Right ventricular (RV) dysfunction is common in critically ill patients.1–3 It is associated with multiple clinical scenarios frequently encountered by the intensivist, including acute cor pulmonale (ACP), acute RV dysfunction of sepsis, and acute RV infarction. In addition, the assessment of RV function is essential for the determination of a patient’s preload responsiveness. Echocardiography is the best available method to diagnose and monitor RV function at the bedside, as it provides the intensivist with a prompt, accurate, noninvasive, and serial method to monitor the function of the right heart and its response to different interventions. This chapter describes a variety of echocardiographic methods to assess RV function that are particularly relevant to critical care practice for both the basic and advanced critical care echocardiographer. While the assessment of RV function in the noncritically ill patient is beyond the purview of this chapter, the techniques described here are also applicable to the assessment of RV function in the ambulatory patient.
This chapter is designed to have utility for intensivists with basic critical care echocardiography (CCE) skills. In this case, the examination is limited to the standard twodimensional (2D) five view approach (see Chapter 6), which focuses on a qualitative visual estimate of RV size and septal dynamics. This approach is a key component of the rapid evaluation of hemodynamic failure.
In addition, this chapter reviews the evaluation of RV function using methods that are relevant only to the intensivist with skill at advanced critical care echocardiography. By definition, these include Doppler-based measurements that are not part of basic CCE. Given the time constraints of bedside scanning in a busy intensive care unit, the discussion will focus on a limited number of methods rather than listing all of the possible methods for evaluation of RV function that are available to the advanced level echocardiographer.
The RV comprises two anatomically and functionally distinct cavities separated by the crista supraventricularis: an inflow region (the sinus) and an outflow tract (the cone or infundibulum). The tricuspid valve (TV) and its apparatus plus heavily trabeculated myocardium form the sinus. Smooth myocardium and the pulmonic valve form the infundibulum. The sinus generates pressure during systole while the infundibulum modulates this pressure and prolongs its duration. RV contraction occurs serially in three different phases: (a) contraction of the sinus along its longitudinal axis, (b) radial contraction of the RV free wall toward the interventricular septum (IVS), and (c) torsion of the left ventricle (LV) (clockwise rotation of the LV base with counterclockwise rotation of apex) pulling the RV in similar manner. Overall, LV contraction contributes 25% of its own stroke work to the generation of RV stroke work via the IVS.4
The normal RV is less muscular than the LV and has a free wall thickness that measures 3–4 mm. As a consequence, it is easily affected by its surroundings and the effects of increased afterload. Unlike the LV, it is able to acutely dilate. Relative to the LV, the RV is a lower pressure chamber, with normal pressures of less than 25/8 mmHg with the patient in the resting state. When afterload increases acutely, the RV is unable to acutely generate higher pressures. However, when subjected to chronic loading conditions, the RV compensates with a hypertrophic response that is suggested by a thickened RV free wall on echocardiography. In this situation, the RV can generate up to systemic-level pressures, such as is seen with advanced pulmonary arterial hypertension.
ACP is defined as the clinical setting in which the RV experiences a sudden increase in afterload.5 This may occur in the context of previously normal RV function or in the RV that is already impaired. Sudden increases in RV afterload occur frequently in critically ill patients (Table 11-1). ACP is synonymous with acute right heart failure. It is characterized by the combination of systolic and diastolic overload, both of which have distinctive echocardiographic features.
Acute left heart failure (ischemic, myocardial, or valvular origin) |
Acute pulmonary embolism |
Acute respiratory distress syndrome (ARDS) |
Inappropriately adjusted ventilatory support |
Respiratory and metabolic acidosis3 |
Fat emboli |
Gas emboli |
Low Pao2 |
Dyskinesis is defined as a myocardial segment moving outward during systole. Septal dyskinesia is the echocardiographic hallmark of a sudden elevation of RV systolic afterload and occurs because of ventricular interdependence. When RV afterload is increased, its contraction is prolonged, requiring a longer time for completion than left ventricular systole. Because the RV is still contracting at the end of systole when the LV is beginning to relax, the right intraventricular pressure becomes transiently greater than the left intraventricular pressure, and the IVS is displaced in the direction of the LV cavity.6 When due to pulmonary vascular processes (e.g., acute respiratory distress syndrome [ARDS] and pulmonary embolism [PE]), septal dyskinesia develops rapidly in the course of disease.
