KEYWORDS
alarms and safety features
operating rooms
electrical grounding
isolation transformers
basic electronics
macro and micro current hazards
As we all know, electrical equipment is a big part of our lives. At home, out and about, in hospitals and the operating room (OR), electricity is flowing and powering our lives. The OR is a special area with special electrical requirements and unique dangers that we will explore. Naturally, part of that discussion involves some basic physics about electricity and discussion about circuitry. Even as we go through the definitions, keep in mind why the discussion about electricity is important. Besides the fact that electricity powers most of the equipment in an OR, electricity can be a major source of injury to patients, staff, and us by fire or by shock (Figure 21-1).
BASICS
Let’s start off with a classic equation of electricity, Ohm’s law, which is V = I × R. V is the force or voltage difference in volts, I is the current in amperes, and R is the resistance in ohms. If we rearrange the symbols to I = E/R, the flow, or current in amperes, is equal to the voltage difference divided by the resistance. So, the higher the voltage difference for a given resistance, the higher the flow of current is and vice versa. Following along in the equation, the higher the resistance for a given voltage, the lower the flow will be, and again, the reverse is also true. Another way to think of is to compare this to water behind a dam. As long as the dam is intact, there is no flow across the dam, but the water pressure behind the dam is still there. And the more water the dam is holding back, the higher the water pressure is behind the dam, and the more potential flow there would be if the dam were to fail. That is like the voltage in an electrical circuit. If there is a hole in the dam, then there will be flow, and that is similar to the amperage in the circuit (electrical current is electrons in motion). If you make a hole in a dam that has very little water behind it, the flow through the hole in the dam won’t be as great compared with a dam that has a lot of water behind it. This is the same as in an electrical circuit, where the higher the voltage or potential difference is, the more current will flow for a given resistance. This example also holds up for resistance. If you make a huge hole in a dam, the resistance to water flow through the dam will be less than if the hole is really small so there would be more flow through the dam with the big hole. It’s the same for electricity. The lower the resistance, the more flow there is for a given voltage.
There are only a few more terms we need to have clear in our minds. Wattage is electrical power, and its formula is P = V × I. Because V is I × R, wattage = I2 × R. The amount of work done by the electrical power is coupled with time. So a watt-second is called 1 joule. For the outside electrical wires that bring electricity to your house, the expression is in kilowatt-hours to accommodate higher amounts of energy. Impedance is applicable to AC circuits and is what opposes the flow of current in an AC circuit. Insulators have a high impedance, and conductors have a low impedance. Capacitance is the ability to store charge within a circuit. In all equipment, there is at least some stray capacitance, which means the equipment is able to hold a charge because it is made of conductors and insulators, not just because capacitors were built into its circuitry. Stray capacitance in electrical devices in the OR could cause a problem for OR personnel and especially patients with cardiac devices such as defibrillators. More on stray capacitance later.
So now we need to spend a little time talking about circuits. In its simplest form, an electrical circuit is a loop formed by wire that has a voltage source attached to it, like a battery. There is no flow of electricity if there is not a complete circuit leading to and from the voltage source. There is at least one other thing added to the circuit, and that something is what the electricity is powering, such as a light bulb, computer, or electrical appliance. The battery provides the voltage difference so that there is flow of electrons through the circuit, going to the appliance and returning to the battery. The higher the voltage is in the battery, the higher the flow will be for a given resistance (like the water behind the dam example we used earlier). Just a quick note about AC and DC circuits. There is a rich history in the United States of alternating current (AC) versus direct (DC) transmission on larger scales with AC eventually winning for reasons that aren’t important for this discussion. What is important is that AC current is usually how electricity travels over wires in the United States, and AC current is also what is on both sides of the isolation transformer from the OR side. What is important about DC circuits is that is what is usually inside many of the everyday devices we use such as computers.
Let’s talk a little bit about electrical ground or grounding. We have all heard about grounding, and it is important for us to have a basic understanding. If you think back to our discussion of a circuit, recall that a circuit is really just a loop for electrons or current to flow through. So there is a hot wire that brings the electricity to something and a neutral wire to bring the current back to its source, thus completing our loop or circuit. If the hot wire gets disrupted, there is no flow unless the current somehow finds a connection to the neutral wire or ground. But what happens if the neutral wire gets disrupted for some reason? Then the hot wire could bring the current to an appliance, casing, or outlet and charge it, and when you touch it, you could get shocked. There is a third wire, the grounding wire, that would take that stray current and send it to the earth ground, where it won’t hurt anybody. In this way, if the neutral wire is disrupted, the current has somewhere to go because current is lazy and will follow the path of least resistance, and the ground wire is a very low-resistance place for it to go. So grounding helps prevent charge from getting stored up on appliances or other things. We will talk about implications for grounding in the OR in just a little bit.
