KEY POINTS
1. Mechanical circulatory support devices most often are ventricular assist devices, which are indicated for bridging to myocardial recovery or cardiac transplantation or as a permanent assist device (destination therapy).
2. The principal growth area for ventricular assist devices in recent years has been destination therapy. The predominant device used for this purpose has changed from the pulsatile, bulky Heartmate XVE to the compact nonpulsatile (continuous flow) Heartmate II device.
3. In New York Heart Association Class IV Heart Failure (minimal activity induces symptoms), both the Heartmate XVE and Heartmate II provide better survival and quality of life than medical therapy, but Heartmate II outcomes are superior to those for Heartmate XVE.
4. A variety of nonpulsatile right ventricular assist devices (RVAD) and a pulsatile total artificial heart are Food and Drug Administration-approved for bridging to recovery or to transplantation. RVADs are sometimes needed as a bridge to RV recovery for patients undergoing destination LVAD placement.
5. Newer short-term bridging VADs include the CentriMag, Tandem Heart, and Impella pumps, all of which are continuous-flow pumps that implant and operate in separate and distinct ways.
6. Placement of a destination LVAD most often requires a median sternotomy and cardiopulmonary bypass. Anesthetic management is similar to that for orthotopic cardiac transplantation in that it requires recognition that preoperative LV systolic function is abysmal.
7. Separation from CPB after LVAD placement is often complicated by either primary or secondary RV failure, which may require various combinations of pulmonary arterial vasodilators (e.g., milrinone, nitric oxide), inotropes (e.g., dobutamine, epinephrine), and systemic arterial vasoconstrictors (e.g., vasopressin).
8. Transesophageal echocardiography is essential to VAD placement for its assessment of biventricular preload, valve function, RV function, presence or absence of a patent foramen ovale, and VAD inflow and outflow. Aortic valve insufficiency is particularly troublesome for LVAD patients.
9. Early postoperative problems include bleeding and thromboembolism. Late postoperative problems include device malfunction and infection, especially for pulsatile VADs.
10. Continuous-flow VAD patients presenting for noncardiac surgery pose a variety of clinical problems, which may include obtaining accurate measurements of blood pressure, VAD blood flow, and pulse oximetry as well as acquired von Willebrand’s syndrome and aortic valve insufficiency.
11. Intra-aortic balloon pumps (IABPs) are most often used to provide temporary LV support to patients undergoing interventional cardiology or cardiac surgical procedures. IABPs augment diastolic blood flow to reduce LV afterload, while simultaneously potentially increasing coronary artery blood flow.
12. IABP inflation and deflation must be synchronized to the cardiac cycle, which can be done using either electrocardiography or an intra-arterial waveform. Inflation should commence just after systole ends, and deflation should end just before systole begins.
13. The most common serious IABP complication is lower limb ischemia.
I. Introduction
Aside from the intra-aortic balloon pump (IABP), the use of a mechanical circulatory support device (MCSD) has historically been relegated to “salvage” therapy. Although a selective role continues for this type of intervention, MCSDs are taking their place as part of a therapeutic continuum that can be successfully used to treat a multitude of cardiovascular problems. Indeed, many therapeutic interventions using MCSDs are performed on an elective or urgent basis rather than as a life-saving emergency. Most current devices and devices in development are designed for long-term outpatient therapy with excellent quality of life.
Devices are getting smaller, easier to implant, and more durable. The potential growth for this emerging therapy is quite substantial. This chapter will discuss the current state of MCSDs, future directions, and also will review the most common circulatory assist device, the IABP.
