The electrical equipment used in the operating theatre and intensive care unit is designed to improve patient care and safety. At the same time, however, there is the potential of exposing both the patient and staff to an increased risk of electric shock. It is essential for the anaesthetist to have a thorough understanding of the basic principles of electricity, even though these devices include specific safety features.
In the UK, mains power source of electricity is supplied as an alternating current with a frequency of 50 Hz. The current travels from the power station to the substation where it is converted to main power voltage by a transformer. From the substation, the current travels in two conductors, the live and neutral wires. The live wire is at a potential of 240 V (or more accurately 240 RMS [root mean square]). The neutral is connected to the earth at the substation so keeping its potential approximately the same as earth. The live wire carries the potential to the equipment whereas the neutral wire returns the current back to the source, so completing the circuit.
Principles of electricity ( Fig. 15.1 )
Electric current (I)
An electric current is the flow of electrons through a conductor past a given point per unit of time, propelled by the driving force, i.e. the voltage (potential difference). The current is measured in amperes (A). One ampere represents a flow of 6.24 × 10 18 electrons (one coulomb of charge) past a specific point in 1 second.
- 1.
Direct current (DC): the current flows in one direction (e.g. flow from a battery).
- 2.
Alternating current (AC): the flow of electrons reverses direction at regular intervals (e.g. main power supply); in the UK, the frequency of AC is 50 cycles per second (Hz).

Potential difference or voltage (V)
It is the electrical force that drives the electric current. When a current of 1 A is carried along a conductor, such that 1 watt (W) of power is dissipated between two points, the potential difference between those points is 1 volt (V).
Electrical resistance (R)
Electrical resistance is the resistance along a conductor to the flow of electrical current. It is not dependent on the frequency of the current. Electrical resistance is measured in ohms (Ω).
Impedance (Z)
Impedance is the sum of the forces that oppose the movement of electrons in an AC circuit. The unit for impedance is the ohm (Ω). The term impedance covers resistors, capacitors and inductors and is dependent on the frequency of the current. Substances with high impedance are known as insulators. Substances with low impedance are known as conductors. The impedance through capacitors and inductors is related to the frequency (Hz) at which AC reverses direction. Such an impedance, i.e. frequency related, is known as reactance (X).
- 1.
Capacitor: impedance ∝ 1/frequency.
- 2.
Inductor: impedance ∝ frequency.
Ohm’s law
Electric potential (volts) = current (amperes) × resistance (ohms) [E = I × R]
Capacitance
Capacitance is a measure of the ability of a conductor or system to store an electrical charge. A capacitor consists of two parallel conducting plates separated by an insulator (dielectric). The unit for capacitance is the farad.
With AC, the plates change polarity at the same current frequency (e.g. 50/s). This will cause the electrons to move back and forth between the plates so allowing the current to flow.
The impedance of a capacitor = distance between the plates/current frequency × plate area.
Inductance
Inductance occurs when electrons flow in a wire resulting in a magnetic field being induced around the wire. If the wire is coiled repeatedly around an iron core, as in a transformer, the magnetic field can be very powerful.
Identification of medical electrical equipment
A single-fault condition is a condition when a single means for protection against hazard in equipment is defective or a single external abnormal condition is present, e.g. short circuit between the live parts and the applied part.
The following classes of equipment describe the method used to protect against electrocution according to an International Standard (IEC 60601).
Class I equipment
This type of equipment offers basic protection whereby the live, neutral and earth wires do not come into contact with each other. There is a secondary protection whereby parts that can be touched by the user, such as the metal case, are insulated from the live electricity and connected to an earth wire via the plug to the main power supply. Fuses are positioned on the live and neutral supply in the equipment. In addition, in the UK, a third fuse is positioned on the live wire in the main power source plug. This fuse melts and disconnects the electrical circuit in the event of a fault, protecting the user from electrical shock. The fault can be due to deteriorating insulation or a short circuit, making the metal case ‘live’. Current will pass to earth causing the fuse to blow (this current is called ‘leakage currenť). Some tiny non-fault leakage currents are always present as insulation is never 100% perfect. A faultless earth connection is required for this protection to function.
