Fig. 13.1
Physiological functions of a2 adrenoceptor subtypes derived from gene-targeted mouse models. Endogenous catecholamines stimulate a2 adrenoceptors to mediate essential physiological functions (upper panels): The a-2B adrenoceptor subtype is required for vascular development of the placenta in mice, and a-2A and a-2C adrenoceptor subtypes regulate catecholamine release from sympathetic nerves and the adrenal gland, respectively. Pharmacological stimulation of a2 adrenoceptors results in a variety of biological effects (lower panels). From Brede M, Philipp M, Knaus A, et al. Alpha-2 adrenoceptor subtypes—novel functions uncovered in gene-targeted mouse models. Biol Cell. 2004; 96:343–48. Used with permission from John Wiley and Sons
The physiological effects of DEX are mediated via postsynaptic alpha-2 adrenoceptor and activation of a pertussis toxin-sensitive guanine nucleotide regulatory protein (G protein) resulting in decreased adenyl cyclase activity [20]. A reduction of intracellular cyclic adenosine monophosphate (cAMP ) and cAMP-dependent protein kinase activity results in the dephosphorylation of ion channels [21]. Alterations in ion channel function, ion translocation, and membrane conductance lead to decreased neuronal activation and the clinical effects of sedation and anxiolysis. The hypnotic effect of DEX is mediated by the change in membrane ion conductance which leads to a hyperpolarization of the membrane, which suppresses neuronal firing in the locus ceruleus as well as activity in the ascending noradrenergic pathway. Decreased noradrenergic output from the locus coeruleus allows for increased firing of inhibitory neurons including gamma aminobutyric acid (GABA ) which further inhibited the locus ceruleus and tuberomamillary nucleus. This inhibitory response also causes a decrease in the release of histamine which results in a hypnotic response. There is some evidence that sedation induced with DEX resembles normal sleep [4, 22]; in rats, the pattern of c-Fos expression (a marker of activation of neurons) is qualitatively similar to that seen during normal NREM sleep, suggesting that endogenous sleep pathways are causally involved in DEX-induced sedation [4].
Centrally acting alpha-2 adrenergic agonists also activate receptors in the medullary vasomotor center reducing norepinephrine with a resultant central sympatholytic effect leading to decreased heart rate (HR) and blood pressure (BP). The sedative and anxiolytic effects of DEX result primarily from stimulation of parasympathetic outflow and inhibition of sympathetic outflow from the locus coeruleus in the brainstem.
The precise mechanisms and pathways by which DEX induces analgesia have not been fully elucidated. The brain, spinal cord, and peripheral mechanisms all seem operant. The most important of these sites may be the spinal cord, where the analgesic effects are believed to be related with the activation of both α2-C and α2-A, situated in the neurons of the superficial dorsal horn especially in the lamina II [23–25], which directly decreases pain transmission by reducing the release of pro-nociceptive transmitter, substance P, and glutamate from primary afferent terminals and by hyperpolarizing spinal interneurons via G-protein-mediated activation of potassium channels. Suppression of activity in the descending noradrenergic pathway may also modulate nociceptive neurotransmission and terminate propagation of pain signals leading to analgesia [26]. One should be aware that the analgesic potential of DEX, however, does not approximate the potency of opioids.
The pharmacokinetic profile of DEX includes a rapid distribution phase (6 min), a terminal elimination half-life of approximately 2 h [3, 27, 28], and a steady-state volume of distribution of 118 l. DEX exhibits linear kinetics over the recommended dosage range of 0.2–0.7 mcg/kg/h delivered via continuous intravenous infusion for up to 24 h. DEX is 93 % protein bound [3] and is extensively metabolized through both the cytochrome P450 enzyme system, primarily by CYP2A6, and direct glucuronidation in the liver to inactive metabolites [29]. A very small fraction of DEX is excreted unchanged in urine and feces. DEX administration has been described by intravenous, intramuscular, intranasal (IN) [30], and buccal route [31]. Via the nasal and buccal route, DEX bioavailability approximates 65 % (35–93 %) and 81.8 % (72.6–92.1 %), respectively [32, 33]. The bioavailability of DEX by the oral route is very poor (16 %) and administration by such a route is unwarranted [32].
Information on the pharmacokinetics of DEX in the pediatric population is limited, especially in children younger than 2 year of age. Infants appear to require larger doses of DEX compared with older children [34]. A recent study that examined pharmacokinetics of intravenous DEX in children under 11 years of age showed that total plasma clearance is similar in younger and older children, but the volume of distribution and the terminal elimination half-life were greater in children younger than 2 years of age compared with older children [28]. A recent study that examined the pharmacokinetics of DEX in 23 neonates (age, 1 day–1 month) and 36 infants (age, 1 month–24 months) after open heart surgery showed that continuous infusions of up to 0.3 mcg/kg/h in neonates and 0.75 mcg/kg/h in infants were well tolerated. The study concluded that DEX clearance is significantly diminished in full-term newborns and increases rapidly in the first few weeks of life. The dependence of clearance on age during the first few weeks of life most likely reflects the relative immaturity of metabolic processes during the newborn period [35].
A recent study examined the pharmacokinetics of prolonged infusion (maximum duration of 14 days) of high dose of DEX in critically ill patients [36]. The authors quantified for the first time in humans the concentrations of the previously poorly characterized H3 metabolite of DEX. The result of their study suggests that DEX obeys linear pharmacokinetics up to the dose of 2.5 mcg/kg/h. They could not establish any new safety findings despite the high dosing regimen and prolonged infusions. Abrupt cessation of DEX may produce withdrawal symptoms similar to those seen with clonidine withdrawal (i.e., agitation, irritability, headache, and rebound hypertension). In clinical practice DEX has been used for more than 24 h. However, the manufacturer recommends that DEX not be used for more than 24 h [29].
End-Organ Effects of Dexmedetomidine
Effect of Dexmedetomidine on Respiration
The recent enthusiasm for DEX use for variety of pediatric and adult procedures stems from the ability to maintain ventilation and airway patency in the presence of increasing level of sedation especially in patients with obstructive sleep apnea (OSA). The administration of anesthetic/sedative agents increases airway collapsibility due to increase closing pressure [37], loss of pharyngeal muscular tone [38], and failure of coordination of phasic activation of upper airway muscles with diaphragmatic activity [39]. These agents depress upper airway dilator muscle activity and diaphragm muscles to varying degrees. One possible explanation for the difference between the anesthetic/sedative agents on airway collapsibility can be attributed to the impact of pharmacologic mechanisms of the sedative or sleep-inducing actions on processes that control motor efferents to pharyngeal musculature as well as afferents from airway mechanoreceptors. In contrast to traditional sedative agents, DEX produces a state closely resembling physiological sleep [4, 5], which gives further support to earlier experimental evidence for activation of normal NREM pathways. A recent study compared the respiratory effect of DEX to that of remifentanil in six healthy male volunteers and reported similarity between the hypercapnic arousal phenomenon during DEX infusions and natural sleep [22].
The ability to maintain spontaneous ventilation and upper airway tone makes DEX an attractive choice for sleep endoscopy and dynamic airway imaging [40–42] [43]. Providing anesthesia that mimics physiological sleep and the need to avoid the use of airway interventions in these procedures is a challenge but is critical for accurate interpretation of the airway evaluation [44, 45]. A recent retrospective study reviewed the records of 52 children receiving DEX and 30 children receiving propofol for anesthesia during MRI sleep studies and showed that the total number of airway interventions was significantly less in the DEX group than in the propofol group [40]. The same authors examined the effects of increasing depth of DEX anesthesia on upper airway morphology in 23 children with normal airway. Images of the upper airway were obtained during low (1 mcg/kg/h) and high (3 mcg/kg/h) dose of DEX anesthesia. The authors concluded that increasing doses of DEX in children with normal airway are associated with statistically significant reductions in airway dimensions at the level of the posterior nasopharynx and retroglossal airway; these changes were small in magnitude and do not appear to be associated with clinical signs of airway obstruction in [41].
