INTRODUCTION
Electrical injuries are divided into high-voltage injuries (≥1000 V), low-voltage injuries (<1000 V), and electric arc flash burns, which by definition do not result in passage of current through the tissues. Lightning injury is an extreme and unique form of electrical injury. This chapter also discusses injuries caused by electronic control devices, such as the Taser®. Burns from electrical accidents can result from heating due to electric current flow through tissues, explosions, and burning of flammable liquids, clothes, and other objects. Burns are discussed in the chapter 216, “Thermal Burns.”
EPIDEMIOLOGY
Approximately 6500 electrical injuries occur per year in the United States, accounting for 4% of total burns. Of these, the majority are work related (61%), mostly industrial injuries. The overall complication rate is 10.6%, with the fewest complications among children age 1 to 5 years (2%).1 The most common high-voltage injuries in the United States are also work related and include arc burns in electricians and high-voltage injuries in power line workers.2 The Electrical Safety Foundation International estimates that contact with electric current caused nearly 1800 workplace fatalities between 2003 and 2010.3 High-voltage power line injuries are particularly disabling because they often lead to deep-muscle necrosis and the need for fasciotomy and amputation.2
BASICS OF CURRENT FLOW
Electric current is the movement of electrical charges. Table 218-1 lists a few key terms related to electricity.
Term | Unit of Measure |
---|---|
Electric current Movement of electrical charges | Amperes |
Current flow Driven by voltage or electrical potential difference | Volts |
Resistance Hindrance to flow of current | Ohms |
Ohm’s law (current = voltage/resistance) The current is proportional to voltage. The current is inversely proportional to resistance. | — |
Current flow is measured in amperes. Current flow is driven by an electrical potential difference, which is measured in volts. Intervening material between two or more contact points resists electric current flow; this resistance is measured in ohms. Ohm’s law describes the relationship between current (I), voltage (V), and resistance (R) and states that the current through a conductor between two points is directly proportional to the potential difference or voltage drop across the two points and inversely proportional to the resistance between them. For example, a person who grasps a grounded pipe in one hand and a metal cable connected to a 120-V source in the other hand will experience a current flow through the body, the magnitude of which varies inversely with the resistance of the circuit. If the total resistance from the power source, through the person, and to ground is estimated to be 1000 Ω, the current would be I = (120 V)/(1000 Ω) = 0.120 A = 120 mA.
Conductors are materials that allow electric current to flow easily. Insulators are materials that do not allow electric current flow. Most biologic materials conduct electricity to some extent. Tissues with high fluid and electrolyte content conduct electricity better than tissues with less fluid and electrolyte content. Bone is the biologic tissue with the greatest resistance to electric current, whereas nerves and vascular structures have low resistance. Dry skin has high resistance, but sweaty or wet skin has much less resistance.
Many of the physiologic effects of electric shock are related to the amount, duration, and type of current (alternating current [AC] or direct current [DC]) and the path of current flow (Table 218-2). DC current flows in a constant direction, whereas AC current alternates direction in a cyclical fashion. Standard household electricity is AC. Electricity in batteries and lightning is DC. Low-frequency (50- to 60-Hz) AC can be more dangerous than similar levels of DC because the alternating current fluctuations can result in ventricular fibrillation. The identification of electric shock as due to AC or DC is also important to reconstruct the mechanism of injury. AC current can produce muscular tetany, during which the victim cannot let go of the electrical source. Both AC and DC current can hurl the victim away from the current source, which results in severe blunt force injury.
Effect | Current Path | Minimum Current: 60 Hz AC (mA)* |
---|---|---|
Tingling sensation, minimal perception | Through intact skin | 0.5–2.0 |
Pain threshold | Through intact skin | 1–4 |
Inability to let go: tetanic contractions of hand and forearm tighten grasp, decreasing skin resistance | From hand through forearm muscles into trunk | 6–22 |
Respiratory arrest: can be fatal if prolonged | Through chest | 18–30 |
Ventricular fibrillation | Through chest | 70–4000 |
Ventricular standstill (asystole): similar to defibrillation; if current stops, sinus rhythm may resume | Through chest | >2000 |
For current to flow through an individual, a complete circuit must be created from one terminal of a voltage source to a contact area on the body, through the subject, and then from another contact on the person to the other terminal of the voltage source (or to a ground that is connected to the voltage source). Current flows through a person from one contact area to another along multiple, somewhat parallel paths. For example, electrical power source contacts just on the left hand and left leg would result in current flow through those limbs and the trunk, including the heart, muscles of respiration, and other tissues in the trunk. Current would not flow through the other limbs or head, because they are not in an electrically conductive path between the two power source contacts. The pattern of current flow through the body, if known, can be used to raise or lower suspicion of injury to certain body parts; for instance, hand-to-hand flow involves crossing the thorax and heart, raising the suspicion for cardiac involvement.