Class II equipment
This type of equipment, also called double-insulated equipment, has double or reinforced insulation protecting the accessible parts. There is no need to earth this type of equipment. The power cable has only ‘live’ and ‘neutral’ conductors with only one fuse.
Class III equipment
This type of equipment does not need electrical supply exceeding 24 V AC or 50 V DC. The voltage used is called safety extra low voltage (SELV). Although this does not cause an electrical shock, there is still a risk of a microshock. This equipment may contain an internal power source or be connected to the main power supply by an adaptor or a SELV transformer. The power input port is designed to prevent accidental connection with another cable.
The following types of equipment define the degree of protection according to the maximum permissible leakage current.
Type B equipment
This may be class I, II or III main source-powered equipment or equipment with an internal power source. This equipment is designed to have low leakage currents, even in fault conditions, such as 0.5 mA for class I and 0.1 mA for class II. It may be connected to the patient externally or internally but is not considered safe for direct connection to the heart.
The equipment may be provided with defibrillator protection.
Type BF equipment
This is similar to type B equipment, but the part applied to the patient is isolated from all other parts of the equipment using an isolated (or floating) circuit. This isolation means that allowable leakage current under single fault conditions is not exceeded even when 1.1 times the rated main power source voltage is applied between the attachment to the patient and the earth. The maximum AC leakage current is 0.1 mA under normal conditions and under a single fault condition is 0.5 mA. It is safer than type B but still not safe enough for direct connection to the heart.
The equipment may be provided with defibrillator protection.
Type CF equipment
This can be class I or II equipment powered by mains power source with an internal electrical power source, but considered safe for direct connection to the heart. Isolated circuits are used. There is no risk of electrocution by leakage currents (allows 0.05 mA per electrode for class I and 0.01 mA per electrode for class II). This provides a high degree of protection against electrical shock. This is used in electrocardiogram (ECG) leads, pressure transducers and thermodilution computers.
The equipment may be provided with defibrillator protection.
Attention!
The user must consult the accompanying documents for any equipment. A black triangle on a yellow background with an exclamation mark means that there is no standardized symbol for the hazard:
High voltage and risk of electrocution!
Protective earth
The equipment itself has its own earth connection via the green-and-yellow lead in the conventional three-pin plug. The earth lead is connected to the external case of the equipment so reducing it to zero potential. Although this provides some protection, it does not guarantee it.
Functional earth
This is part of the main circuit. The current is returned via the neutral wire back to the substation and so to earth. In effect, all conventional electrical circuits have functional earth. It is necessary for proper functioning of electrical equipment and is not a safety feature. On older equipment, the same symbol may have been used to denote protective earth.
Additional protective earth
This equipment carries an additional protective earth. This protects against electric shock in cases of a single fault condition.
Equipotentiality
This is used to ensure that all metalwork is normally at or near zero voltage. Therefore, under fault conditions, all the metalwork will increase to the same potential. Simultaneous contact between two such metal appliances would not cause a flow of current because they are both at the same potential, therefore no shock results. This provides some protection against electric shock by joining together all the metal appliances and connecting to earth.
Drip proof, splash proof, water tight
Depending on the nature and use of the equipment, some are drip proof, splash proof or water proof.
Anaesthetic-proof equipment
Anaesthetic-proof (AP) equipment standards are based on the ignition energy required to ignite the most flammable mixture of ether and air. They can be used within 5–25 cm of gas escaping from a breathing system. The temperature should not exceed 200°C. It is a less stringent requirement.
Anaesthetic-proof equipment category G
Anaesthetic-proof equipment category G (APG) standards are based on the ignition energy required to ignite the most flammable mixture of ether and oxygen. Can be used within 5 cm of gas escaping from a breathing system. The temperature should not exceed 90°C. This is a more stringent requirement as the energy level should be less than 1 mJ.
CE
This is one of the most important symbols. It means conformity has been met according to the Council of Europe Directive 93/42/EEC concerning medical devices.