RISK OF SHOCK
Before we head to the OR, let’s spend a little time on electrical shock. So how do our patients, and we, get shocked? First of all, shock is electrical current applied to the body. How relevant that shock is to the patient or to us is dependent on several variables. First, where is the exposure? If it is to the skin, it is called macroshock, and if it goes straight to the heart, it is called a microshock. Microshock could occur if there was an exposed pacemaker lead, for example. As you can imagine, it usually takes a lot more electricity to hurt someone in a macroshock situation than it does in a microshock situation. For instance, the human threshold for perception of electric current on dry skin is about 1 milliamp, which, applied to skin, is noticeable but not dangerous. If that current were applied to a wire connected directly to the heart, there is a very high chance it would cause ventricular fibrillation (Table 21-1).
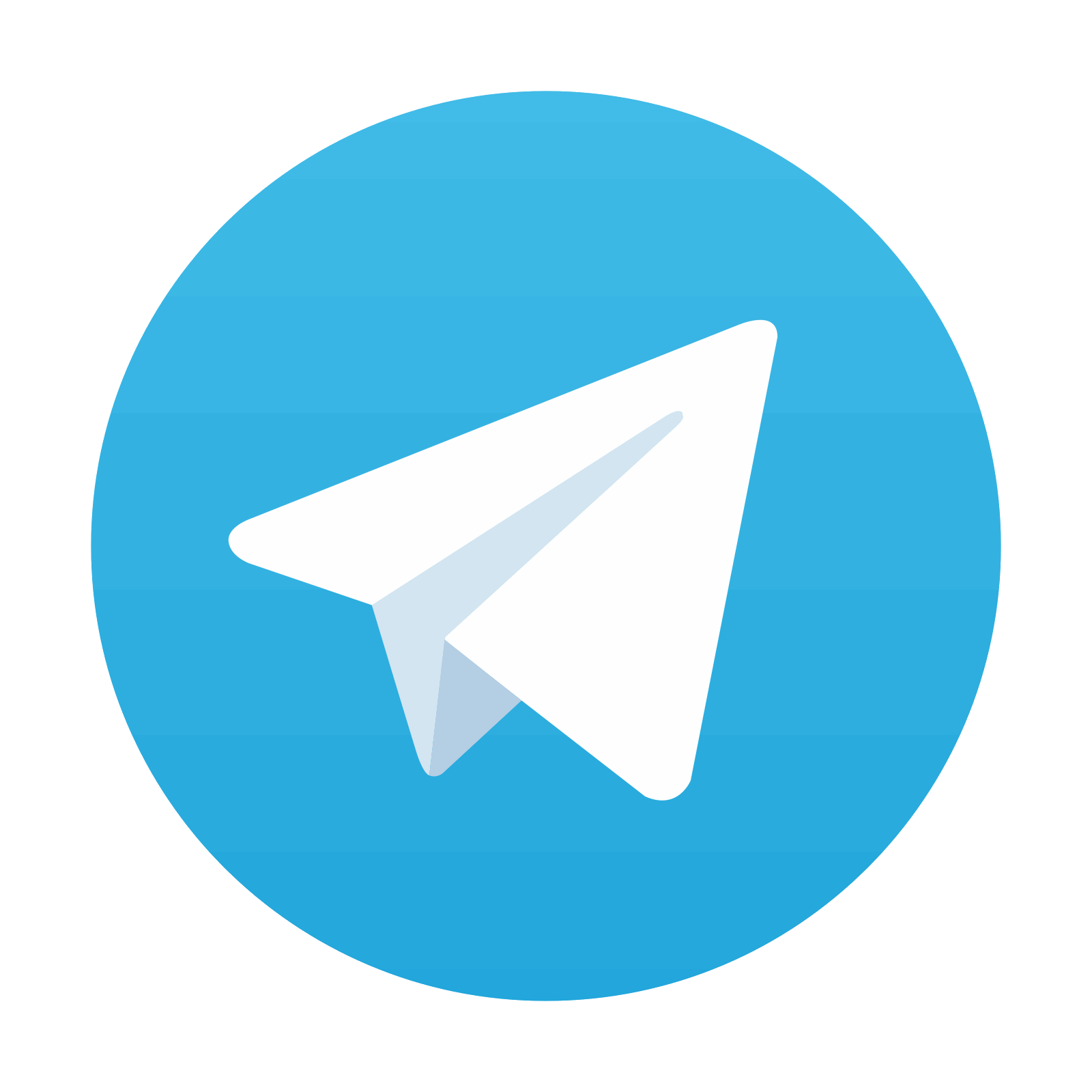
Stay updated, free articles. Join our Telegram channel

Full access? Get Clinical Tree
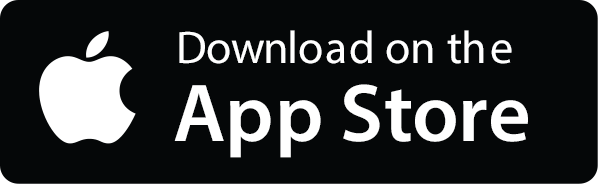
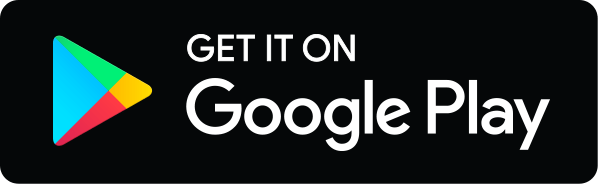