An exception to the requirement that a circuit be present occurs with electrostatic discharge. Electrostatic discharge is the transfer of excess charge to or from a person or object to another object and results when objects at different potentials come into direct contact with each other. An example is static electricity, which can cause pain and reflex movements.
MECHANISMS OF ELECTRICAL INJURY
The risk for serious and fatal electrical injury increases with voltage, especially >600 V (Table 218-2). High voltage is usually defined as >1000 V. Power lines in U.S. residential areas typically carry 7620 V AC. This is stepped down by transformers to 240 V AC before entering most residential and nonindustrial buildings. In the United States, home outlets are 120 V, and in Europe and Australia, they are 240 V. Urban subway electrical third rails may be 600 V or more AC or DC. High-voltage injuries are more often associated with severe musculoskeletal, visceral, and nervous system injury than are low-voltage injuries.
Electricity-induced injuries can occur via several mechanisms: (1) direct tissue damage from the electrical energy, (2) tissue damage from thermal energy, and (3) mechanical injury from trauma induced by a fall or muscle contraction.
Electrical burns are severe when high voltages are involved, because only a fraction of a second of current flow is necessary for severe damage to occur.4 Burns are less common with low-voltage injuries, because low-voltage contact produces little heat energy in the skin and other tissues.5
Electricity arcing from one conductor to another may radiate enough heat to burn and even kill persons 10 or more feet from the arc. There can also be a blast force that throws the person. Arcs that do contact a person directly will have heating due to current flow through the body, in addition to the flash burn and mechanical blast forces. Serious burns often result.6 The voltages that create an electric arc are usually in the thousands of volts. Temperatures as high as 20,000°C (35,000°F) are created. Serious, and sometimes fatal, burns may result from heat radiated by the arc and clothing ignited by the arc.
Electric current can induce sustained muscular contraction, or tetany. The overall effect varies according to type (AC or DC), frequency, voltage, and extent of contact.4,7 For example, AC current flowing through the forearm can cause flexor tetany of the fingers and forearm that overpowers actions of the extensor muscles. Forceful muscle contractions can cause fractures and joint dislocations, especially around the shoulders.8 If the hand and fingers are properly positioned, the hand will grasp the conductor tightly, leading to prolonged, low-resistance contact with the power source because the person cannot let go. This allows current to flow for many seconds or minutes.
The increased duration of contact and the decreased contact resistance caused by a tight sustained grasp greatly increase the heat-related damage to deep tissues. Heating due to electric current builds over time. Because heating is directly proportional to the duration of current flow and proportional to the square of the current amplitude (until burning and other tissue changes occur), a person who is unable to let go of a conductor for 30 seconds receives about 90 times as much tissue heating as a person who recoils from the voltage source in one third of a second. To make injury still more severe, a tight grasp on a conductor decreases contact resistance, which leads to an increase in current flow. Thus, heat-related injury to deep tissues is often more than a hundred times worse when there is a history of the patient’s being unable to let go of the conductor.
Current flow through the trunk and legs may cause brief, but strong, opisthotonic (arching) posturing and leg movements. The person appears to be thrust from the voltage source due to these muscle contractions and may sustain mechanical trauma in addition to electrical injury. If a person does manage to hold on to a high-voltage source, severe tissue damage occurs.
CLINICAL FEATURES OF ELECTRICAL INJURY
Electric current can induce immediate cardiac dysrhythmias, respiratory arrest, and seizures. Current that traverses the chest vertically (hand to foot or head to toe) or horizontally (hand to hand) can produce arrhythmias and respiratory arrest.
Fatalities due to asystole or ventricular fibrillation usually occur prior to arrival in the ED.5 Asymptomatic patients with normal ECGs on arrival to the hospital do not develop later dysrhythmias after low-voltage (<1000 V) injuries.9,10,11,12,13 However, nerve and deep-muscle injuries are relatively common following contact with voltages >400 V. Therefore, carefully examine for peripheral nervous system and CNS function and burns, and check for elevated serum creatine phosphokinase.
Neurologic impairment is common in electrical injuries, occurring in approximately 50% of patients with high-voltage injuries.2 Nerve tissue has the lowest resistance in the body, encouraging electrical passage through these tissues and causing associated damage. Given the multisystem dysfunction present in many electrical injury patients, document a neurologic exam, if possible, before intubation and sedation.