Septal dyskinesia can be assessed qualitatively and quantitatively. The qualitative evaluation consists of observing for paradoxical septal motion by 2D echocardiography. With TTE, septal dyskinesia is well observed from the apical four-chamber and parasternal short-axis views, the latter at the midventricular level papillary muscle level (Figure 11-1 and Video 11-1). Identification of septal dyskinesia is part of the basic CCE examination. M-mode may be used for identification of abnormal septal motion (Figure 11-2).
Figure 11-1
This parasternal long-axis view shows a flattened septum. Review of Video 11-1 demonstrates the septal dyskinesia that is associated with this septal flattening.

Quantification of systolic RV overload can also be achieved by measurement of the systolic eccentricity index (EI). To do this, the short-axis view of the mid-LV (when both papillary muscles are displayed) is obtained with transthoracic (TTE) or transesophageal (TEE) echocardiography. At end systole, D1 is measured as the diameter that bisects the papillary muscles and D2 is the orthogonal diameter to D1. The systolic EI = D2/D1. A normal systolic EI is 1. Septal dyskinesia will result in an EI > 1 (Figure 11-3).7 An atrial septal defect can result in a falsely elevated EI.8 Therefore, a search for intracardiac shunting should always be considered when the EI is >1.
Systolic RV overload can also be indirectly identified by Doppler assessment of the right ventricular outflow tract (RVOT) or main pulmonary artery (MPA) with both TTE and TEE using pulsed-wave (PW) Doppler. The MPA is imaged in the parasternal short-axis view with TTE and in the great vessel view with TEE. The PW sample volume is placed in the MPA in order to obtain the systolic velocity envelope. The systolic velocity envelope is recorded allowing both qualitative and quantitative assessments of increased pulmonary vascular impedance (Table 11-2). These measurements have methodological problems that include the following:
Poor TTE image quality: This is problematic in the critically ill patient on ventilator support. The use of TEE obviates this problem.
Misalignment between the ultrasound beam and RVOT jet: Even with color Doppler guidance, the spectral Doppler signal may not reflect the spatial distribution of the pulmonary artery (PA) jet, which is higher along the inner edge of its natural curvature.
Misinterpretation of the spectral Doppler signal: Only the outer edge of the spectral envelope should be used.
Difficulty in measurement of the short time intervals being measured: Inter and intraobserver variability is a problem.
The time required to perform the measurements: The intensivist, having responsibility for image acquisition at the bedside, has limited time to perform a comprehensive examination. Time constraints are a reality in a busy intensive care unit. The intensivist needs to select a limited number of measurements that have utility in defining RV function, as it is unrealistic to perform all possible measurements.
Normal | RV Systolic Overload | |
Decreased stroke volume | 70–100 mL | <70 mL |
Decreased RVOT velocitytime integral (VTI) | 18 ± 3 cm | <15 cm |
Decreased acceleration time | ≥120 m/s | <80 m/s |
Decreased ejection time | 304 ± 23 m/s | <281 m/s |
Decreased acceleration time/ejection time | >0.342 | <0.34 |
In actual clinical practice and when using TTE, we routinely visualize the MPA through its bifurcation. In the parasternal short axis at the base of the heart, the tri-leaflet aortic valve will be in the center of the screen with the TV on the patient’s right and the pulmonic valve on the patients left. In most cases, the anterior leaflet of the pulmonic valve is visualized. With TTE, it may be necessary to tilt the transducer downward and angle it leftward in order to visualize the MPAP (Figure 11-4 and Video 11-2). With TEE, the pulmonic valve, the MPA, and its bifurcation can be readily assessed on the basal short-axis planes, usually with the transducer tip 25–30 cm from the incisors (Figure 11-5 and Video 11-3). We perform Doppler interrogation of the MPAP to obtain a qualitative assessment of its signal, to detect the normal monophasic systolic spectral Doppler contour or the abnormal biphasic pattern that suggests pulmonary hypertension (Figure 11-6). We do not routinely perform comprehensive Doppler analysis beyond measurement of the pulmonary systolic acceleration time which has utility for assessment for pulmonary hypertension (see below).
