Chapter 110 Drowning
Definitions
The definition of drowning events remains a source of confusion. In addition to drowning, various terms such as near-drowning, suffocation by submersion in water, secondary drowning, immersion syndrome, and wet and dry drowning have been used. The plethora of terms and the lack of a standard definition have made it difficult to analyze and compare studies and outcomes. In June 2002, the World Congress on Drowning was convened to develop a more standardized definition of drowning using the Utstein-style for uniform reporting of data, and to make recommendations regarding preventive measures and care. The Congress was initiated by the Maatschappij tot Redding van Drenkelingen (Dutch Organization to Rescue People from Drowning), an organization established in Amsterdam in 1767 to promote drowning awareness in the Netherlands.1 The final recommendation of the Congress was to define drowning as the process of experiencing respiratory impairment from submersion/immersion in liquid. The definition implies that a drowning victim develops an air-liquid interface that prevents the breathing of air. All other terms such as near-drowning and secondary drowning were abandoned. Outcome is described according to death or survival with survivors further categorized according to neurologic function. Papa et al. performed a systematic review of definitions for drowning accidents in 2005 and identified at least 43 publications where various definitions of drowning were used (Table 110-1).2 They concluded that there is a need to use a single, uniform definition of drowning and supported the one recommended by the Utstein Focus World Congress on Drowning.
Table 110–1 Categories and Terms Used to Describe Drowning
Terms | Explanation |
---|---|
SPECIFIC CATEGORIES | |
Primary vs. secondary | “Secondary drowning” is delayed death from drowning from complications or death occurring in minutes to days after initial recovery. |
Wet vs. dry/with aspiration vs. without aspiration | “Dry drowning” or “without aspiration” is laryngeal spasm with no or little aspiration of water or from respiratory obstruction and asphyxia from a liquid medium. |
Warm vs. cold water | “Cold water drowning” is drowning in an outside body of water during the autumn, winter, and early spring months with a patient core temperature of ≤32° C on arrival to the emergency department. Some use water temperature <20° C. |
Salt vs. fresh water | Kind of water in which incident occurred. |
Active vs. passive (or silent) | “Active” refers to a witnessed drowning event in which victim makes some motion. In “passive” drowning, the victim is found motionless. |
Intentional vs. nonintentional | Describes cause. |
Fatal vs. nonfatal | Describes outcome. |
Submersion vs. immersion | “Submersion” suggests the head was submerged in water; “immersion” suggests the head was out of water. |
With and without hospitalization | Whether victim was admitted to hospital. |
SPECIFIC CIRCUMSTANCES | |
Iceberg phenomenon | People who have been submerged but have subsequently not died from drowning. |
Immersion frigida | Death from cooling in water. |
Immersion syndrome/immediate disappearance syndrome | Occurs when syncope is provoked by sudden contact with water at least less than 5° C, presumably from bradycardia, tachycardia, or arrhythmia. |
Save | Rescue of victim from water by someone who perceived individual to be a potential victim of submersion injury. |
From Papa L, Hoelle R, Idris A: Systematic review of definitions for drowning incidents, Resuscitation 65:255–264, 2005.
