defined classically in a functional context. However, in experimental situations where function cannot be assessed, other criteria to classify a neuron as a nociceptor have been advanced. These include the presence or absence of axon myelination, cell size, and/or cell content (e.g., peptide or ion channel) as well as central termination pattern. Sensory neurons commonly identified as nociceptors are those with unmyelinated (C-fiber) axons, small cell body diameters (<20 or 25 µm), that terminate in the superficial layers of the spinal cord dorsal horn. The presence or absence of certain markers (e.g., the tetrodotoxin (TTX)-resistant sodium channel NaV1.8, the transient receptor potential vanilloid receptor TRPV1) have been used to identify subsets of nociceptors. More recently, with the ability to analyze many genes expressed in a single cell, patterns of gene expression have been used to further define subpopulations of nociceptors.6,7 With respect to cell body size and myelination, it should be appreciated that there exist some large diameter cells with heavily myelinated and rapidly conducting axons that have been documented functionally to be nociceptors.8 Conversely, many non-nociceptors have unmyelinated axons, and thus, axon myelination or cell diameter cannot be applied as reliable criteria to define a nociceptor. Similarly, identifying nociceptors by content or by what genes they express has limitations. Two examples of this are that (1) cells other than nociceptors express TRPV1 and (2) the features of the subset of nociceptors in the mouse skin that stain positive for the isolectin B4 do not apply to the visceral innervation.9 And although patterns of gene expression may enable identification of additional subpopulations of nociceptive sensory neurons,10 the extent to which any particular pattern reliably reveals a neuron’s function remains to be established.
The fact that no single criterion can be used to identify all nociceptors combined with the growing number of examples to the ways in which these neurons are heterogeneous is at the core of a growing concern over the utility of the term. In addition to the anatomical, biochemical, and physiologic heterogeneity in the afferent population, generally referred to as nociceptors, there is functional heterogeneity. As will be discussed later, this functional heterogeneity is manifest both within the context of nociceptive signaling (i.e., subpopulations of nociceptors may underlie distinct “types” of pain such as cold allodynia or thermal hyperalgesia) and in the context of nonnociceptive function (i.e., such as the maintenance of tissue integrity).
Even the two functional properties that have been viewed as common to all nociceptors, that they encode stimulus intensity into the noxious range and they sensitize, are, at best, generalities. As noted earlier, the so-called mechanically insensitive afferents shown to play such a prominent role in visceral pain11 and the burning pain associated with capsaicin12 do not appear to code stimulus intensity in the noxious range. Similarly, a variety of nociceptive afferents may be desensitized under the appropriate conditions.13,14,15,16 The link between the stimulus-response properties of a given subpopulation of afferents and the functional role of these neurons in the context of nociceptive signaling has been further complicated by results of selective ablation/silencing studies in mice, where modality specific deficits in nociceptive processing are associated with the silencing of specific subpopulations of afferents despite electrophysiologic data suggesting that the majority of the afferents silenced/ablated are polymodal. For example, the majority of cutaneous neurons in the mouse that express the Mas1-related G protein-coupled receptor D (MrgprD) are polymodal nociceptors,17 yet mice in which this subpopulation of neurons has been ablated respond normally to changes in temperature, demonstrating, instead, a relatively selective mechanosensitivity deficit.18 Conversely, the voltage-gated sodium channel (VGSC) NaV1.8 is enriched in nociceptive afferents that are responsive to both noxious thermal (TRPV1-expressing) and mechanical (MrgprD-expressing) stimuli, yet mice in which NaV1.8-expressing neurons have been ablated respond to noxious heat but not noxious mechanical pressure or cold stimuli.19 Minimally, these results suggest that the behavioral response to noxious stimulation involves a number of factors in addition to the types of afferents that are activated.