Recently a prospective, single-blinded controlled comparative study examined the dose–response effects of DEX and propofol on airway morphology in children and adolescents with OSA. MRI images of the upper airway measurements were obtained during low (1 mcg/kg/h) and high (3 mcg/kg/h) doses of DEX or low (100 mcg/kg/min) and high (200 mcg/kg/min) doses of propofol. Most airway measurements demonstrated statistically nonsignificant associations with increasing doses of propofol and DEX. As dosage increased, average airway dimensions were typically unchanged or slightly increased with DEX compared to unchanged or slightly decreased with propofol. An airway intervention was required for oxygen desaturation in three children (11 %) in the DEX group versus seven children (23 %) in the propofol group. The authors concluded that both agents provided an acceptable level of anesthesia for sleep cine MRI studies in patients with OSA with statistically nonsignificant changes in airway dimensions [42].
Cardiovascular and Hemodynamic Effects
The hemodynamic effects of DEX result from peripheral and central mechanisms. DEX displays a biphasic, dose-dependent BP response. The initial response to rapid DEX infusion may be a transient hypertension related to stimulation of peripheral postsynaptic alpha-2B adrenergic receptors which results in vasoconstriction, whereas the eventual decrease in BP and HR results from central presynaptic alpha-2A adrenergic receptor stimulated sympatholysis. Hypotension and bradycardia associated with DEX administration have been reported in adults and children, especially in the presence of comorbid cardiac disease, when administered with other medications that possess negative chronotropic effects or following large [3] or rapid bolus doses. DEX can mediate a significant increase in BP when the plasma concentration of DEX increases from 0.5 to 3.2 ng/ml. The pressor effect of DEX has been shown to correlate with the rate of IV infusion and plasma concentration of the drug [46]. In general, at serum concentrations greater than 1 mcg/l, the BP changes from a mild decrease from baseline to an elevation (Fig. 13.2) [47]. Although decreases in HR and a biphasic effect on BP are observed with increasing doses of DEX, the literature supports that concurrent hemodynamic collapse or need for pharmacologic resuscitation does not occur. Rather, a recent publication suggests that the bradycardia associated with alpha-2 adrenergic agonists in children may not require treatment nor for adverse hemodynamic consequence [48]. Although bradycardia and hypotension have been described in patients receiving DEX, to date, there are only rare reports of clinically significant bradycardia with the use of DEX in infants and children. This exaggerated physiological effect seems to be related to the use of a loading dose and/or preexisting hypovolemia, and the occurrence of hypotension can be attenuated by pretreatment with balanced salt solution boluses [49].
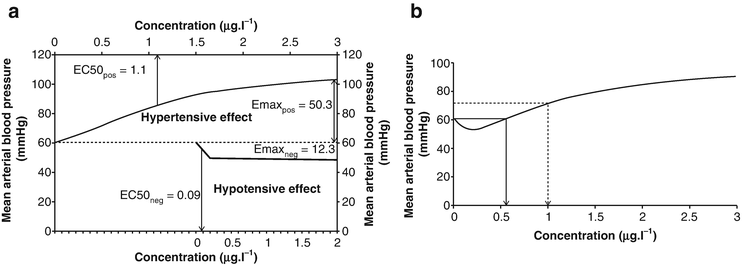
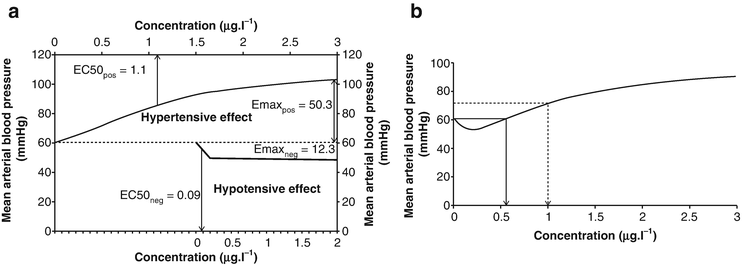
Fig. 13.2
Hyper- and hypotensive effect of dexmedetomidine on mean arterial blood pressure. (a) Composite Emax model, showing hyper- and hypotensive effect of dexmedetomidine on mean arterial blood pressure (MAP). (b) Combined hyper- and hypotensive effect of dexmedetomidine on MAP. The solid arrow indicates the concentration at which the hypertensive effect begins; the dashed arrow indicates the concentration producing a 20 % increase in MAP from baseline. From Potts AL, Anderson BJ, Holford NH, Vu TC, Warman GR. Dexmedetomidine hemodynamics in children after cardiac surgery. Pediatric anesthesia. 2010;20(5):425–33. Used with permission from John Wiley and Sons
A recent study that utilized a continuous noninvasive cardiac output to measure the hemodynamic effects of DEX sedation in healthy children undergoing radiological imaging studies found a significant decrease in HR within 5–10 min of initiating a DEX bolus (2 mcg/kg bolus over 10 min). HR and cardiac index (CI ) decreased after a single bolus and recovered to baseline within 60 min after a brief exposure (completed the procedure within 10 min). No changes in stroke index (SI) or systemic vascular resistance index were observed after a brief exposure, but prolonged exposure (procedures lasting longer than 10 min) leads to decreases in HR, CI, and SI that did not recover back to baseline.
A common concern with this novel sedative agent is bradycardia. Bradycardia or a decrease in resting HR (up to a 30 % decrease from baseline) is expected and should be considered as a predictable physiologic response anticipated with DEX. The HR responses are rarely of clinical significance, as they do not usually require intervention [3, 48, 50] [51]. An accidental overdose with the administration of DEX up to 0.5 mcg/kg/min and an infusion up to 10 mcg/kg/h did not result in hypotension or severe bradycardia [52, 53]. Extreme bradycardia can occur if administered to a patient receiving digoxin and syncope, likely from a vasovagal response, and has been cited in the literature as well as in the package insert [29, 54, 55]. A recent study examined the effect of DEX on sinus node, atrioventricular node , and conduction pathways and found a decrease in HR with significant depression of sinus and atrioventricular nodal function in a clinical study in pediatric patients undergoing electrophysiological study [56]. However, a prospective observational controlled study in pediatric patients with congenital heart disease showed DEX does not have direct effect on cardiac conduction [57]. In healthy women undergoing elective hysterectomy, a 6.2 % prevalence of intraoperative and 14.1 % prevalence of postoperative bradycardia (HR less than 40 beats/min) were noted in patients premedicated with intramuscular DEX (2.5 mg/kg) when administered with or without fentanyl [58]. Severe bradycardia (HR, 27 beats/min) and transient sinus arrest (20–30 s) have been reported in two healthy subjects who had received intramuscular DEX [59].
Although there are no absolute contraindications to DEX in the literature or package insert, it is recommended that DEX be avoided in patients with depressed left ventricular function, recent high-degree AV block, and volume depletion and in children receiving digoxin, beta adrenergic blockers, calcium channel blockers, or other agents which predispose to bradycardia or hypotension.