Epidemiology
In 2005, the latest year for which statistics are available, drowning accounted for 30% of all deaths in children from 1 to 4 years of age.3 Drowning is the second-leading cause of unintentional injury-related deaths in children age 1 to 14 years. Those younger than 5 years and males aged 15 to 19 years are the two groups most at risk. Males are four times more likely to die from unintentional drowning than females. Between 2000 and 2005, the mortality rate from drowning of African Americans of all ages was 1.3 times that of whites, and among children age 5 to 14 years, the rate was 3.2 times higher. Cultural and racial differences in access to swimming pools and emphasis on swimming skills may play a role in the difference in drowning rates. Children younger than 1 year most often drown in bathtubs, buckets, or toilets. Child abuse should be investigated in these situations.4 As many as 35% of children between the ages of 10 to 18 months are able to climb into a bathtub.5 Drowning in a bathtub should not therefore be considered as a priori evidence of child abuse. Others at risk for bathtub drowning are those with seizure disorders.6 The residential swimming pool is the most common site for drowning in children younger than 5 years, whereas older children and adults more frequently drown in canals, lakes, ponds, and oceans.7 Drowning is highest during the summer months. Most children who drown were last seen inside the home, in the care of one or both parents, but left unsupervised for less than 5 minutes.8
Other important risk factors in drowning deaths include failure to wear a life jacket and alcohol use. In 2006, the U.S. Coast Guard reviewed reports of boating incidents. Of the 500 people who drowned, 9 of 10 were not wearing life jackets.9 Alcohol use is involved in up to half of adolescent and adult deaths associated with water recreation.10 Ethanol and other neurotropic agents can diminish manual dexterity, impair judgment, and increase risk-taking behavior. Recent alcohol consumption by supervising adults may also contribute to submersion accidents involving children.11 Expert swimmers have also been known to drown during underwater swimming. The practice of hyperventilation to prolong the duration of underwater swimming is particularly hazardous in this regard because significant hypoxemia may result in loss of consciousness before hypercarbia stimulates respiration and alerts the swimmer of the urgent need to breathe.12
Review of the drowning literature suggests that the key to prevention includes careful supervision of children, and education of the public regarding drowning prevention and the hazards of drowning. Children playing near or in water should always be supervised by a responsible adult who is not distracted by any other activity. Alcohol should be avoided before or during swimming, boating, and while supervising children. A four-sided, self-closing, self-latching fence at least 4 feet high that completely separates the house and play area of the yard should be installed around household pools. Those who are in or around natural bodies of water should wear U.S. Coast Guard approved life jackets irrespective of distance to be travelled, size of boat, or swimming ability.9
Pathophysiologic Considerations
The sequence of events after submersion has been described by Karpovich13 in an animal model. After the initial panic and violent struggle, automatic swimming movements are followed by breath-holding and swallowing of large amounts of water. Subsequently, water is aspirated into the lungs as a result of attempts to breathe. Convulsions and spasmodic efforts resulting from asphyxia precede death. The single most important and prognostically significant consequence of drowning is decreased oxygen delivery to the tissues. A number of clinical variables determine the magnitude of hypoxia and the subject’s ability to withstand it. The pathophysiology of drowning is thus closely integrated with the genesis of hypoxemia and its effects on various organ functions. A working knowledge of these pathophysiologic principles and multiorgan involvement is extremely helpful in directing therapeutic strategies for optimum survival.
Type of Aspirated Fluid
Although the differences between changes in electrolytes and blood volume after salt and fresh water aspiration have been emphasized in the past, they are of little clinical significance in patients who survive long enough to be transported to a medical facility.14 Aspiration of more than 11 mL/kg of fluid is required for blood volume to be altered, and aspiration of more than 22 mL/kg is necessary before significant electrolyte changes occur.15,16 Most drowning victims aspirate less than 3 to 4 mL/kg of fluid. Hypervolemia resulting from fresh water aspiration is rarely a problem. Most drowning victims are hypovolemic regardless of the type of aspiration because of excessive capillary permeability resulting from asphyxia and the loss of protein-rich fluid into the alveoli.