Isolated or floating circuit
This is a safety feature whereby current is not allowed to flow between the electrical source and earth. These circuits are used to isolate individual equipment. An isolating transformer is used with two coils insulated from each other. The main power source circuit is earthed whereas the patienťs circuit is not earthed, so floating. As current flows through the main power source coil (producing an electromagnetic field), a current is induced in the patienťs coil. To complete the patienťs circuit, the wires A and B should be connected. Contact with wire A or B alone will not complete a circuit, even if the subject is earthed.
Current-operated earth leakage circuit breakers (COELCB)
These safety features are also known as an earth trip or residual circuit breakers . They consist of a transformer with a core that has an equal number of windings of a live and neutral wire around it. These are connected via a third winding to the coil of a relay that operates the circuit breaker. Under normal conditions, the magnetic fluxes cancel themselves out, as the current in the live and neutral wires is the same. In the case of a fault (e.g. excessive leakage current), the current in the live and neutral wires will be different so resulting in a magnetic field. This induces a current in the third winding causing the relay to break circuit. The COELCB are designed to be very sensitive. A very small current is needed to trip the COELCB (e.g. 30 mA) for a very short period of time reducing the risk of electrocution.
Maintenance of equipment
Two factors should be checked during the maintenance of equipment (details of the tests are beyond the scope of this book):
- 1.
Earth leakage : the maximum current allowed is less than 0.5 mA. Devices that are connected directly to the patienťs heart should have a leakage current of less than 10 mA.
- 2.
Earth continuity : the maximum resistance allowed is less than 0.1 Ω.
Hazards of electrical shock
An electric shock can occur whenever an individual becomes part of the electric circuit. The person has to be in contact with the circuit at two points with a potential difference between them for the electrons to flow. This can happen either with a faulty high-leakage current or by a direct connection to the main power source. Main power source frequency is very dangerous as it can cause muscle spasm or tetany. As the frequency increases, the stimulation effect decreases but with an increase in heating effect. With a frequency of over 100 kHz, heating is the only effect. Electric shock can happen with both AC and DC. The DC required to cause ventricular fibrillation is very much higher than the AC.
If a connection is made between the live wire and earth, electricity will flow through that connection to earth. This connection can be a patient or member of staff. Main power source supplies are maintained at a constant voltage (240 V in the UK). According to Ohm’s law, current flow is ∝ 1/impedance. A high impedance will reduce the current flow and vice versa. The main impedance is the skin resistance which can vary from a few hundred thousand ohms to one million ohms. Skin impedance can be reduced in inflamed areas or when skin is covered with sweat.
Current density is the amount of current per unit area of tissues. In the body, the current diffusion tends to be in all directions. The larger the current or the smaller the area over which it is applied, the higher the current density.
Regarding the heart, a current of 100 mA (100 000 μA) is required to cause ventricular fibrillation when the current is applied to the surface of the body. However, only 0.05–0.1 mA (50–100 μA) is required to cause ventricular fibrillation when the current is applied directly to the myocardium. This is known as microshock .
Methods to reduce the risk of electrocution
- •
General measures: adequate maintenance and testing of electrical equipment at regular intervals, wearing antistatic shoes and having antistatic flooring in theatres.
- •
Ensuring that the patient is not in contact with earthed objects.
- •
Equipment design: all medical equipment used in theatres must comply with British Standard 5724 and International Electro-technical Committee (IEC) 60601–1 describing the various methods used for protection and the degree of protection (see above).
- •
Equipotentiality (see above).
- •
Isolated circuits (see above).
- •
Circuit breakers (COELCB) (see above).