Caution should be exercised when administering anticholinergics to treat isolated DEX-associated bradycardia in children, as intravenous glycopyrrolate has been shown to elicit immediate, significant hypertension [60]. In dogs, administration of intramuscular atropine with intramuscular DEX reversed HR changes and hypotension, but arrhythmias (atrioventricular block, premature ventricular contractions, and bigeminy) were observed [61]. A recent retrospective study showed that the administration of prophylactic anticholinergic (atropine or glycopyrrolate) administration prior to DEX in pediatric imaging studies did not show any advantage other than a transient clinically insignificant increase in HR and systolic blood pressure (SBP) (Fig. 13.3a, b). In fact transient exaggerated SBP was noted in a greater number of patients who received anticholinergics as compared to not using prophylactic anticholinergic [62].
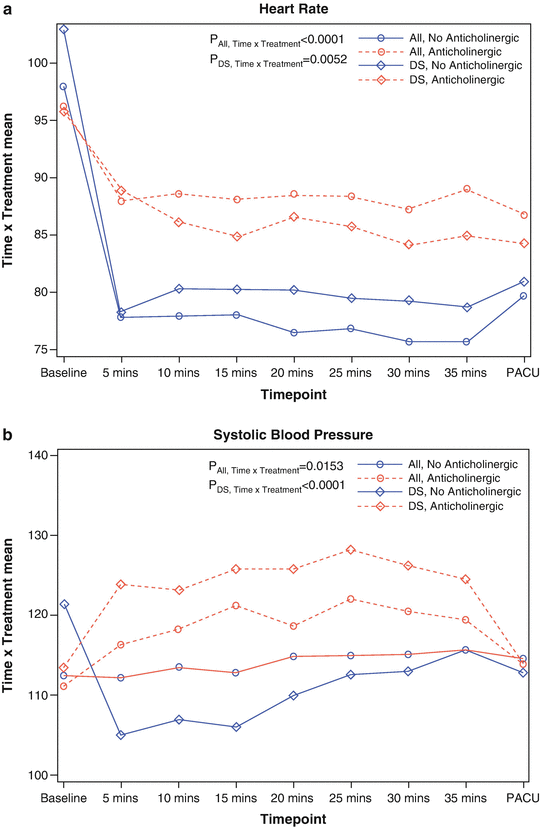
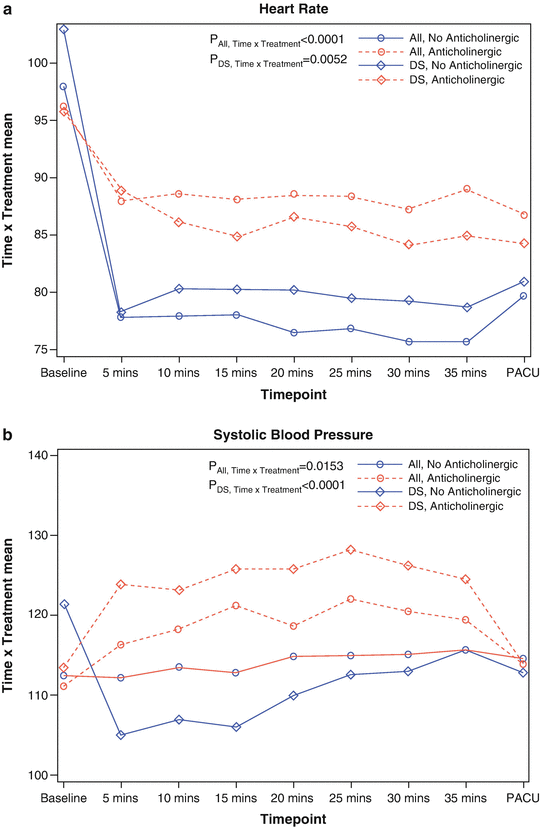
Fig. 13.3
(a) Changes in heart rate after DEX sedation attributed to receiving or not receiving an anticholinergic pretreatment. In this retrospective descriptive study, we reviewed the records of 163 children receiving dexmedetomidine anesthesia during MRI studies. The heart rate between the patients receiving an anticholinergic and not receiving an anticholinergic at baseline, during the scan period, and in the post-anesthesia care unit (PACU) is shown and compared. The x-axis represents the heart rate at baseline (before the start of the scan), during the scan at 5-min intervals (5 min through 35 min), and in the PACU. The y-axis indicates the least squares estimates of time and treatment interaction mean effect. There was a significant reduction in the heart rates during scan period when an anticholinergic was not used compared with using a prophylactic anticholinergic in all patients (P < 0.0001) and in patients with Down syndrome (DS) (P = 0.0052). The solid line and the broken line with the round dot indicate the heart rate for all patients. The solid line and the broken line with the diamond dot indicate the heart rate for patients with DS. (b) Changes in systolic blood pressure after DEX sedation attributed to receiving or not receiving an anticholinergic pretreatment. In this retrospective descriptive study, we reviewed the records of 163 children receiving dexmedetomidine anesthesia during MRI studies. The systolic blood pressure between the patients receiving an anticholinergic and not receiving an anticholinergic at baseline, during the scan period, and in the post-anesthesia care unit (PACU) is shown and compared. The x-axis represents the systolic blood pressure at baseline (before the start of the scan), during the scan at 5-min intervals (5 min through 35 min), and in the PACU. The y-axis indicates the least squares estimates of time and treatment interaction mean effect. There was a significant reduction in systolic blood pressure during scan period when an anticholinergic was not used compared with when a prophylactic anticholinergic was used in all patients (P = 0.0153) and in patients with Down syndrome (DS) (P < 0.0001). The solid line and the broken line with the round dot indicate the systolic blood pressure for all patients. The solid line and the broken line with the diamond dot indicate the systolic blood pressure for patients with DS
ECG abnormalities as noted by RR prolongation and junctional escape rhythms at 2 mcg/kg, administered as a single dose, have been reported with DEX use [6]. The concomitant administration of DEX with medications that have negative chronotropic effects (propofol, pyridostigmine, succinylcholine, and remifentanil) may potentiate vagotonic or negative chronotropic effects [63]. Asystole following 0.5 mg atropine to treat a HR in the 1930s was reported in a 52-year-old woman receiving a DEX infusion during general anesthesia (fentanyl, propofol, sevoflurane) [64].
The DEX package insert recommends administering the drug (1 mcg/kg) over a 10-min infusion to attenuate dose-dependent, biphasic, hemodynamic response caused by peripheral α2 adrenoreceptor stimulation and subsequent central α2 adrenoreceptor-mediated sympatholysis. However, rapid infusion of 0.25, 0.5, 1.0, or 2.0 mcg/kg DEX over 2 min was well tolerated in healthy volunteers with a biphasic hemodynamic effect observed initially and eventual decrease from baseline of the mean arterial pressure (MAP) at 60 min of 14, 16, 23, and 27 % [6]. Rapid boluses in small doses (0.25 and 0.5 mcg/kg) have been shown to be well tolerated in 12 pediatric heart transplant patients undergoing cardiac catheterization. The denervation of the sinoatrial node, however, should be considered as a potential factor for the minimal observed response [46]. A recent study examined the dose–response DEX bolus over 5 s administered to healthy children. The maximum elevation in MAP was a 33 % increase from baseline and the maximum decrease in HR was 36 % from baseline (Fig. 13.3). An ED50 (no hemodynamic response in half of the subjects) of 0.49 mcg/kg was extrapolated to avoid hemodynamic responses in half of the subjects [65].