II. History
Dr. Michael DeBakey performed the first successful clinical implant of a ventricular assist device (VAD) for postcardiotomy cardiac failure in 1966 [1]. The patient was mechanically supported for 4 days. The first successful use of MCSD for a “bridge” to transplantation occurred in 1978 at the Texas Heart Institute. That patient was supported for 5 days by a pneumatically actuated paracorporeal device. The first implant of a total artificial heart (TAH) was performed on Dr. Barney Clarke, a retired dentist, by Dr. William DeVries in 1982 at the University of Utah. The pump was a Jarvik TAH designed by Dr. Robert Jarvik. Dr. Clarke lived for 112 days; unfortunately, the world watched him slowly and courageously die over the ensuing months from sepsis and embolic events. As with the pioneering experience with cardiac transplantation in the 1960s, there was public criticism of TAH as an “advancement” that was not worthwhile. Nevertheless, development of more sophisticated devices for short-term and long-term use quietly persisted. Successful recovery of patients in acute cardiogenic shock using short-term VADs occurred and the data improved. The first pump to receive Food and Drug Administration (FDA) approval in the United States was the Abiomed BVS 5000 (Abiomed, Danvers, MA, USA) in November, 1992. Two implantable pumps were then approved for inpatient long-term use, the Heartmate IP system (Thoratec Corp., Pleasanton, CA, USA) and the Novacor system (Worldheart, Ontario, Canada). In 1995, both the Heartmate Vented Electric (VE) system and the Novacor system were subsequently approved for outpatient use. This was followed by the Thoratec VAD system (Thoratec Corp., Pleasanton, CA, USA), which was approved as a long-term support system in 1996. The TAH also quietly evolved during this time. There were three different systems that developed with the support of the National Institutes of Health (NIH): (a) The Penn State TAH (Hershey, PA, USA; Minnesota Mining and Manufacturing, Minneapolis, MN, USA); (b) the Abiomed AbioCor TAH (Abiomed, Danvers, MA, USA); (c) the Cardiowest TAH (Syncardia, Tucson, AZ, USA). The Penn State TAH was purchased by Abiomed and is being developed as the AbioCor II. AbioCor I (the original AbioCor) was implanted in 14 patients as a Phase I clinical trial for destination (i.e., permanent) therapy. It was approved for clinical use by the FDA in September, 2006. The Cardiowest TAH represented the evolution of the Jarvik TAH and has had over 1,000 implants worldwide. It received FDA approval as a bridge to transplantation in 2005.
The period of 2007 to 2012 has marked an exciting and exponential rise in the use of newer, smaller rotary and centrifugal left ventricular assist devices (LVADs). The Heartmate II (HM II) LVAD (Thoratec Corp., Pleasanton, CA, USA) received FDA approval for bridge to transplantation in 2007 and destination therapy in 2009. The Jarvik rotary LVAD (Jarvik Heart, Inc., New York, NY, USA) remains in clinical trials, but offers implantation through a left thoracotomy, which may be advantageous in certain circumstances. The Heartware HVAD (HeartWare International, Framingham, MA, USA) is a centrifugal pump which has the advantage of implantation directly into the LV apex without requiring an additional extrapericardial pocket. Since an additional pump pocket is not required, there will likely be fewer infectious complications and faster implant times for this pump when compared with contemporary rotary pumps. In addition, the HVAD is the first long-term centrifugal pump to be tested in clinical trials.
III. Indications
There are three commonly described indications for the use of MCSDs: Bridge to myocardial or hemodynamic recovery, bridge to cardiac transplantation, and alternative to cardiac transplant or “destination therapy.”
A. Bridge to recovery. The use of an MCSD as a recovery system has focused on short-term use. Traditionally, this has been thought of as support for days to weeks. Clinical indications for this use include cardiogenic shock in the following settings:
1. Postcardiotomy
2. Acute myocardial infarction (MI)
3. Viral cardiomyopathies
4. Inadequate preservation of donor heart after cardiac transplantation
1
5. Other causes
The postcardiotomy use of these pumps has declined over the past several years [2]. This likely derives from improved perfusion and preservation techniques. Nevertheless, in this patient population the anticipated recovery time was 3 to 5 days. Animal data for support to recovery supported this timeline, as recovery was seen quite early [3]. We now recognize that there may be substantial variability in the time it may take for patients’ hearts to recover while on support. This is likely to be diagnosis specific. Data from the Abiomed voluntary registry showed that mean support time to successful recovery may exceed 30 days [2]. Several devices are now employed as short-term bridge-to-recovery devices: The Abiomed system, the Centrimag (centrifugal system, Thoratec Corp., Pleasanton, CA, USA), the Impella 2.5 or 5.0 and the Tandem Heart (CardiacAssist Inc., Pittsburgh, PA, USA), and lastly conventional veno- arterial extracorporeal membrane oxygenation (ECMO). The goal of short-term bridge-to-recovery device is to provide end-organ perfusion while the heart is recovering. These short-term devices are especially useful in catastrophic, sudden presentations of cardiogenic shock (e.g., prolonged cardiac arrest during high-risk percutaneous coronary interventions) situations in which the likelihood of intact neurologic recovery remains unknown. These devices allow time to determine whether an irreversible neurologic injury would preclude a successful recovery or transplantation.