Pulmonary Effects
Functional residual capacity (FRC) is the only source of gas exchange at the pulmonary capillary level in the submerged state. Increased metabolic demands from struggling, breath-holding, a depletion of FRC from breathing efforts, and aspiration of fluid into the lungs all result in seriously compromised O2 uptake and CO2 elimination, with consequent hypoxia and hypercarbia. Between 10% and 15% of drowning victims have severe laryngospasm after submersion resulting in fatal asphyxiation without aspiration of water into their lungs (Figure 110-1). A combined respiratory and metabolic acidosis caused by hypercapnia and anaerobic metabolism is often encountered. Patients without significant fluid aspiration recover from asphyxia rapidly if they are successfully resuscitated before cardiac arrest or irreversible brain damage occurs. Aspiration of fluid, however, results in persistently abnormal gas exchange. Aspiration of a little as 1 to 3 mL/kg of fluid results in profound impairment of gas exchange.16,17 Soon after the aspiration of fluid, there is an elevation of PaCO2 and a fall in PaO2 as a result of airway obstruction, hypoventilation, and impaired gas exchange between alveoli and pulmonary capillary blood. With adequate resuscitation, normocapnia or even hypocapnia is usually achieved while hypoxemia persists, indicating a significant ventilation/perfusion mismatch and diffusion defect leading to intrapulmonary shunting and venous admixture.18
The surfactant system of the lung is affected differently in fresh water and seawater aspiration.19 Freshwater aspiration results in marked disruption of the surfactant system of the lung resulting in alveolar instability and atelectasis. Seawater, because of its hypertonicity, draws water into the alveoli. Although the surfactant may be diluted by the presence of seawater in alveoli, its surface tension properties are not significantly altered. Zhu et al.20 examined serum levels of pulmonary surfactant-associated protein and lung weights in 53 victims of fatal drowning. They showed significantly heavier lungs in those who drowned in seawater versus freshwater, suggesting an osmolar effect. Although they found no difference in serum surfactant-associated protein, intra-alveolar aggregates of pulmonary surfactant associated protein were noted more frequently in those who drowned in freshwater. This is likely related to the disruption of surfactant noted in freshwater drowning. Karch demonstrated marked changes in the pulmonary vasculature in rabbits within 30 minutes of aspiration of both freshwater and salt water.21 Mitochondrial swelling and disruption of pulmonary vascular endothelial cells were consistently observed in these experiments. Clinically, pulmonary abnormalities are encountered in both fresh and sea water aspiration. These are consistent with pronounced injury to alveoli and pulmonary capillaries resulting in increased membrane permeability, exudation of proteinaceous material in alveoli, pulmonary edema, decreased lung compliance, and increased airway resistance. The extent of these abnormalities may not be manifested fully for several hours after the submersion episode and may be progressive in nature.
Acute respiratory distress syndrome (ARDS) is the hallmark of delayed pulmonary insufficiency resulting from aspiration in drowning. This is characterized by progression of alveolar-capillary block, increased capillary permeability, and pulmonary edema. Reduced FRC and diffusion barrier resulting from accumulation of fluid and inflammatory cells in the alveoli and interstitium further accentuate hypoxemia. Aspiration of stomach contents and other debris such as sand, mud, and algae may also impair gas exchange. Bacterial pneumonia resulting from aspiration of contaminated water may further contribute to pulmonary insufficiency. A number of factors contribute to drowning-associated pneumonia. These include aspiration of vomitus or aspiration of contaminated material from water that may contain sewage. Water temperature plays a role, with warmer temperatures predisposing to a higher number of organisms. The chemical composition of the water such as pH, salt content, and presence of organic and inorganic substances influence bacterial growth as well. Organisms include aerobic gram-negative bacteria such as Klebsiella and Pseudomonas, and gram-positive bacteria, which include Streptococcus pneumoniae and Staphylococcus aureus. Fungi such as aspergillus have been reported to cause drowning-associated pneumonia as well. The diagnosis of pneumonia is based on clinical parameters such as fever, leukocytosis, respiratory cultures, and new infiltrates on chest radiographs.