In addition to its potential to cause hypotension, hypertension, and bradycardia, a significant area of concern is DEX’s effect on pulmonary vascular resistance (PVR ). The results of studies on the effect of DEX on pulmonary hemodynamics vary along with the doses and study conditions that were used. At present, there is limited information regarding the effect of DEX on the pulmonary vasculature and PVR in adults and children with varying degrees of pulmonary hypertension (PH). The perioperative infusion of DEX without a loading dose is not associated with any increase in the mean pulmonary artery pressure (MPAP) [66, 67]. In the animal model, DEX (2 mcg/kg over 1 min) transiently increased MPAP and PVR [68]. Similar transient pulmonary hemodynamic changes have been shown in healthy adult volunteers subjected to increasing DEX infusions to a plasma concentration of 1.9 ng/ml [6]. Post cardiac surgery, DEX doses as permitted by labeling had minimal effect on the pulmonary artery pressure, leaving ventricular function unchanged [69].
The effect of DEX on infants and children with pulmonary hypertension can be variable. Children with pulmonary hypertension as compared to those without both demonstrated no significant change in pulmonary vascular resistance, pulmonary artery pressure, and cardiac index in response to up to 1 mcg/kg DEX bolus followed by a continuous infusion of 0.7 mcg/kg/h [70]. In contrast, a recent FDA-monitored study evaluating the effect of DEX on PVR in children with pulmonary hypertension was terminated due to increased PVR in addition to premature ventricular complexes, bradycardia (<60 bpm), and hypotension in one of the subjects [71]. Another prospective observational pilot study demonstrated that DEX after congenital cardiac surgery did not have a demonstrable effect on pulmonary artery pressure of children who did not have pulmonary hypertension [69]. Given the potential impact of these findings on infants and children with preexisting pulmonary hypertension, future studies are needed before advocating routine DEX in this patient population.
Endocrine, Renal, and Hepatic Effects of Dexmedetomidine
Because DEX contains an imidazole ring, there are theoretical concerns regarding its effects on steroidogenesis. In the concentrations that are used clinically, there is no evidence to suggest that can depress adrenocortical function to the extent that occurs with etomidate [72]. DEX administration for up to 7 days in dogs failed to suggest any adrenal shock or severe impairment of the hypothalamic–pituitary axis [73].
There seems to be no evidence of significant accumulation of any metabolic products that would limit the prolonged use of DEX. DEX has been used more than 2 months on a 9-month-old infant with liver cirrhosis, who underwent liver transplantation. The respiratory conditions improved when DEX was added to midazolam and fentanyl. The infant was then successfully extubated 10 weeks later. No serious adverse effects or disturbance of liver function was found when DEX was used as a prolonged infusion up to 1.4 mcg/kg/h [74]. DEX was also used in six patients with severe renal impairment. The authors showed that there was no difference between renal disease and control groups. However, DEX resulted in a more prolonged sedation in subjects with renal disease [75]. A recent study showed that a metabolite called H3 was quantified, but seemed to have no relevant pharmacologic activity [36].
The α2 adrenoreceptors are widely distributed in the renal proximal and distal tubules, peritubular vasculature, as well as in systemic tissues. DEX seems to induce diuresis by inhibiting the antidiuretic action of vasopressin (AVP), enhance renal blood flow and glomerular filtration, and increase urine output [76, 77]. Recent animal studies showed that it can also protect against radiocontrast nephropathy by preserving outer medullary renal blood flow [78]. Anesthesia/sedation providers should also be aware of the potential development of polyuric syndrome when DEX is used. A recent case report in adult patient who underwent posterior spinal fusion under general anesthesia with isoflurane, sufentanil, DEX, and lidocaine infusions showed that urine output increased from 150 to 950 ml/h. An increasing serum sodium, low urine specific gravity, and increased serum osmolarity occurred simultaneously with the polyuria. Within 2 h of discontinuing the DEX infusion, urine output greatly decreased and all signs of the polyuric syndrome resolved spontaneously in 24 h [79]. Despite the diuretic effect of DEX, in adults, the 74 % increase in urinary output that has been seen for up to 4 h post cardiac surgery does not affect renal function when compared to placebo in a double-blind design [80]. There is some data to support a renoprotective effect of DEX. A retrospective review of cardiac and thoracic surgeries in adults who received a DEX infusion of up to 0.6 mcg/kg/h for up to 24 h postoperatively revealed a decrease in a 30-day mortality and decrease in serum markers for acute kidney injury [81]. Similar results were found in children who received intravenous iodine contrast for cardiac angiography. Compared to the control group, children 6 months–6 years of age had decreased elevation in plasma endothelin, renin, and markers of acute renal injury [82]. Future studies are warranted to delineate the patient population, surgical procedures, and resultant effect of DEX on renal function.
Effect of Dexmedetomidine on the Central Nervous System
Because of the complexity associated with normal brain development, the developing nervous system has been hypothesized to be more susceptible than the mature brain to certain neurotoxic insults. The issue of anesthetic-induced neurotoxicity is a topic of ongoing interest and has continued to gain attention and research support over the past decade, as some studies have suggested that inhalational and intravenous anesthetics may both cause neurotoxicity [83–86] and may contribute to neuronal apoptosis in neonates. One of the most interesting directions of DEX research involves its potential for neuroprotection particularly in children. To date, only DEX and possibly xenon have been proposed to be neuroprotective in animal studies [87].
The precise mechanism of the neuroprotective effect of alpha-2 adrenergic agonists is not clear. DEX’s neuroprotective effects may be mediated by a reduction in caspase-3 expression (a proapoptotic factor); increased expression of active (autophosphorylated) focal adhesion kinase (FAK), a nonreceptor tyrosine kinase which plays a role in cellular plasticity and survival; and upregulation of antiapoptotic proteins [88, 89].
Catecholaminergic neurotransmission is also considered to possibly be related to the neuroprotective effect of DEX. Cerebral ischemia is associated with an increase in circulating and extracellular brain catecholamine concentrations. The treatment with agents that are capable of reducing the release of norepinephrine in the brain (e.g., alpha-2 agonists) may provide protection against the damaging effect of cerebral ischemia. Various animal models with complete and incomplete as well as transient and permanent ischemic injury have attempted to define DEX’s protective effects during central nervous system injury [90–92] and attenuated hypoxic-ischemic brain injury in developing brains, highly susceptible to neuronal damage [93, 94]. Moreover, improvement in functional neurological outcomes was shown after brain injury [95]. As mentioned before, the exact mechanisms of neuroprotection are not clear, but catecholamine pathways play an important role, and a positive correlation between circulating norepinephrine and neurological outcome was revealed after cerebral ischemia [96]. Several studies demonstrate that DEX reduces excitatory neurotransmitter (e.g., glutamate) and may protect against excitotoxic injury to the developing brain [93, 97].
It is possible that an opioid-based technique with DEX may be a better alternative than current volatile anesthetic techniques in neonates. Large, multicenter studies designed to assess the effect of DEX on neurocognitive development are required to confirm the neuroprotective beneficial effect in humans.
The effects on memory formation of α2 adrenoceptor agonists in both animals and man remain controversial in the literature. These contradictory findings can be ascribed to the dose used, the type of memory involved, and the timing of drug administration [98]. Several animal studies report disruption of memory formation induced by α2 adrenoceptor agonists. In ten healthy adult male volunteers, sequential 40-min infusions of DEX were administered to achieve plasma concentrations of 0.5, 0.8, 1.2, 2.0, 3.2, 5.0, and 8.0 ng/ml [45]. The two volunteers who received the highest incremental dose (calculated to achieve a plasma concentration of 8.0 ng/ml) were not arousable even with vigorous shaking. Picture recall and recognition were preserved during the lowest incremental infusion (0.5 ng/ml) but were 0 % (0 of 10) and 20 % (2 of 10), respectively, with the second and third infusion levels (0.8 and 1.2 ng/ml). Another study examined recall with DEX confirmed that with increasing serum concentrations of DEX, there is a decrease in the Observer Assessment of Alertness/Sedation (OASS) scale and visual analog scale [99]. With increasing plasma concentrations of DEX administered to healthy adult volunteers, the correct recall or recognition of a picture decreased [99]. There is no literature to date which describes the effect of DEX on memory acquisition, recall, and amnesia in children.