B. Bridge to transplantation. The use of MCSDs as a bridge to cardiac transplantation constituted the early human laboratory to understand the long-term effects of support on many physiologic parameters as well as on quality-of-life measures. The therapy was instituted in individuals who were listed for cardiac transplantation, but failing conventional or inotropic therapy. The MCSD would be employed to support the patient and improve the physiologic condition until a donor heart became available. The MCSD would then be explanted at the time of cardiac transplantation.
Many of the VADs (Thoratec IVAD [implantable VAD]/PVAD [paracorporeal VAD], Heartmate XVE, Novacor) or the Cardiowest TAH are approved for this indication. The Thoratec HM II pump (Fig. 22.1) has become the mainstay of pumps implanted for a bridge-to-transplantation indication. However, a randomized clinical trial comparing the HM II LVAD to the Heartware HVAD has recently been completed and the data submitted to the FDA seeking a bridge-to-transplantation indication for the Heartware HVAD [4,5].
Figure 22.1 Diagram of a patient with an implanted HM II continuous-flow LVAD including driveline and portable power source. (Reproduced with permission from Thoratec, Inc., Pleasanton, CA, USA.)
2
C. Destination therapy. As experience with long-term use of MCSD led to successful outpatient use of this therapy, the concept of using MCSD as another option for patients with end-stage heart failure who were not transplant candidates emerged.
A landmark study called Randomized Evaluation of Mechanical Assistance in the Treatment of Congestive Heart failure (REMATCH) concluded that end-stage heart-failure patients who received a Thoratec Heartmate VE LVAD experienced improved survival and quality of life when compared to optimal medical therapy. The use of MCSD as permanent support for end-stage heart failure is now an accepted and viable therapy that is also supported by the Center for Medicare Services (CMS). The Heartmate XVE was the first LVAD approved for this indication. Although the Heartmate XVE provided superior outcomes to medical therapy, this device was found to only support patients for approximately 2 yrs before the device failed. In a subsequent randomized trial comparing the Heartmate XVE with the HM II LVAD (continuous-flow pump), the HM II LVAD proved superior to the Heartmate XVE, with 2-yr survival in the HM II cohort of 58% versus 25% in the XVE group. The HM II received FDA approval for destination therapy in 2009 and the most recent Interagency Registry for Mechanically Assisted Circulatory Support (INTERMACS) database documented that more than 99% of destination therapy implants in 2010 were continuous-flow pumps [6].
3
IV. Classification and attachment sites of VADs
VADs can be used as an isolated LVAD, an isolated right ventricular assist device (RVAD), or as a biventricular assist device (BIVAD). These devices are attached to the heart through cannulae that allow the blood to enter the pumping chamber from which it is ejected into the systemic or pulmonary circulation. TAHs differ from VADs in that a TAH removes all of the native ventricles and part of the atria. In contrast, if a BIVAD is implanted, the LV and RV remain in place, but the majority of the cardiac output emanates from the BIVAD.
A. LVAD. For left-sided circulatory support, all of the devices can be attached to the left ventricular (LV) apex as the inflow connection to the pumping chamber. The LV apex is the preferred site of LVAD attachment for most of the devices and is the only option for some devices (HM II LVAD and Heartware HVAD). Some devices can function through cannulation of the left atrium either directly or percutaneously through the interatrial septum. The left atrium is not the ideal cannulation site for long-term support as the left ventricle may then form large thrombi as a result of blood stasis [7]. In addition, flows through the LVAD are generally higher with LV versus LA cannulation for VAD inflow. For cardiogenic shock where short-term LVAD support is anticipated, left atrial cannulation is a reasonable option. The LVAD outflow graft is routinely anastomosed to the aorta, usually the ascending aorta (Fig. 22.2).