Cardiovascular Effects
Profound cardiovascular instability is often encountered after a severe submersion event thereby posing an immediate threat to survival after the initial rescue. The hypoxemia that occurs because of ventilation-perfusion mismatch can cause life-threatening dysrhythmias such as ventricular tachycardia, ventricular fibrillation, and asystole. The two determinants of oxygen delivery, namely cardiac output and arterial O2 content, can be adversely affected by the submersion event. A decrease in PaO2, if sufficiently severe, decreases oxygen saturation and therefore arterial O2 content. This decrease in arterial O2 content can cause a decrease in myocardial oxygen delivery, which contributes to worsening cardiac output and decreased myocardial perfusion pressure. Smooth muscle contraction banding in the media of major coronary arteries and local ventricular myocytes with focal myocardial necrosis have been described after a submersion episode.22,23 Cytosolic calcium overload and oxygen-derived free radicals have also been implicated in the mechanism of myocardial injury after resuscitation after cardiac arrest.24 Cardiogenic shock may result from hypoxic damage to the myocardium. Metabolic acidosis may further impair myocardial performance. Additionally, therapeutic application of PEEP causes decreased venous return, right and left ventricular preload, and right ventricular afterload while decreasing left ventricular afterload. Right ventricular afterload is also increased by structural pulmonary microvascular damage and humoral inflammatory mediators involved in ARDS. The right ventricle is anatomically designed to tolerate increased preload, but it is not as tolerant of high pressures and afterload as the left ventricle. If pulmonary hypertension is severe enough, it may lead to decreased left ventricular preload due to right ventricular failure. These factors, as well as the excessive capillary permeability of pulmonary and systemic capillaries, result in hypovolemia and decreased left ventricular filling pressures. These alterations of components of oxygen delivery; namely oxygen content, myocardial contractility, left and right ventricular afterload, and preload, can potentially result in an inadequate supply of oxygen to tissues to meet their metabolic demands.