In adults, it has been suggested that on continuous recognition tasks using photograph recognition to differentiate working from long-term memory, DEX impairs familiarity more than recognition [100]. Whether a similar response is seen in children is yet to be determined. Thus, until the effect of DEX on amnesia has been clarified, the authors suggest that synergistic administration of amnestic medications be administered if recall is unwanted.
Periprocedural Applications of Dexmedetomidine
A summary of the current adult and pediatric periprocedural applications of DEX is provided in Table 13.1.
Table 13.1
Current common periprocedural applications of dexmedetomidine in adults and children
Pre-procedural applications | Advantage |
---|---|
Anxiolysis | Easy and quick arousal from sedation Minimal respiratory depression Attenuates sympathetic hemodynamic response |
Periprocedural applications | |
Airway procedures Rigid bronchoscopy Drug-induced sleep endoscopy MRI sleep studies Open thyroplasty Anterior mediastinal mass biopsy | Obtunds airway reflexes while maintaining stable hemodynamic and respiratory profiles in spontaneously ventilating children and provides sedative properties paralleling natural sleep |
Neurosurgical procedures | |
Posterior spine fusions | Lowers propofol and inhalational agent requirements and facilitates intraoperative wake-up tests |
Brain tumor and epileptic seizure foci resection | Preserves epileptiform activity and allows comfortable and cooperative sedation |
Cardiac surgery | Blunts sympathetic response, provides analgesia and sedation postoperatively, and expedites extubation |
Invasive procedures | |
Extracorporeal shock wave lithotripsy Burn dressing change Lumbar puncture Bone marrow biopsy Central venous line placement Chest tube insertion | Combining ketamine and dexmedetomidine in these procedures provides sedation, analgesia, amnesia, and hemodynamic stability |
Vascular surgery Carotid endarterectomy Carotid angioplasty and stenting | Provides excellent hemodynamics due to its sympatholytic effects |
Bariatric surgery | Potentiates opioid analgesia with minimal additional respiratory depression |
Post-procedural applications | |
Adenotonsillectomy Postoperative shivering Postoperative emergence agitation | May reduce the incidence of severe emergence agitation, opioid requirements, and episodes of oxygen desaturation in children with obstructive sleep apnea |
Sedation in the pediatric intensive care | Produces a state of cooperative sedation associated with minimal respiratory depression and facilitates withdrawal from benzodiazepines or opioids |
Miscellaneous applications | |
Palliative care Treatment of cyclic vomiting syndrome | May provide a bridge to wean the patients’ dependency on opioids |
Pre-procedural Applications: Anxiolysis
Premedication with DEX not only offers anxiolysis , sedation, and analgesia but also helps in attenuating the stress responses to tracheal intubation/extubation and emergence from anesthesia. DEX administration has been described as a premedication by intramuscular, IN [30], and buccal route [31]. The buccal route ensures more compliance and better absorption, while the intranasal technique causes no discomfort during administration and is relatively quick and simple. The nasal route is effective and well tolerated for sedation and postoperative analgesia in adults in the dose of 1 mcg/kg given 45 min before surgery [104].
When used as a premedication in pediatric patients, IN DEX has been shown to produce more satisfactory effect of sedation than buccal DEX (1 mcg/kg) or oral midazolam (0.5 mg/kg) [31, 105, 106]. Compared to IN dose of DEX (1 mcg/kg), higher IN doses of DEX (2 mcg/kg over 1 mcg/kg) are more efficacious at producing sedation, anxiolysis, better acceptance to a mask inhalation induction, and less cardiovascular variability [30].
A recent study that examined the onset time of IN DEX premedication (1 mcg/kg) in 100 healthy children aged 1–12 years undergoing elective surgery showed that the median onset time of sedation was 25 min and the median duration was 85 min [107]. At a higher dose, 2 mcg/kg IN DEX (compared to 0.5 mg oral midazolam) produced shorter onset of sedation without a demonstrable difference in conditions at induction, emergence, and recovery [108]. In a prospective, randomized, open-label clinical trial, children were enrolled into one of the three groups of premedication: midazolam 0.5 mg/kg PO, clonidine 4 mcg/kg PO, or transmucosal DEX 1 mcg/kg. All groups produced similar anxiolysis, ease of separation from parents, recovery, and discharge time. DEX had advantages (compared to midazolam) with respect to analgesia and attenuation of sympathetic hemodynamic response [109].
A recent meta-analysis of 13 randomized controlled trials (RCTs ) on non-intravenous (IN, sublingual, or oral) DEX versus midazolam was performed to examine the efficacy in improving perioperative sedation and analgesia and in reducing postoperative agitation when used as a preanesthetic medication in 1033 children. The authors concluded that DEX as a premedication is superior to midazolam in ensuring satisfactory levels of sedation in children undergoing surgery, both at separation from parents and at emergence [110].
DEX that is characterized by an easy and quick arousal from sedation resembling natural sleep makes it theoretically a promising premedication. On the other hand its slow onset of anxiolysis makes it an unsuitable substitute for oral midazolam [111]. The current major limitations of studies examining efficacy of DEX in improving perioperative sedation are the significant heterogeneity between studies in the scales and measures used for sedation and children’s anxiety evaluation, differences in the anesthesia protocols, and differences in the doses. More studies are needed to evaluate the effect of premedication routes on various outcome measures like preoperative anxiety levels, induction time, emergence excitation, postoperative analgesic requirements, and postoperative behavior disturbances. Randomized clinical trials are also required to identify optimal doses and appropriate monitoring of DEX use for premedication.
Intra-procedural Applications
Airway Procedures
Tracheal intubation withou t the use of muscle relaxants is commonly used in pediatric anesthesia. A single dose of DEX (1 mcg/kg) has been shown to improve intubation conditions in children after induction with propofol (3 mg/kg) and remifentanil (2 mcg/kg) without muscle relaxants in 60 ASA physical status I children aged 5–10 years [112]. DEX did not affect the hemodynamic response to intubation. IV administration of DEX (1 mcg/kg) has also been found to decrease the target effect-site concentration of propofol and remifentanil that is required for suspension laryngoscopy by 1.29 μg/ml and 0.64 ng/ml, respectively. Single-dose bolus injection of DEX (0.5 mcg/kg) before tracheal extubation has been shown to attenuate airway-circulatory reflexes during extubation [113].
Airway procedures such as rigid bronchoscopy are challenging and require meticulous anesthesia management including airway access for the surgeons, adequate oxygenation and gas exchange to avoid hypoxemia, and stabilized hemodynamics. Total intravenous propofol anesthesia, with or without remifentanil , is a common technique used in these procedures. Challenges with this technique include the ability to maintain spontaneous respiration, protect the airway, and prevent laryngospasm. Comparatively, DEX offers some advantages: In contrast to other agents, DEX converges on sleep pathways at the locus ceruleus and is associated with changes in neuronal activity similar to those seen in deeper stages of NREM sleep, without significant respiratory depression [114, 115]. The ability to maintain spontaneous ventilation and upper airway tone makes DEX an attractive choice for this scenario, particularly for children with severe preoperative airway impairment [40, 45]. Previous reports have demonstrated that for rigid bronchoscopy, DEX offers advantages of obtunding airway reflexes while maintaining stable hemodynamic and respiratory profiles in spontaneously ventilating children [116–118].