Electrocution can result in a broad range of CNS dysfunction. Transient loss of consciousness is common and may be followed by seizures. Victims may be confused and agitated or deeply comatose and require airway protection. Patients may also demonstrate focal neurologic deficits such as quadriplegia, hemiplegia, aphasia, or visual disturbances. Obtain head and cervical spine CT to rule out traumatic etiologies for these deficits; MRI may be necessary for purely electrical damage. Electrical injury can cause blindness14 due to occipital lobe injury as well as direct injury to the optic nerve.15,16 Neurologic deficits may be transient, but survivors may have persistent difficulties with attention, working memory, and learning.17
Spinal cord injury can occur in up to 8% of high-voltage electrical injuries.18 Spinal cord injuries can be the result of compressive vertebral fractures, sometimes at multiple levels.19 However, damage can also occur due to purely electrical damage without fractures, through direct cellular damage as well as vascular injury.20 With electrical trauma, initial spinal cord MRI results do not necessarily correlate with prognosis. MRI findings may be normal in electrical trauma patients with permanent spinal cord injury,21,22,23 although newer MRI imaging protocols may detect abnormalities silent on standard MRI.24 With mechanical trauma, emergency MRI after spinal cord injury provides accurate prognostic information regarding neurologic function.
Neurologic deterioration can occur days to months after the initial injury25,26,27 and generally has incomplete resolution. There is a motor predominance in deficit in most of these cases. Delayed onset of spinal cord dysfunction may be due to progressive vascular injury (especially to the spinal artery branch supplying the anterior horn cells) or delayed cell membrane damage via the cumulative effect of free radicals (electroporation), leading to progressive demyelination.21,28 The clinical features may take the form of transverse myelitis, amyotrophic lateral sclerosis, or a Guillain-Barré–like illness.29
Peripheral nerve injuries often involve the hands after the individual touches a power source. Paresthesias may be immediate and transient or delayed in onset, appearing up to 2 years after injury.2,30 Extensive peripheral nerve damage may occur with minimal thermal injury. Electrical contact with the palm produces median or ulnar neuropathy more often than radial nerve injury.31 Brachial plexus lesions have also been reported. Persistent symptoms related to peripheral nerve damage can occur despite normal results on nerve conduction studies.32
Cutaneous burns are often seen at the electrical contact areas (often referred to as entry and exit wounds in the case of DC current, or contact wounds in the case of AC current). Many seriously injured patients have burns on either the arm or skull, paired with burns on the feet. Burns are typically painless, gray to yellow, depressed areas. Most patients with burns from electrical injury need admission and care by a burn specialist. See chapter 216 for detailed discussion of management of cutaneous burns.
Fractures may be caused by tetanic muscle contractions or associated falls. Fractures may be missed on initial assessment due to altered mental status and the overall severity of systemic illness. A comprehensive tertiary survey should be performed when clinically feasible to detect orthopedic injuries. Although fractures are more likely to result from high-voltage injury, fractures of the wrist, forearm, humerus, femoral necks, shoulders, and scapulae have been reported from exposure to household voltages (120 to 220 V AC) without associated trauma.7,33,34 Posterior shoulder dislocations are commonly seen with electrical injury.
Vascular and muscle injuries occur most commonly in the setting of high-voltage injury, such as power line contact. Electric current passing along peripheral arteries may cause early spasm and persistent deficits in endothelial and smooth muscle function,35 as well as subsequent thrombosis, stenosis, or aneurysm formation. Because of concomitant vascular and muscular destruction, patients with high-voltage shocks are at significant risk for development of compartment syndrome, even if the contact (or arcing) lasted <1 second. Compartment syndrome has also been noted in patients with injuries from 120 V AC or higher who sustain contact for longer than a few seconds. Patients typically exhibit ongoing muscle pain with movement.6
Contact with >1000 V, prehospital cardiac arrest, crush injury, and full-thickness skin burns are associated with significant tissue damage requiring surgical intervention. Fasciotomy and amputation are frequent sequelae of high-voltage injuries, occurring in 29% and 41% of patients in one study.36 High-voltage electrical injury is associated with rapid loss of body fluids into the areas of tissue damage, requiring aggressive resuscitation.
Thermal injury or tissue necrosis from electric current can cause a variety of coagulation disorders. Low-grade disseminated intravascular coagulation may be a result of hypoxia, vascular stasis, rhabdomyolysis, and release of procoagulants from damaged tissue. Transient coagulopathies have also been reported with high-voltage injury, including acquired, transient factor X deficiency.37 Electrical injury may unmask underlying vascular injury and has been reported in association with ischemic stroke at low voltage.38
Electric arcs in the industrial environment or near a power line can produce a strong blast pressure, similar to those seen in other types of explosions.39 Cognitive complaints following blast injury may resemble those that result from moderate mechanical head trauma. Mechanisms of brain injury include mechanical trauma related to the blast and arterial air emboli associated with blast-related alveolar disruption (see chapter 7, “Bomb, Blast, and Crush Injuries”).