Central Nervous System Effects
There are several developmental factors that render the neurologic effects of pediatric cardiac arrest and hypoxia different than that of adults. Similar to other causes of pediatric asphyxia, such as foreign body airway obstruction, apnea, asthma, and suffocation, cardiac arrest encountered after drowning is preceded by a period of hypoxic perfusion. This leads to worse neurologic injury as opposed to a sudden cardiac arrest, such as that induced by a dysrhythmia, which is the more common precipitating factor for adult cardiac arrest.25,26 Approximately 13% of all children with cardiopulmonary arrest survive to hospital discharge, and only 9% if the arrest occurred outside the hospital.27 Studies in humans and animals indicate that there are several developmental differences in excitotoxic pathways. In the neonatal period, there is increased vulnerability to NMDA receptor activation28 and glutamate toxicity,29 as well as heightened capability for apoptosis, which, albeit an important process of normal brain development, may render the immature brain vulnerable to neuronal loss after an insult.30 In addition, a developmental difference exists in cerebral blood flow (CBF) after cardiac arrest. In adult models of cardiac arrest, global hyperemia is present for 15 to 30 minutes after return of spontaneous circulation followed by delayed hypoperfusion that persists for several hours. In an immature animal model of a brief asphyxial cardiac arrest, hyperemia is observed for 10 minutes after return of spontaneous circulation followed by restoration of baseline CBF, whereas prolonged cardiac arrest is followed by hypoperfusion and blood pressure-dependent CBF.31
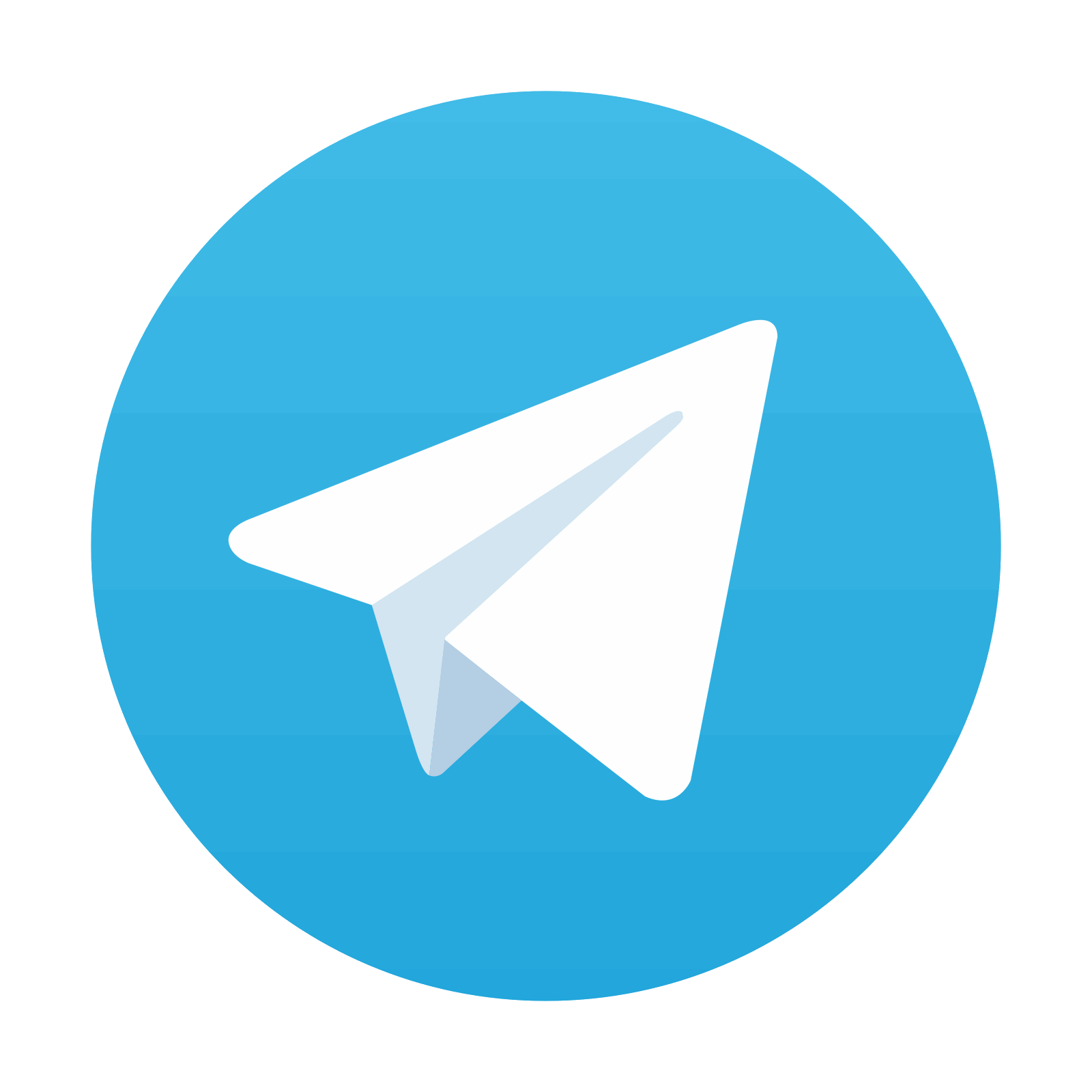
Stay updated, free articles. Join our Telegram channel

Full access? Get Clinical Tree
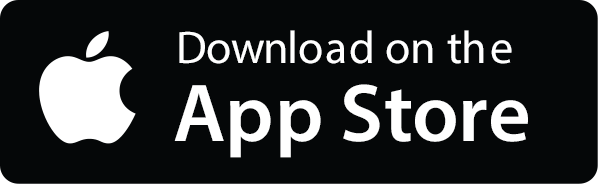
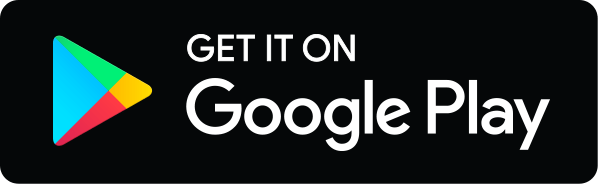