Figure 22.2 Cannulation configurations for the Thoratec VAD either as a BIVAD on the left and right sides (as shown in A and B) or left side (LVAD) only (shown in C). A shows right-atrial inflow to pulmonary artery outflow configuration for the RVAD. B shows an RV inflow to pulmonary artery outflow for the RVAD. A, B, and C all show the traditional LV inflow to aortic outflow configuration for the LVAD. (Reprinted with permission from Thoratec Corporation.)
4
B. RVAD. For right-sided support, either right atrial or right ventricular (RV) cannulation can be used successfully. Substantial thrombus formation in the RV does not appear to result from right atrial cannulation even in long-term support. The RVAD outflow graft is anastomosed to the pulmonary artery. VADs can be implanted without the support of cardiopulmonary bypass (CPB), but CPB is generally used to maintain patient stability [8].
C. BIVAD. A BIVAD (Fig. 22.3) consists of the simultaneous use of RVAD and LVAD with connections as noted earlier for the individual assist devices.
Figure 22.3 Illustration of the Centrimag continuous-flow VAD positioned as an RVAD with inflow from the right atrium and outflow into the pulmonary artery. Also pictured is an HM II LVAD with inflow from the LV apex and outflow into the ascending aorta. (Reproduced with permission from Thoratec, Inc., Pleasanton, CA, USA.)
D. TAH. Placement of a TAH requires excision of the native heart. The current systems require removal of the ventricles, preserving the atria for securing the device. Sewing cuffs connect the TAH pumping chambers to the atria. Both of the current clinical systems have valves in both inlet (atrioventricular) and outlet (aortic or pulmonary) positions. Separate graft conduits connect the two pumping chambers to the aorta and main pulmonary artery. Once these grafts and cuffs are sewn, pressurized saline is used to test for leaks.
V. Mechanical assistance systems
A variety of mechanical support systems are used to treat patients with end-stage heart failure. However, there are only two distinct methods of blood flow delivery by these assist devices, which are essentially pumps. One method provides a constant flow to the patient’s circulatory system through a high-speed rotor pump. The other method provides pulsatile flow generated by a volume displacement pump (unsynchronized with the patient’s native heartbeat). Although the implantation of these devices is identical, there are differences in how the two systems fill and empty the heart and in the afterload the heart will experience. These differences may influence the right side of the heart (in the case of isolated LVADs) as well as end-organ function (e.g., brain, kidneys, liver). Both types of pumping systems may be used for short-term or long-term support.
A. Pulsatile. Pulsatile pumps are generally volume displacement pumps that work like the native heart. These are often called first-generation pumps. A chamber fills until a set volume is achieved, and this volume of blood is ejected into the aorta. Pulsatile pumps all have inflow and outflow valves mimicking the native mitral and aortic valves. The method of energy delivery used to eject the blood from the chamber may be air (pneumatic), fluid (hydraulic), or a mechanical pusher plate.
1. Short-term support. The Abiomed BVS 5000/AB ventricle system serves as an example of a pulsatile pump used for short-term support. These pumps can be used for single ventricle support of either the right or left side as well as for biventricular support (using two pumps). They are easily implanted and require minimal setup to initiate support. This “plug and play” implantability as well as relatively low cost has kept them in most institutions as the emergency support system. Recently, there has been a shift to using the Levitronix Centrimag system. This system is also an extracorporeal VAD; however, in contradistinction to the ABIOMED system, the Centrimag provides nonpulsatile continuous flow. The Thoratec PVAD has also been FDA-approved for potential short-term recovery, but it is more expensive and more complex to initiate. Its advantages are an excellent safety profile and durability. It is also approved for long-term outpatient use, should the patient require bridge to transplantation.