Open thyroplasty with vocal cord medialization is another challenging airway surgery performed to treat dysphonia. To optimize surgical repair, the patients need to be awake or lightly sedated during the procedure. The anesthesia challenges require that the non-intubated patient has adequate anxiolysis, sedation, and analgesia while maintaining the ability to phonate on command. In combination with local anesthetic, DEX has been described for this case scenario to maintain spontaneous ventilation and patient cooperation during laryngoplasty [119]. The favorable physiological effects of DEX on airway in addition to the antisialagogic [120] effect make it a useful anesthetic choice in adults and children who have a potential for airway catastrophe such as those with history of known difficult airway. Incorporating and expanding the applications of DEX into appropriate airway surgeries will be invaluable, as it has already been successfully implemented for patients at risk of airway collapse or a difficult airway [121–123]. By maintaining spontaneous ventilation and avoiding respiratory depression, DEX has been useful for those at risk of suffering fatal cardiopulmonary, respiratory, and cardiovascular complications [124].
Dexmedetomidine Use During Neurosurgical Procedures
The addition of DEX to anesthetic regimen is desirable in neurosurgical procedures and may contribute significantly to patient comfort and safety. A recent randomized trial evaluated the opioid-sparing effect of an intraoperative DEX infusion (0.2–0.5 mcg/kg/h) after craniotomy and demonstrated the opioid-sparing effect of DEX as evidenced by (1) a reduced verbal numerical pain rating scale, (2) a prolonged time before an analgesic request, (3) a reduced opioid requirement to control postoperative pain, and (4) fewer opioid-related side effects [125]. DEX has been used a as an adjunct to total intravenous anesthesia (TIVA) in the perioperative regimen used in the care of patients undergoing posterior spine fusion due to the anesthetic-sparing effects by lowering the propofol and inhalational agent requirements and facilitating emergence from anesthesia for the intraoperative wake-up test (when requested) and at the completion of surgery [63, 126]. It is also often continued into the postoperative period because of its ability to provide sedation and potentiate opioid analgesia with minimal additional respiratory depression. One should know that the potential risks of adding high doses of DEX to the anesthesia regimen in neurosurgical procedures require motor evoked potentials (MEP) monitoring (Fig. 13.4). A target plasma concentration of 0.4 ng/ml DEX and 2.5 mcg/ml propofol seems to have minimal effect on MEP. Higher plasma serum DEX concentrations, however, may attenuate the amplitude of MEP [127]. DEX (0.2–0.7 mcg/kg/h) can also be used as an adjunct to establish and maintain controlled hypotension (mean arterial BP of 55–65 mmHg) during anterior spinal fusion [128]. Its concomitant effect, when used for controlled hypotension, on cardiac function, cerebral blood flow, and cerebral perfusion pressure has not been carefully evaluated.
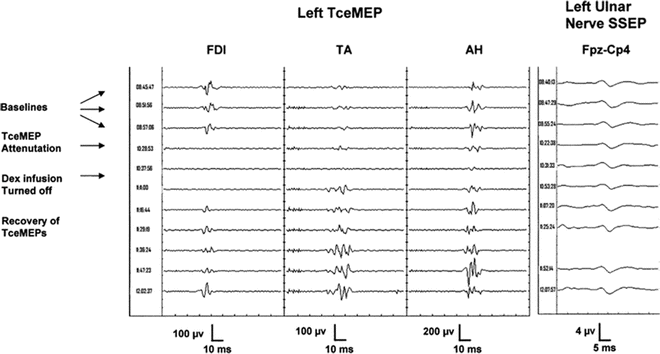
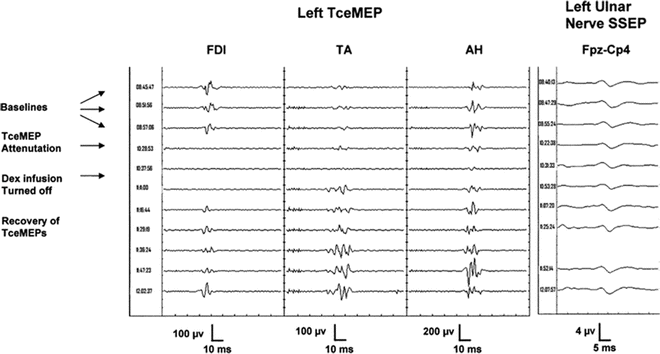
Fig. 13.4
Significant attenuation of transcranial electrical motor evoked potential amplitude after infusion of dexmedetomidine. Samples of transcranial electrical motor evoked potentials (TceMEP) recorded in a 13-year-old, 90-kg female patient with kyphoscoliosis presented for posterior spinal fusion with instrumentation of T2 to L3. Total intravenous anesthesia (TIVA) was initiated immediately after intubation with propofol 200 mcg/kg/min, remifentanil 0.5 mcg/kg/min, and dexmedetomidine 0.5 mcg/kg/h. Samples from left first dorsal interosseous (FDI), tibialis anterior (TA), and abductor hallucis (AH) muscles 1 h after starting TIVA (baselines), 1.5 h after acquisition of baselines (TceMEP attenuation), and during a 2-h period after the infusion of dexmedetomidine (DEX) were stopped (recovery of TceMEPs). Cortical somatosensory evoked potentials (SSEP) to stimulation of the left ulnar nerve recorded during the same time period as the TceMEPs are shown
Brain mapping and neurophysiologic testing have recently become an integral part of many neurosurgical techniques, brain tumor and epileptic seizure foci resection in particular. The anesthetic regimen for these tests should aim for a deep plane of anesthesia during the highly stimulating craniotomy and then a fully awake, comfortable, and cooperative patient during lesion resection in order to provide instant neurological feedback [129, 130]. Clinicians should also carefully balance the anesthetic depth in order to avoid untoward incidents such as airway obstruction, respiratory depression, hypercarbia, coughing, and hypotension. A key advantage of using DEX in these procedures is maintaining ventilation and airway patency in the presence of increasing level of sedation. Another critical advantage is ability to easily awaken patients by verbal stimulation. DEX 0.1–0.3 mcg/kg/h has been shown to maintain respiratory drive and airway patency while still enabling the patient to be awakened and responsive to verbal stimulation for functional brain mapping [131].
Common anesthetic agents (both inhalational and intravenous) that are used for general anesthesia are known to suppress brain electrical activity by stimulating the GABA-A receptors in the cerebral cortex. DEX acts on subcortical areas of the brain and does not bind to the GABA receptors. It preserves epileptiform activity in children with seizure disorders, facilitating localization and identification of seizure foci [115, 132]. In a recent prospective study, electrocardiogram was monitored during DEX infusion in 34 adult patients undergoing anterior temporal lobe resection with amygdalohippocampectomy for drug-resistant mesial temporal lobe epilepsy. The authors concluded the DEX is useful during intraoperative electrocorticogram recording in epilepsy surgery as it enhances or does not alter spike rate in most of the cases, without any major adverse effects [133].