Chemical toxins such as ozone can be produced by coronas and arcs. Acute effects of ozone exposure include mucous membrane irritation, temporarily reduced pulmonary function, and pulmonary hemorrhage and edema. Fires and explosions associated with electric incidents may lead to inhalation of carbon monoxide and other toxic substances.
Electrical shock can cause a wide range of ocular trauma, most commonly to the cornea (epithelial erosion/defect, keratitis, scarring), as well as uveitis, retinal detachment, macular edema, optic nerve damage, and intraocular bleeding and thrombosis.15,40,41 Obtain a full ophthalmologic evaluation for any electric shock patient with an ocular complaint to document related injury. Cataract formation has been described weeks to years after electrical injury to head, neck, or upper chest.7 Cataracts have also occurred after electric arc or flash burns.
The auditory system may be damaged by current or by hemorrhage in the tympanic membrane, middle ear, cochlea, cochlear duct, and vestibular apparatus. Delayed complications include mastoiditis, sinus thrombosis, meningitis, and brain abscess. Hearing loss may be immediate or develop later as a result of complications. Hearing should be briefly checked in the ED, with follow-up formal testing arranged for any patient who appears to have a deficit.
Pain due to bowel perforation and intra-abdominal hemorrhage may be attributed to more obvious coexisting injuries.42 There are reports in the literature of lethal intra-abdominal injuries from electric current that were found only at autopsy. Ileus may develop in association with spinal cord injury.
SCENE AND PREHOSPITAL CARE
Table 218-3 outlines prehospital care of the patient with electrical injury.
Stay at least 10 m (32 ft) from downed power lines, jumping power lines, and support structures. Turn off the source of electricity prior to rescue, if possible. If electrical source cannot be quickly turned off, take precautions to prevent electrical injury to the rescuer. Wear gloves and shoes rated for the power line voltage. Initiate rescue breathing and resuscitation efforts while injured person is still on pole, if no contact with source of electricity is assured. Maintain spinal immobilization of injured person, if possible. |
Stay away from downed power lines. The scene of a high-voltage electric incident contains many hazards for rescue personnel. Power lines are almost never insulated, although a line may appear to be insulated because of atmospheric contaminants deposited on the line over time. Electrocution is possible when walking on ground near a downed power line because of voltage gradients in the ground. Stay at least 10 m (32 ft) from downed power lines. Even this distance is not without risk.
Reapplication of voltage to downed lines sometimes occurs as circuit breakers automatically reset, which makes the lines physically jump many feet with great force. This danger may be mitigated by placing a heavy object over the line, but this maneuver itself is fraught with risk.
Support structures may be electrically alive. Metal cables that support telephone and power poles are normally grounded, but may be energized if they are broken or disconnected from a ground attachment and contact is made with a nearby power line.
Victims still in contact with a source of voltage may transmit an electric current to would-be rescuers. It is best first to turn off the source of electricity if it can be done quickly. If this cannot be done quickly, precautions must be taken to prevent electrical injury to the rescuer. With voltages above about 600 V, dry wood and other materials may conduct significant amounts of electric current and therefore cannot be used to remove the person from a voltage source. A rescuer standing on the ground touching any part of a vehicle that is in contact with a power line is likely to be killed or seriously injured. Electric shock is not prevented in this situation by the rescuer’s wearing rubber gloves and boots, unless these are designed for the voltage present and have been recently tested for insulation integrity. Persons inside a vehicle in contact with a power line are likely to be killed as they step out of the vehicle and may also receive a shock if they touch objects at different potentials inside the vehicle.
Rescue breathing for line workers in respiratory arrest on utility power poles should be initiated by rescuers while the injured individual is still on the pole as long as the injured worker and rescuers are no longer in contact with the energized line. As soon as the patient is lowered to the ground, chest compressions can be done if there is cardiac and respiratory arrest.