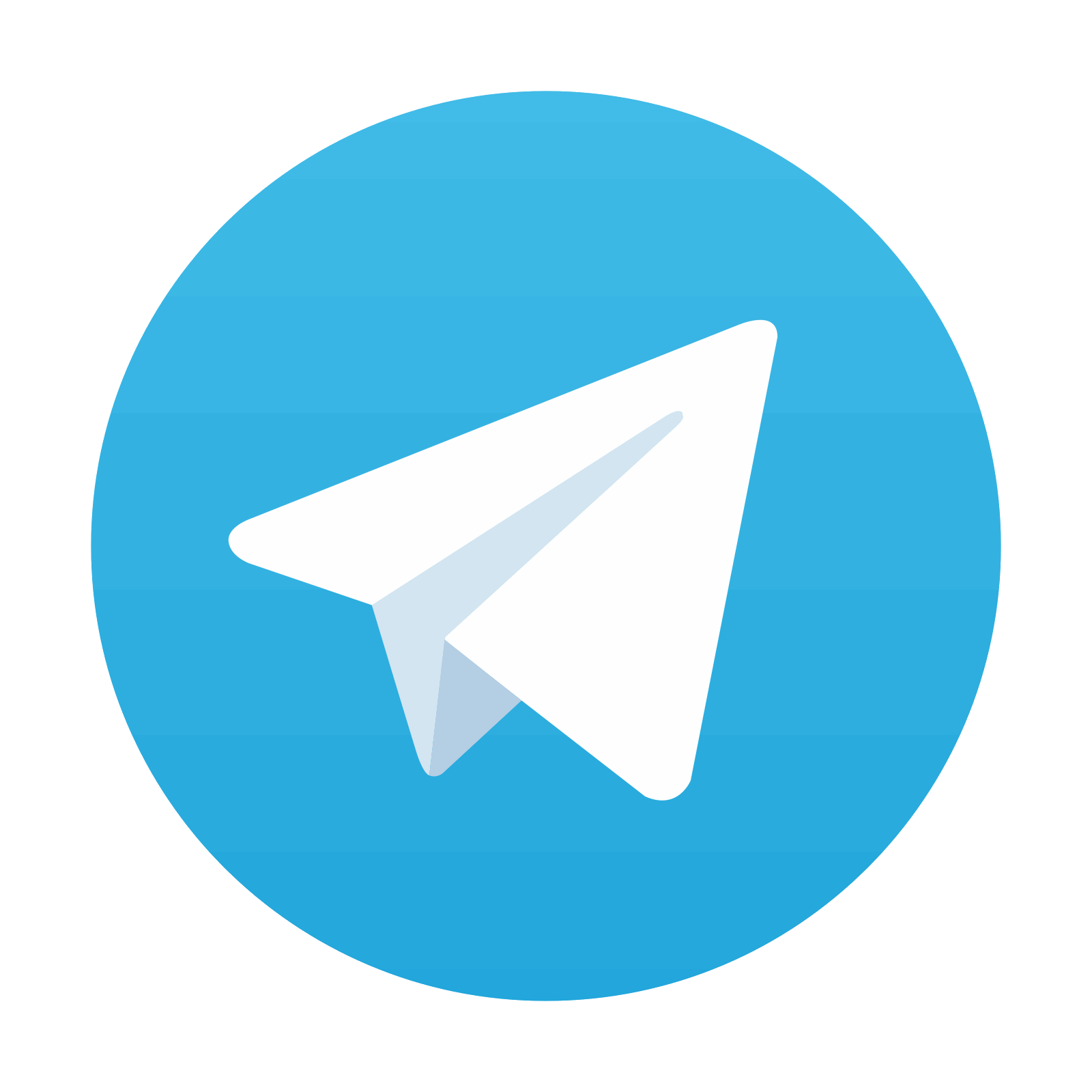
Stay updated, free articles. Join our Telegram channel

Full access? Get Clinical Tree
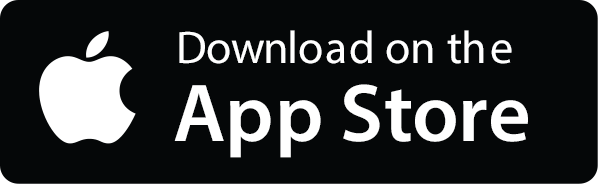
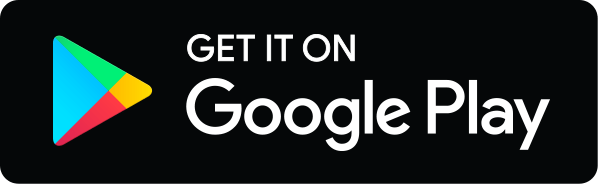