Cardiac Procedures
Adult and pediatric cardiac surgery is associated with a high risk of cardiovascular and other complications that may lead to increased morbidity and mortality and prolonged hospital stays. Alpha-2 agonists have many desirable effects, including analgesia, anxiolysis, inhibition of central sympathetic outflow, and blunting of the sympathetic response, that improve hemodynamic stability, positively affect myocardial oxygen supply and demand, may provide myocardial protection, and maximize neurocognitive function and expedite extubation [134–136]. Administration of DEX prior to global ischemia/reperfusion resulted in a reduction in infarct size, which was abrogated by pretreatment with yohimbine. This indicates that DEX exerts a cardioprotective effect against ischemia/reperfusion injury [137]. Several studies also suggested that DEX has a protective effect against myocardial ischemia injury in animals. These effects are not centrally mediated; it may result from preventing an increase in myocardial norepinephrine level in the ischemic region through cardiac presynaptic alpha-2 adrenoreceptor stimulation [138, 139]. In contrast to the previous studies, a recent randomized controlled trial found DEX did not provide cardioprotection in coronary artery bypass grafting with cardiopulmonary bypass [140].
The cardioprotective effects of DEX are based on blunting hemodynamic responses to perioperative stress, i.e., controlling HR through centrally mediated sympatholysis [141]. Under conditions of regional ischemia, DEX has been shown to exhibit cardioprotective effects: it optimized the blood flow of coronary arteries [142] and decreased lactate release during emergence from general anesthesia [143]. As an adjunct to anesthetic induction, DEX blunts the hemodynamic response to endotracheal intubation in patients undergoing cardiac surgery [144, 145]. A retrospective study in adults showed that perioperative DEX use was associated with a decrease in postoperative mortality up to 1 year and reduced the risk of overall complications and delirium in patients undergoing cardiac surgery [146]. In children (1–6 years) undergoing cardiac surgery, DEX 0.5 mcg/kg IV followed by an infusion of 0.5 mcg/kg/h attenuates the hemodynamic and neuroendocrine (epinephrine, norepinephrine, blood glucose, plasma cortisol) responses at time of incision, time of sternotomy, and after bypass [147]. Whether there is a long-term benefit on reducing delirium and mortality in the pediatric population is yet to be determined.
Although the negative chronotropic effect of DEX is considered as an adverse event, it has been used as a therapeutic maneuver in various clinical scenarios. In a retrospective study, 13 (93 %) of 14 children who received DEX for atrial and junctional tachyarrhythmias were converted to normal sinus rhythm [148]. Another prospective cohort study of pediatric patients undergoing cardiothoracic surgery [149] found that perioperative use of DEX may reduce the incidence of both ventricular (0 % vs. 25 %) and supraventricular (6 % vs. 25 %) tachyarrhythmias without significant adverse effects. The same authors examined the potential efficacy of DEX in the acute treatment of AV nodal-dependent reentrant tachyarrhythmias in pediatric patients compared with adenosine. The authors showed that the administration of DEX (0.7 ± 0.3 mcg/kg) successfully terminated 26 episodes of supraventricular tachycardia (96 %) at a median time of 30 s (20–35 s) [31].
Bariatric Surgery
Worldwide, obesity in both children and adults continues to increase in most countries and to a greater extent in modern societies. As a result bariatric surgery continues to grow throughout the world. Perioperative management of these patients is more challenging than in nonobese patients because airway anatomy is often abnormal with excess pharyngeal tissue and tongue size making it difficult to ventilate and to intubate [150]. Respiratory comorbidities in these patients may profoundly impact the anesthetic management. These patients are at an increased risk of developing opioid-induced ventilatory depression. Because of the increased risks with the conventional opioid-based anesthesia, DEX was recently added as an adjunct to anesthetic regimen to potentiate opioid analgesia with minimal additional respiratory depression. A recent study showed that using DEX infusion (0.2–0.8 mcg/kg/h) during laparoscopic bariatric surgery decreased fentanyl use, antiemetic therapy, and the length of stay in the recovery room. However, it failed to facilitate late recovery (e.g., bowel function) or improve the patients’ overall quality of recovery [151]. Although the study did not facilitate late recovery, rapid turnover of these procedure and early recovery of these patients can be extremely important in busy practices.
Dexmedetomidine Applications During Vascular Surgeries
Perioperative management of hypertension is very important in patients who undergo carotid endarterectomy (CEA ). If not managed appropriately, the patients can have intracerebral bleed, left ventricular failure, myocardial infarction, or fatal arrhythmias. The sympatholytic effect and the hemodynamic stability provided by DEX make it an attractive choice in these procedures [152]. A recent study recruited 54 patients for CEA under regional anesthesia and enrolled 25 patients in the DEX group and 29 patients in the standard group (midazolam and fentanyl). Patients in the DEX group received 0.5 mcg/kg bolus over 10 min followed by infusion of 0.2 mcg/kg/h. DEX provided less intraoperative and postoperative hypertension and tachycardia. But the incidence of bradycardia and hypotension was also more in the DEX group [153]. In conjunction with local anesthesia, DEX provided adequate sedation for a patient for axillofemoral bypass graft in a patient with history of difficult airway [154].
Dexmedetomidine for Dental Procedures
DEX appears to provide the desirable properties for successful dental sedation than traditional sedatives because of its analgesic property, antisalivatory properties , anxiolytic and sympatholytic effects, and less cognitive impairment and respiratory depression. It has been trialed for adult and pediatric dental sedation via different routes [155–161]. Along with its beneficial effects, DEX was reported to exert potential anti-inflammatory and antioxidant effects. Previous studies revealed that DEX significantly decreased the levels of inflammatory cytokines [162]. Compared to midazolam, DEX appears to provide better sedation and postoperative analgesia via anti-inflammatory and antioxidation pathway during office-based artificial tooth implantation [163]. A recent study that compared the efficacy (sedation, anxiolysis, analgesia, operating conditions, and patients’ satisfaction) and safety of DEX and midazolam as sedatives for dental outpatient procedures showed that DEX works, as well as midazolam, for outpatient dental procedures and can be used as an alternative to midazolam [164]. Conversely, a Japanese study compared intravenous sedation with DEX and propofol for minor oral surgery and reported hypotension and bradycardia with DEX but no difference in respiratory depression with propofol [165].
Although the bioavailability of DEX is poor via the oral route, a prospective, triple-blind, randomized study compared the efficacy and safety of one of the three doses of oral DEX (3, 4, and 5 mcg/kg) combined with ketamine (8 mg/kg) for pediatric dental sedation. The study showed that ketamine and DEX at 5 mcg/kg provided faster onset of sedation, higher intra- and postoperative analgesia and anterograde amnesia, and delayed recovery from sedation when compared with lower dose of DEX groups [166].
Regional Anesthesia
Clonidine has been employed clinically to achieve the desired effects in regional anesthesia (epidural, intrathecal, peripheral nerve block) for decades [167]. The successful use of epidural clonidine in adults led to its evaluation in pediatric caudal block, and so far there is reasonable extensive clinical experience and published literature to dispel any concern regarding its neurotoxicity. DEX has been described with regional blocks in both adults and children. In adults, a meta-analysis of 16 RCTs included 1092 adults and compared outcomes between DEX (intrathecal, epidural, or caudal) and bupivacaine or ropivicaine. DEX was found to decrease the pain and prolong the analgesia. Although there was an increased incidence of bradycardia in the DEX group, it was not associated with hypotension and did not warrant treatment [168]. Similarly, the combination of caudal DEX and bupivacaine (1 mcg/kg and 2.5 mg/kg, respectively) in children has been shown to decrease the sevoflurane requirements, incidence of emergence agitation, adjuvant postoperative analgesics, and duration of postoperative pain relief, to a greater degree as compared to bupivacaine alone. The addition of caudal DEX to bupivacaine did not affect the hemodynamic response [169]. Epidural DEX and clonidine produced similar analgesia, duration of action, and hemodynamic profile when used with bupivacaine (2.5 mg/kg) for lower abdominal surgery in children [170]. A recent meta-analysis concluded that the addition of DEX to a caudal anesthetic provided extended duration of postoperative pain relief in 328 pediatric patients. There was no statistically significant effect on hemodynamics and adverse events with the addition of DEX to the local anesthetic. Subgroup analysis showed no advantage of the 2 mcg/kg of caudal DEX over the 1 mcg/kg in terms of analgesia [171]. Currently there are still some concerns regarding neuraxial DEX safety. DEX via the neuraxial route is off label and the safety has not been established in humans. In animal models, perineural administration of DEX attenuated inflammation in the sciatic nerve via reducing inflammatory cytokine levels [172]. However, in rabbits, epidural DEX elicited what appeared to be a demyelination of oligodendrocytes in the white matter of the spinal cord [173]. Future studies are warranted in order to support or dispel the concerns of neurotoxicity from DEX.