2. Long-term support. Five systems are currently FDA-approved for bridge to transplantation: Thoratec PVAD/IVAD, Thoratec Heartmate XVE, Thoratec HM II LVAD, Worldheart Novacor System, and Syncardia Cardiowest TAH. The Heartmate XVE and HM II LVAD are also the only devices that are currently FDA-approved for destination therapy, although other devices, notably the Heartware HVAD, are undergoing clinical trials for this indication.
B. Continuous flow. Continuous-flow devices come in different variations: Axial flow or centrifugal flow, and with different bearings: Mechanical (ball bearing, ruby bearing, fluid bearing) or magnetic (mag-lev or bearingless). Continuous-flow devices with bearings are considered “second-generation” devices, whereas the mag-lev pumps are considered “third-generation” devices.
5
1. Short-term support. Three continuous-flow pumps are currently being utilized in the United States as short-term support devices: CentriMag (Levitronix, Waltham, MA, USA), the Tandem Heart (CardiacAssist, Inc., Pittsburgh, PA, USA), and Impella (Abiomed, Danvers, MA, USA). The Centrimag pump (Fig. 22.3) is most commonly utilized with an open chest implantation with left atrial or LV inflow cannulation for the LVAD, and ascending aortic cannulation for outflow. The right atrium or right ventricle can be cannulated for inflow for an RVAD and the pulmonary artery as outflow for an RVAD. The Tandem Heart pump is attractive as a percutaneous LVAD. The inflow is placed via the right common femoral vein, across the intra-atrial septum into the left atrium. The outflow is in the descending thoracic aorta via a cannula placed in the femoral artery. The Impella pump is placed percutaneously via the common femoral artery retrograde across the aortic valve into the left ventricle. Inflow is through the left ventricle and outflow is into the ascending aorta—the VAD is placed across the aortic valve.
2. Long-term support. The Thoratec HM II LVAD was FDA-approved for bridge to transplantation in 2008. The HM II received FDA approval in January 2010 for use as destination therapy. The ENDURANCE trial is currently in progress and is a randomized clinical trial comparing Heartware HVAD to the HM II LVAD for destination therapy.
3. Advantages and disadvantages. Continuous-flow devices offer the advantage of being much smaller than their pulsatile counterparts, as no “blood chamber” is needed. Their smaller size often leads to easier implantation with less intraoperative dissection. This can reduce blood loss, shorten operative times, reduce infection rates, and provide the potential for more rapid recovery. The data generated from the outcomes with utilization of continuous-flow devices as a bridge-to-transplant indication show excellent 1-yr survival, approaching 90%. The 2-yr survival for the cohort that received the HM II for destination therapy was 58%. This was double the survival in the cohort that received the XVE.
Continuous-flow devices pose inherent challenges. These devices do not have valves and need to flow a minimum rate to prevent “device insufficiency.” This regurgitant flow can be catastrophic if power to the device is suddenly turned off. In addition, as patients are managed with longer-term nonpulsatile devices, certain complications that are device related have emerged, The HM II LVAD universally cleaves von-Willebrand’s factor (VWF). Nearly all patients with a HM II LVAD acquire a deficiency in VWF and are prone to mucosal bleeding events—particularly in the gastrointestinal tract [9]. Ongoing research into this phenomenon will hopefully gain more insight into who is at risk and how to optimally manage patients with bleeding. For the present, it appears likely that these patients would respond favorably to a desmopressin bolus (30 mcg/kg). Additionally, many patients have minimal arterial pulsatility when on full support. This is not generally problematic when arterial pressure is monitored with an indwelling arterial catheter, but may become so when the patient leaves the intensive care unit (ICU) or leaves the hospital for home or an extended care facility. In those settings, if a patient with a continuous-flow LVAD were found unresponsive, it would be challenging for the first responder to determine whether “circulation” is present or not.