All of this heterogeneity does not bode well for the development of broadly acting novel analgesics. That is, given our current understanding of the complexity of peripheral pain mechanisms, it seems unlikely that the range of intensities
within any one modality (thermal, mechanical) that is encoded by a nociceptor and initiates nociceptive transmission involves only a single voltage- or ligand-gated channel. Similarly, even if the ability to sensitize is one means of functionally defining a peripheral neuron as a nociceptor, the endogenous mediators and factors that contribute to an increase in the excitability of nociceptors (i.e., sensitization) are numerous and synergetic and differ in different pain conditions (e.g., inflammation, nerve injury). A sampling of the complexities and contributors to sensitization are discussed in the following section.
within any one modality (thermal, mechanical) that is encoded by a nociceptor and initiates nociceptive transmission involves only a single voltage- or ligand-gated channel. Similarly, even if the ability to sensitize is one means of functionally defining a peripheral neuron as a nociceptor, the endogenous mediators and factors that contribute to an increase in the excitability of nociceptors (i.e., sensitization) are numerous and synergetic and differ in different pain conditions (e.g., inflammation, nerve injury). A sampling of the complexities and contributors to sensitization are discussed in the following section.
Why include in a clinical textbook of pain management a chapter on peripheral pain mechanisms and nociceptors? There are many reasons. First, in most cases, blockage of peripheral nociceptor activity removes the “drive” for the experience of pain. Further, if a primary goal is to develop mechanism-based strategies for pain management, it is critical that characteristics of a key player—the nociceptor—are fully understood. Finally, nociceptor characteristics change as the local environment in which they reside changes (inflammation, nerve injury, etc.). I discuss in the following section how nociceptors are activated, differ in different tissues, and contribute to the experience of pain and how their behavior changes when they become sensitized. Where possible, we have added relevant clinical examples and have inserted text boxes to elaborate on key issues.
Nociceptor Characteristics
ANATOMY OF THE NOCICEPTOR
As stated earlier, nociceptors are sensory neurons with a cell body located in dorsal root, trigeminal, or nodose ganglia. All sensory neurons arising from these ganglia are pseudo-unipolar neurons with a central process terminating in the central nervous system (e.g., spinal dorsal horn) and a peripheral process terminating in a peripheral target such as the skin, muscle, or viscera. Both central and peripheral processes terminate in a branching pattern referred to as a terminal arbor. The extent of the peripheral arbor depends on the afferent type and site of innervation with the general rule that the higher the spatial resolution for sensory discrimination, the smaller the terminal arbor. In contrast to low-threshold afferents that are responsive to nonnoxious stimuli such as brush or vibration and which terminate in specialized structures, such as Ruffini endings or Merkel disks,20,21 nociceptors are said to have “free” (unencapsulated) nerve endings because peripheral terminals of these afferents do not appear to be associated with any specific cell type.22
Both light and electron micrographic analyses of peripheral nociceptor terminals reveal complex anatomical structures. As suggested earlier, the structure of the terminal arbor varies with target of innervation. There is also evidence that subpopulations of nociceptive afferents have distinct terminal arbor patterns within the same structure. For example, the terminal arbor morphology of cutaneous C fibers varies according to whether the C fiber is peptidergic (i.e., expresses substance P or calcitonin gene-relative peptide) or expresses the MrgprD.23 On the other hand, the terminal morphology of a subpopulation of high-threshold Aδ fibers that appear to mediate the pain associated with hair pull24 is similar to that of a subpopulation of low-threshold mechanosensitive Aβ fibers that appear to underlie the sensation of gentle stroking of the skin.25 Evidence also suggests that distinct but overlapping subpopulations of nociceptors may signal distinct aspects of the painful experience (i.e., sensory/discriminative vs. emotional/motivational),26 suggesting that it may someday be possible to selectively treat the suffering associated with chronic pain while still enabling patients to appropriately respond to noxious stimuli in their environment.
Four distinct events are necessary for a nociceptor to convey information to the central nervous system about noxious stimuli impinging on peripheral tissues (Fig. 3.1—the events are discussed fully in the following paragraphs). First, “energy” from the stimulus (mechanical, thermal, or chemical) must be converted into an electrical signal. This process, referred to as signal transduction, results in a generator potential or depolarization of the peripheral terminal. Second, the generator potential must initiate an action potential, the rapid “all or nothing” change in membrane potential that constitutes the basic unit of electrical activity in the nervous system. This process is sometimes referred to as transformation. Third, the action potential must be successfully propagated from the peripheral terminal to the central terminal. And fourth, the propagated action potential invading the central terminal must drive a sufficient increase in intracellular calcium ions to enable release of enough transmitter to initiate the whole process once again in the second-order neuron. Distinct sets of proteins underlie each of these processes and are, therefore, the targets of a wide variety of therapeutic interventions.