Low-voltage AC can produce ventricular fibrillation by direct stimulation of the heart, or it can occur after several minutes of respiratory arrest resulting from paralysis of respiratory muscles (Table 218-2). High-voltage AC and DC are more likely to produce transient ventricular asystole. Asystole sometimes reverts spontaneously to normal sinus rhythm with pulses, but prolonged apnea may continue. Apnea with pulses
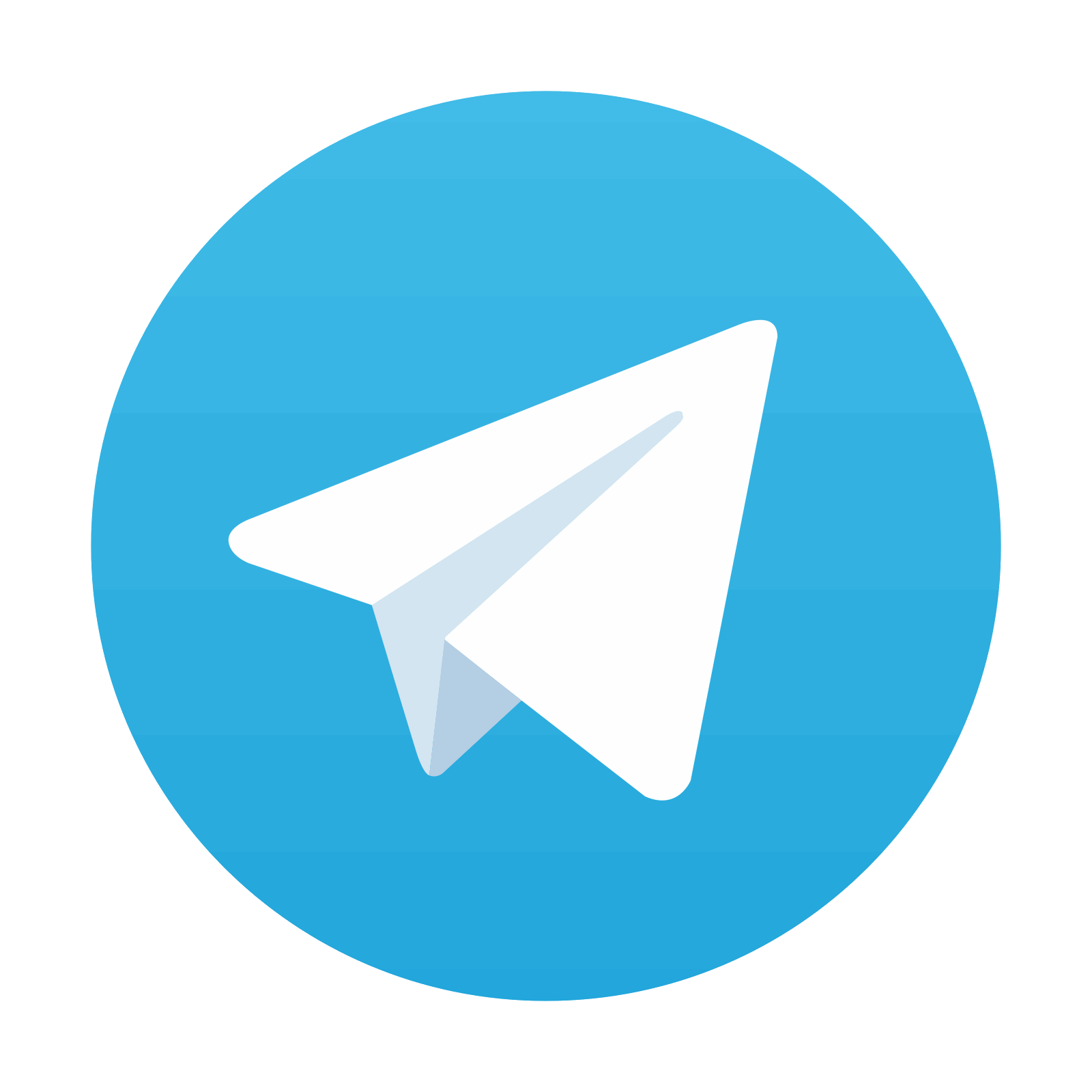
Stay updated, free articles. Join our Telegram channel

Full access? Get Clinical Tree
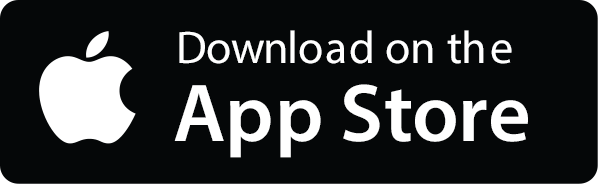
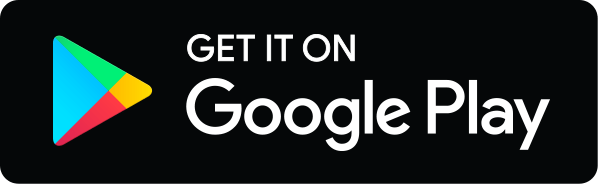