VI. Intraoperative considerations
A. Anesthetic considerations
1. Monitoring and vascular access. Large-bore vascular access is required because blood loss and coagulopathy can be substantial. This can be achieved with one large-bore (16 gauge or larger) peripheral intravenous catheter and a large-lumen (e.g., 9 Fr) central-access introducer or with its equivalent via central venous access (e.g., a pulmonary artery catheter introducer with additional double-lumen large-bore integral ports). Standard American Society of Anesthesiologists monitors (electrocardiography [ECG], pulse oximetry, temperature) are used with the possible exception of a noninvasive blood pressure (BP) cuff. As noted earlier, an arterial catheter is essential and consideration might be given to its placement in a larger central artery such as the femoral artery, in order to minimize the potential problem of central-to-peripheral BP discrepancies at separation from CPB or thereafter. Pulmonary artery catheters can be very helpful for the assessment of RV function and pulmonary vascular resistance, but they may be mechanically infeasible after initiation of therapy with RVAD, BIVAD, or TAH. Intraoperative transesophageal echocardiography is essential for the assessment of aortic valve competency, patent foramen ovale (PFO), LV thrombus, adequacy of VAD inflow and outflow, cardiac preload assessment, and RV function (for isolated LVADs).
6
2. Anesthetic techniques. General endotracheal anesthesia is used, and most practitioners probably place emphasis on intravenous techniques that minimize hemodynamic impact. For example, a total intravenous technique using an opioid (e.g., fentanyl or sufentanil), a benzodiazepine such as midazolam, and a muscle relaxant, such as vecuronium or rocuronium may be selected. If renal or hepatic function is markedly impaired, cisatracurium can be a wise choice. In most centers, there is no advantage to attempting a “fast-track” approach, because these patients will generally remain intubated overnight or longer regardless of the type of device implanted. This may change as experience grows and as the devices become less and less cumbersome to implant. Consequently, these cases present a good opportunity for medium- (e.g., fentanyl 20 to 40 μg/kg total dose) to high-dose opioids (e.g., fentanyl 50 to 75 μg/kg total dose) as a means to ensure hemodynamic stability without concern for early extubation. If hepatic function is compromised, medium or lower doses may be more appropriate. Volatile anesthetic agents such as desflurane, sevoflurane, and isoflurane can be used as hemodynamically tolerated. Nitrous oxide probably should be avoided because of its potential to increase pulmonary vascular resistance (PVR) and because of the high risk of intravascular air.
B. Surgical techniques
1. Cannulation techniques
a. VADs. Atrial cannulation is the most common approach to gain inflow for short-term support when using either a right- or left-sided VAD system. The atria are easily accessed and the low pressures in these chambers facilitate hemostasis upon decannulation when the heart has recovered. The right atrial appendage is used for right-sided support. For left-sided support, the interatrial groove at the junction of the right superior pulmonary vein is the most common site for cannulation. Other cannulation options for left-sided support include the dome of the left atrium (between the aorta and superior vena cava), the left atrial appendage, or through the apex of the LV. Cannulation of the LV apex for inflow minimizes blood stasis and provides the best decompression of the heart. However, decannulation and repair of this high-pressure chamber can be difficult and often requires placement back onto CPB. LV apex cannulation is highly recommended when a prosthetic mitral valve is present to maintain blood flow through the valve and prevent thrombus formation. The LV apex is the sole acceptable inflow cannulation site for most long-term LVADs (Heartmate XVE, HM II, Heartware HVAD, Micromed, Novacor). The ventricular chamber empties into a long tube attached to a coated Dacron vascular outflow graft that is sewn to the ascending aorta or the pulmonary artery.
b. TAH. For implantation of a TAH, excision of the native heart must be performed while preserving the atria. First, the surgeon attaches sewing cuffs that will connect the atria to the TAH pumping chambers, after which the surgeon sews grafts to both the aorta and the main pulmonary artery. After these grafts and cuffs are sewn, leak testing with pressurized saline (tinged with methylene blue) is used. Leak testing is imperative as the suture lines are often inaccessible if bleeding occurs once the TAH is in place. The TAH is then attached to the atrial cuffs followed by the arterial grafts.