STIMULUS TRANSDUCTION
An important implication of the fact that nociceptive afferents terminate in “free nerve endings” is that they are not dependent on other cell types for the transduction of a noxious stimulus. That is, proteins responsible for transduction should be intrinsic to the nociceptor. Consistent with this suggestion, isolated sensory neurons are responsive to thermal (both heating27,28 and cooling29,30), mechanical,31,32 and a wide variety of chemical stimuli, including both endogenous33,34 and exogenous35,36 compounds that activate nociceptors in vivo. Proteins involved in the transduction of each stimulus modality have been identified (see Gold37 for review).
With the exception of transient receptor potential (TRP) channels (see the following discussion), two-pore potassium channels (K2P), and possibly the acid-sensing ion channels (ASICs), chemotransducers are, in general, only activated by chemical stimuli (and not also mechanical or thermal stimuli) and encompass various families of proteins that respond to specific molecules such as adenosine triphosphate (ATP)38 and protons.39 There is also compelling evidence to support the suggestion that different members of the TRP superfamily underlie thermal transduction of temperatures ranging from the very cold (TRPA140) to the very hot (TRPV241), with receptors for cool,42 warm,43,44 and hot45 in between. Subsequent research, however, suggests that in contrast to traditional chemoreceptors, TRP family members are not modality-specific as all are activated by specific chemicals46 and several contribute to mechanical transduction.32,47,48
Because the only sensation associated with TRPV1 activation is pain, this receptor has received considerable attention from pain researchers. Data from an array of studies paint a picture of TRPV1 as an excellent example of a polymodal receptor; it is activated by exogenous compounds such as capsaicin and resiniferatoxin, endogenous compounds ranging from protons to lipids, as well as noxious heat.49 TRPV1 is also present on the central terminals of nociceptive afferents where it also facilitates transmission of noxious mechanical stimuli.50,51,52 Furthermore, excessive activation of the receptor with compounds such as capsaicin results in desensitization of the nociceptive terminal to all modes of stimuli, a process that underlies the therapeutic efficacy of topical capsaicin application.53 More recently, intrathecal application of resiniferatoxin has been used to selectively ablate the central terminals of TRPV1 containing nerve terminals, resulting in a sustained block of nociceptive transmission.54,55 There is also evidence that TRPV1 receptor antagonists may have some analgesic efficacy, although their therapeutic potential may be limited by a small but significant hyperthermia associated with systemic administration of blood-brain barrier permeable analogs.53
Of the three modalities of noxious stimuli, molecular mechanisms of mechanotransduction remain the most elusive. Many mechanically sensitive proteins have been identified,56 but none appears to be both necessary and sufficient for mechanotransduction in nociceptive afferents. Data from null mutant mice, where the deletion of a single putative mechanotransducer results in an increase in mechanosensitivity in one population of afferents and a decrease in others,57,58,59 suggests that several different proteins are likely to work together in specific subpopulations of afferents to enable responses to specific forms of mechanical stimuli (e.g., stretch or compression). This picture is complicated further by the observation that some mechanotransducers such as piezo2 contribute to mechanotransduction in specific subsets of sensory neurons.60 That even these more specialized forms of mechanosensitivity reflect intrinsic properties of afferents are suggested by the emergence of mechanosensitivity at the severed ends of a subpopulation of axons within hours of transection.61 Despite the slow progress in this area, identification of a nociceptor specific mechanotransducer blocker remains an active area of investigation because of its therapeutic potential in light of the fact that mechanical hypersensitivity is the primary complaint associated with the vast majority of chronic pain syndromes.62,63,64