Visualization of the PA with TTE or TEE may occasionally allow diagnosis of a centrally located PE (Video 11-4). This is a key finding in evaluation of the patient with RV failure.
For qualitative assessment of RV systolic function, the intensivist observes the endomyocardial thickening and contraction of the RV free wall and IVS from the apical four-chamber view, in a similar fashion as it is done with the qualitative assessment of the LV function. The contractile function of the RV is graded as normal, mild, moderate, or severely reduced. This approach is dependent upon the skills and experience of the interpreter. There are several quantitative techniques to assess RV contractile function. These include ejection fraction; fractional area of contraction; myocardial performance index; tissue Doppler systolic velocity of the tricuspid annulus; and the measurement of Dp/dt from the tricuspid regurgitation jet. We consider that these measurements are not practical for use by the bedside critical care echocardiographer. A simple measurement of RV systolic function that is easy and reproducible to perform is tricuspid annular plane systolic excursion (TAPSE). Systolic RV failure is associated with reduced TAPSE, which may be measured with M-mode interrogation of the tricuspid annulus. The measurement is made from the apical four-chamber view with the M-mode interrogation line placed through the lateral tricuspid annulus. From a frozen image, the displacement of the annulus during systole is measured using the machine caliper function (Figure 11-7). A TAPSE less than 17 mm indicates RV systolic failure.9
RV diastolic overload is synonymous with RV dilation. There are multiple definitions of right heart dilation. It is difficult to accurately estimate RV volumes with echocardiography because of a failure of geometric models to appropriately reflect the complicated RV anatomy. To circumvent this problem, Jardin and colleagues have proposed a semi-quantitative assessment of right heart diastolic overload.1 Both ventricular areas were measured at end-diastole by tracing the endomyocardium from the apical four-chamber view. When the endomyocardium was not well visualized, the area was traced to the epicardium. The authors found that the RV end-diastolic area to left ventricular end-diastolic area (RVEDA:LVEDA) ratio correlated well with RV dilation. The normal RVEDA:LVEDA ratio was found to be between 0.36 and 0.6 (0.48 ± 0.12). They defined moderate dilation as a ratio = 0.7–0.9, and severe dilation as a ratio ≥1. Fremont et al. confirmed the prognostic value of end-diastolic area (measured at the beginning of the QRS complex) RVEDA:LVEDA ratio in patients with acute PE.10 In their registry of 1416 hospitalized patients with acute PE, 31 subjects (3.3%) died. Among patients with systolic blood pressure ≥90 mmHg, the mortality rate was 3.3% for those with a RV/LV ratio ≥0.6, and 1.1% for those with a ratio <0.6. Using mortality receiver operating curves, an RV/LV ratio ≥0.9 had the best sensitivity (72%) and specificity (58%) to discriminate between those patients with the highest risk of dying: 6.6% if the ratio ≥0.9 and 1.9% if <0.9. Chamber size in this study was frequently measured in the parasternal or subcostal views. This is important, as the subcostal view is frequently the best TTE view in critically ill patients on ventilatory support (Figure 11-8 and Video 11-5).