C. Initiation of support for LVAD
7
1. Initial considerations. Techniques for the initiation of support are similar in all of the pulsatile systems. Ventilation is re-established and the heart is gradually filled. The assist device is manually actuated until adequate preload and afterload are established. If the ventricle(s) are not decompressed with the initiation of support, inflow obstruction (into the VAD) must be considered. A distended ventricle will not be unloaded and will not recover. Pulmonary edema may also develop as the blood backs up into the pulmonary vasculature. If appropriate inflow and outflow cannula placement and decompression of the LV are achieved, TEE should identify interventricular septal displacement toward the LV. This often compromises right heart function by impairing the septal component of RV contraction, and the physical distortion may also induce tricuspid regurgitation. Right-sided function may be further compromised by increased PVR caused by thromboxane A2 [10] and transfusion-induced cytokine activation [11] as a result of CPB.
8
2. Role of TEE. Use of TEE to separate from CPB is essential. TEE is used to assess the presence of air in the ventricle or ejecting from the LVAD into the ascending aorta. The chamber that has been cannulated for the inflow of the MCSD should be decompressed. If the chamber is full, and there is poor device flow, there is a technical issue that needs to be identified and corrected. In the case of an LVAD, the LV should be decompressed; if it is full, there is a technical impingement of flow into the pump (typically faulty inflow or outflow). If the LV is empty and there is poor flow through the VAD, then there is either inadequate RV preload or right heart failure (which may be primary or secondary to high PVR). As the LV is decompressed and global cardiac output increases, there may be some tolerable physiologic right heart dilation, which may at times be difficult to distinguish from right heart failure. The apical interventricular septum can occasionally deflect leftward and obstruct inflow into the LVAD. When this occurs, it is easily identified by TEE. TEE is also used to identify aortic insufficiency and the presence of intracardiac shunts, either of which may arise at the time of VAD support even if absent before that time.
3. Aortic insufficiency. Aortic valve insufficiency (AI) can be catastrophic, and TEE is essential to identify its presence or absence and severity. Even mild preoperative AI can be problematic since patients in cardiogenic shock will have a low mean arterial pressure (MAP) and a high LV end-diastolic pressure (LVEDP) to produce a relatively low aortic transvalvular diastolic gradient. With implementation of LVAD support, the LVEDP will become very low and the MAP will be higher, so the aortic transvalvular gradient (MAP–LVEDP) will be much greater. Mild preoperative AI, therefore, can become severe AI with LVAD support. This induces high LVAD flows as the insufficient valve fills the LV and subsequently the VAD. The resulting high LVAD flow rates are deceptive, because much of the flow is “circular” from LV to LVAD to ascending aorta and back via the incompetent aortic valve to the LV, so the net forward “perfusion” flow will be low. The aortic valve incompetence must be eliminated in this situation, but the approach is controversial depending on the indications for device implant and expected duration of support (valve replacement versus permanent valve closure) [12–14].
4. Intracardiac shunts: Quiescent intracardiac shunts may become clinically apparent and significant as a result of changes in chamber pressures when VAD support is initiated. A PFO is present in up to 20% of the population, but is clinically quiescent in the vast majority of these patients. Upon unloading the LV and left atrium with LVAD support, right atrial pressure will become higher than left atrial pressure. Under these circumstances, even a small PFO can produce a large right-to-left shunt manifested by arterial desaturation and potential paradoxical air embolism. These shunts should be closed when identified and should be assessed by TEE both before and after initiation of VAD support. If identified preoperatively, cannulation techniques for CPB may be altered to facilitate repair.
5. Pharmacologic support. Inotropic agents (e.g., dobutamine or epinephrine) are important to support the right heart when only left-sided support is used. Inhaled nitric oxide (20 to 40 ppm) may be invaluable in the early management of these patients because of its capacity to vasodilate the pulmonary vasculature without the systemic hypotensive effects seen with other pulmonary artery vasodilators. Alternative approaches include the use of a phosphodiesterase inhibitor (e.g., milrinone, which decreases pulmonary and systemic vascular resistances) or nesiritide usually in combination with a systemic vasoconstrictor such as vasopressin. Vasopressin offers an advantage over a-adrenergic agonists because it possesses minimal vasoconstrictive effects on the pulmonary arterial vasculature.