Whereas mechanotransduction is an intrinsic property of many nociceptors, there is evidence that epithelial cells may also be mechanosensitive.65,66,67 Because these cells may store and release transmitters such as ATP, it has been suggested that afferent activity evoked with mechanical stimulation of peripheral structures such as the bladder,65 gastrointestinal tract,68 and skin66,67,69 may be secondary to the transduction event that has occurred in the epithelial cell that subsequently releases a chemical mediator capable of activating nearby nociceptor terminals. The implication of this mechanism from a therapeutic perspective is that it may be possible to attenuate mechanical
hypersensitivity in several peripheral tissues with the appropriate antagonist of the responsible chemoreceptors on nociceptive afferents.70 Consistent with this idea, there is evidence that mechanical hypersensitivity observed in several visceral structures can be attenuated with ATP receptor antagonists.71 That a similar mechanism may contribute to chemotransduction, at least in the intestine, was recently suggested by the observation that enterochromaffin cells are not only activated by a variety of chemicals but are electrically excitable and form synaptic connections with primary afferents.72 However, serotonin appears to be the primary transmitter underlying the signaling from these cells to primary afferents, possibly accounting for the therapeutic efficacy of inotropic serotonin receptor (i.e., 5-HT3) antagonists for the treatment of visceral pain73
hypersensitivity in several peripheral tissues with the appropriate antagonist of the responsible chemoreceptors on nociceptive afferents.70 Consistent with this idea, there is evidence that mechanical hypersensitivity observed in several visceral structures can be attenuated with ATP receptor antagonists.71 That a similar mechanism may contribute to chemotransduction, at least in the intestine, was recently suggested by the observation that enterochromaffin cells are not only activated by a variety of chemicals but are electrically excitable and form synaptic connections with primary afferents.72 However, serotonin appears to be the primary transmitter underlying the signaling from these cells to primary afferents, possibly accounting for the therapeutic efficacy of inotropic serotonin receptor (i.e., 5-HT3) antagonists for the treatment of visceral pain73
Aberrant expression of transducers may play a significant role in chronic pain associated with tissue injury. The presence of a functional transducer at a site other than the peripheral terminal may underlie the emergence of ectopic activity and contribute to ongoing or spontaneous pain. Such changes have been most extensively detailed following nerve injury, where, as mentioned earlier, mechanical sensitivity is detectable in cut axons within hours of injury61 and may persist in neuromas indefinitely.74 Similarly, chemosensitivity, particularly to adrenergic agonists, develops at both the cut ends of nociceptive afferents75 as well as within the ganglia itself,76 contributing to ectopic activity arising from both the site of injury and from the ganglia. The emergence of ectopic activity arising from sites distant to the site of injury may explain why interventions targeting the site of injury are unsuccessful.
PASSIVE ELECTROPHYSIOLOGIC PROPERTIES AND THE SPREAD OF THE GENERATOR POTENTIAL
A generator potential at a primary afferent terminal ending is not equivalent to an action potential propagated along that afferent’s axon. The generator potential decays over distance from the point of origin as a function of membrane resistance, membrane capacitance, and internal resistance of the nerve terminal and may or may not be propagated beyond the terminal ending. Generally considered stable properties, there is evidence for dynamic remodeling of the terminal arbor of central nervous system neurons77 which may influence both membrane capacitance and internal resistance; it remains to be determined whether such changes may also impact passive conduction of generator potentials in peripheral terminals. In contrast, a number of ion channels have been identified that may establish resting membrane resistance and therefore the spread of the generator potential within the terminal arbor.78 This issue is important to nociceptive signaling because action potential initiation does not always occur at the site of stimulus transduction.79 Consequently, the magnitude of the generator potential at the site of action potential initiation must be greater than or equal to action potential threshold. Thus, at least in some fiber types, it may be possible to block nociceptor signaling and therefore pain, with manipulations such as the local administration of potassium (K+) channels openers80,81 that decrease membrane resistance and therefore spread the generator potential.