Caution should be exercised when DEX sedation is used in infants and neonates receiving epidural analgesia, without support from external warming devices. Infants depend more on non-shivering thermogenesis than on shivering and vasoconstriction. In mice, DEX causes hypothermia [174]. The effect is attenuated in animals that are genetically deficient in alpha-2 adrenoceptor. The hypothermic response is postulated to interference with non-shivering thermogenesis and reduction of metabolic heat production [175]. Recently a 2-day-old neonate receiving epidural analgesia, without using external warming devices, developed hypothermia (33 °C axillary) and bradycardia (HR 75 beats/min). There was no change in the HR following the administration of atropine and naloxone that was unresponsive to atropine [176]. The epidural infusion was discontinued and the infant was placed under a radiant warmer. Over the next 2–3 h, the temperature was improved.
Dexmedetomidine Applications for Ambulatory Procedures
Clear understanding of the pharmacokinetic effects of the chosen anesthetic agent is critical for brief procedures in the operating room. An important factor influencing the anesthetic regimen in ambulatory practice is rapid home discharge. There are few studies that examined the use of DEX for ambulatory procedures, perhaps because its half-life and analgesic properties do not lend themselves to the fast pace (induction, emergence, and recovery) of most short procedures. The undesirable prolongation of recovery length of stay associated with administering high dose of DEX for brief procedure makes it a poor choice that does not appear to offer advantages over current standard of practices.
Pressure equalizing tubes procedure is a very common brief (10–15 min) surgical procedure in the pediatric population. Some form of analgesia is required in most children despite the brief nature of the procedure with limited tissue trauma. Management of pain can be a challenge because this procedure is often performed without intravenous access; the options for providing analgesia may be limited. Choosing nasal DEX administration for this procedure is theoretically reasonable because it has been shown to have analgesic effects without significant respiratory depression. DEX administration has been described for this procedure, but without an advantage over intranasal fentanyl or acetaminophen [177]. Early experience with this agent demonstrated it to be ineffective during upper gastrointestinal endoscopy in children; it was not found to offer any advantages either alone or in combination with propofol [178].
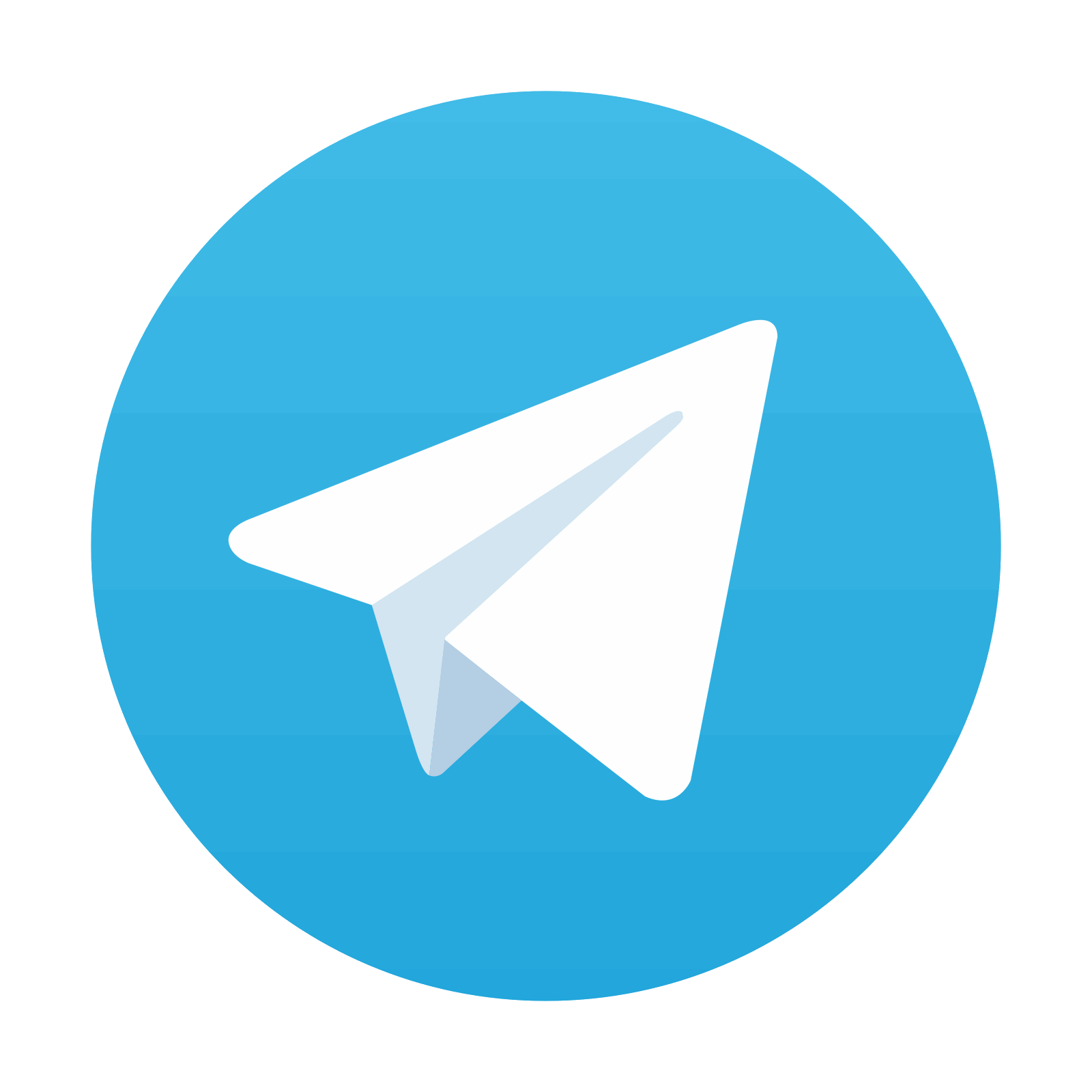
Stay updated, free articles. Join our Telegram channel

Full access? Get Clinical Tree
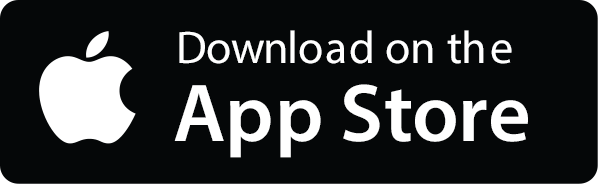
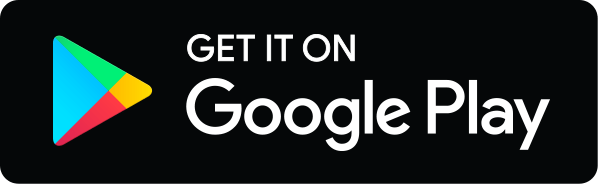