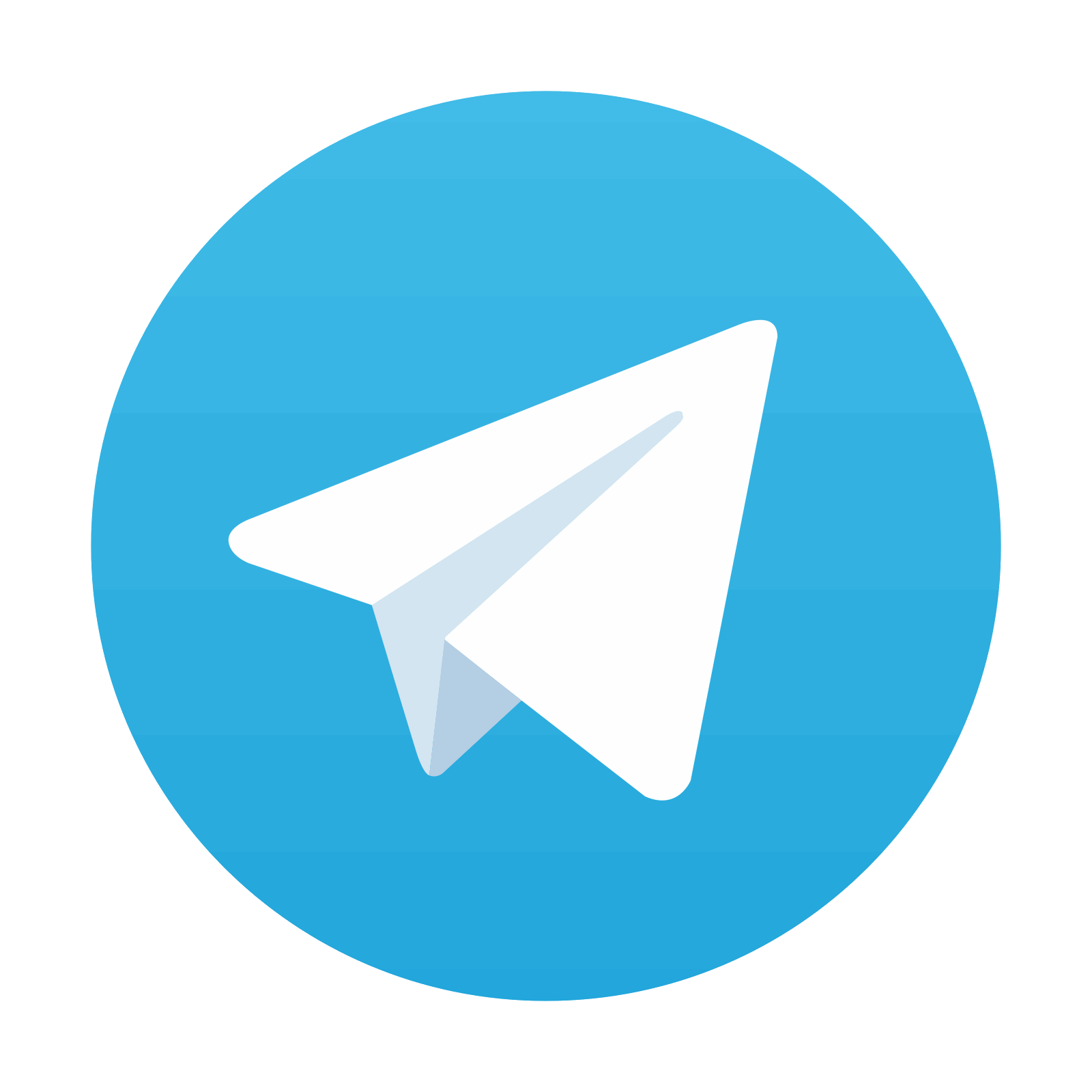
Stay updated, free articles. Join our Telegram channel

Full access? Get Clinical Tree
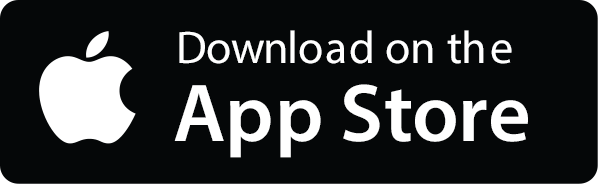
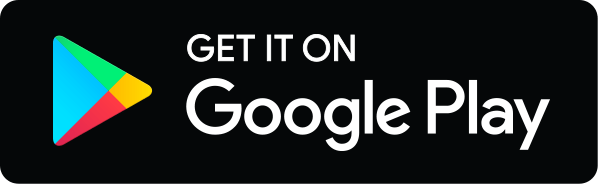