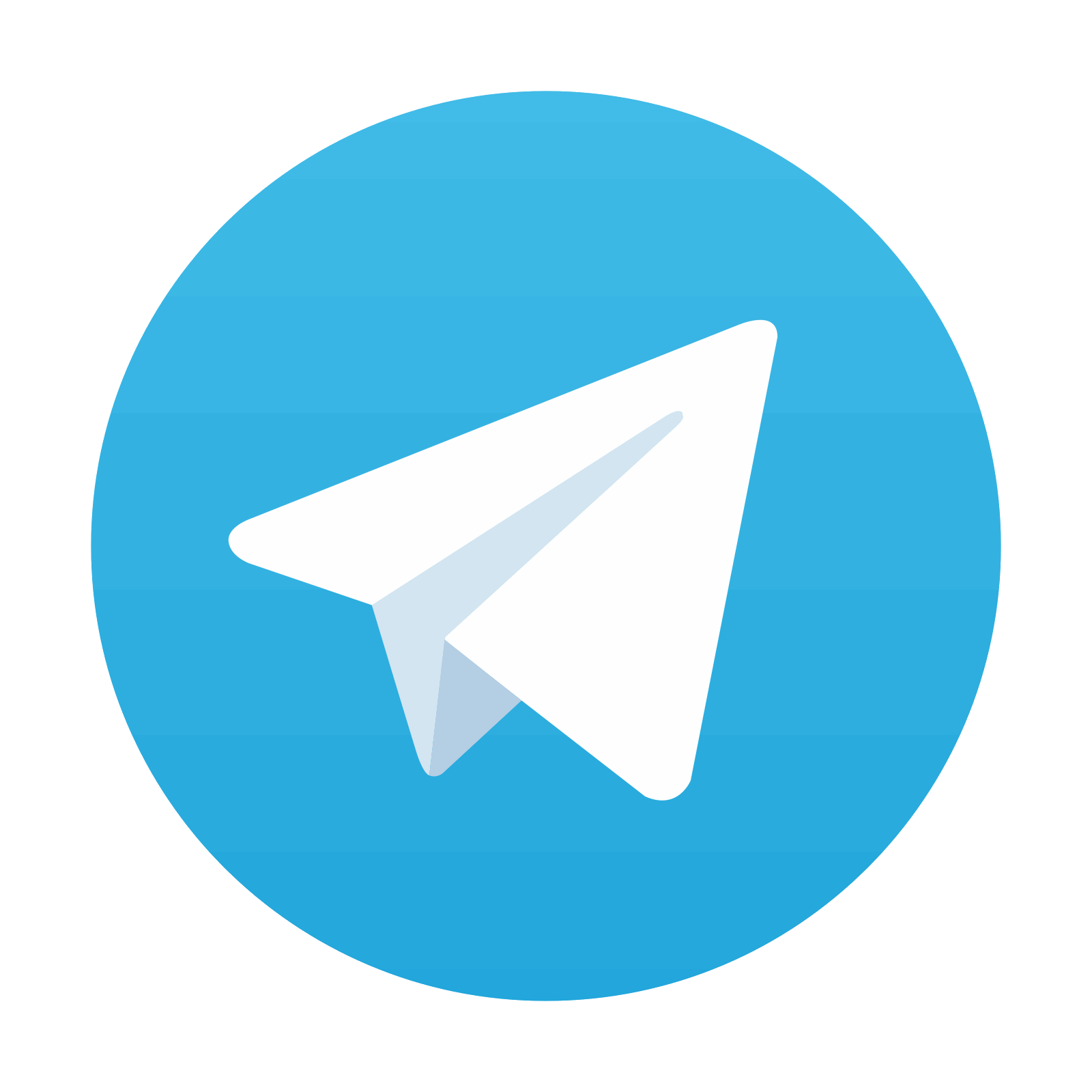
Stay updated, free articles. Join our Telegram channel

Full access? Get Clinical Tree
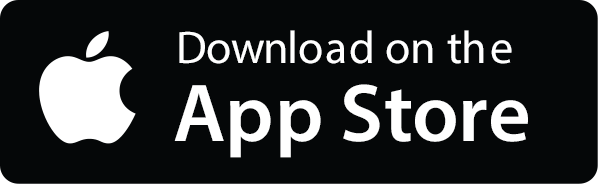
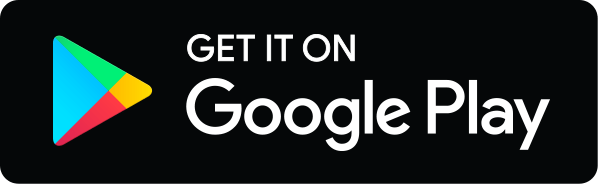