For a variety of reasons, systemic arterial vasodilation commonly occurs after initiation of LVAD support, so a need for a systemic vasoconstrictor is common. Experimental approaches to pulmonary vasodilation include inhaled nitroglycerin, inhaled prostacyclin, and sildenafil. Antiarrhythmics are often initiated before weaning from bypass onto device support as well.
D. Initiation of support for BIVAD
1. Initial considerations. When initiating biventricular support, the left system should be actuated first. Alternatively, both right and left systems may be actuated together. This prevents LV overdistention as well as pulmonary edema. Inotropic support can often be totally discontinued as the heart need not work. Biventricular support allows more complete resting of the heart and usually allows total decompression of the venous system as well. This minimizes hepatic and other end-organ congestion. The PA catheter should not be withdrawn as it will be difficult to reinsert it. Thermodilution cardiac outputs are not calculable when an RVAD is functioning.
2. Flow rates. The RVAD and LVAD should both have similar flow rates. The LVAD often has higher flow rates due to physiologic shunts (bronchial arterial return). RVAD flow that exceeds LVAD flow early after initiating BIVAD support is worrisome. This can occur with two common scenarios:
a. One or both left-sided cannulae are malpositioned to impede inflow to or from the device. If the obstruction is not corrected, the lungs can become flooded with the increased blood flow from the RVAD.
b. The LV is beginning to recover and is ejecting some blood over and above the LVAD flow. This can be identified by the appearance of an arterial pressure waveform corresponding to the QRS complex of the ECG (see weaning from device support). Remember that pulsatile LVADs are not synchronized with the ECG. Initiating TAH differs in that both ventricles will beat immediately, so one can rapidly wean off of bypass onto TAH support.
E. Initiation of support for RVAD
1. Initial considerations. Isolated RVAD support is less common than LVAD or BIVAD support, but when used, the essential considerations are similar to LVAD (air elimination, adequate RV decompression) in some ways, and different in others.
2. Pulmonary circulation. If the RVAD is a pulsatile pump and RV preload is adequate, the pump will function adequately even if the PVR is very high. Even if flow is normal, excessive PA pressures may lead to pulmonary edema, so monitoring and control of PA pressures may be helpful. If the RVAD is a continuous-flow pump, however, excessively high PVR may limit flow.
3. Pharmacologic support. In most instances, some inotropic support of the LV will be necessary.
VII. Postoperative management and complications
A. Right-sided circulatory failure. Right-sided circulatory management is the key to perioperative care for MCSD patients. This is less important for a patient on a TAH or a BIVAD. However, as isolated LVAD is the most common use of this therapy, attention to right heart management and PVR is critical. Strategies include pacing (chronotropy), inotropes, and pulmonary vasodilators to increase flow through the pulmonary vascular bed. For additional considerations, see LVAD initiation.
B. Hemorrhage. Postoperative hemorrhage is common in this patient population. Cardiogenic shock often leads to hepatic congestion and renal insufficiency. Both of these processes lead to imbalances in platelet function and the coagulation cascade. The addition of CPB and the consumptive coagulopathy that can be initiated can exacerbate postoperative bleeding. In addition, exposing the blood to the foreign surfaces of the extracorporeal circuit and of the conduit cannulae can also lead to a consumptive coagulopathy. It should not be a surprise that postoperative hemorrhage and re-exploration for bleeding is a common occurrence. Warming the patient and replenishing platelets and factors of the coagulation cascade is often best accomplished in the ICU with the chest closed. Re-exploration for bleeding/tamponade can then be performed when the patient is adequately resuscitated. Use of a thromboelastogram (TEG) to help target the deficiency in the clotting process is gaining popularity. Transfused platelets and red blood cells (packed cells or whole blood) in transplant candidates should ideally be leukoreduced, because exogenous leukocytes induce alloimmunization that can develop antibodies against future potential donated organs. High levels of these preformed antibodies reduce the pool of otherwise-suitable donor organs for these patients. Fresh frozen plasma and cryoprecipitate do not have high leukocyte content and do not need filtration.
9
C. Thromboembolism. Thromboembolism is associated with all current assist systems. The unique design characteristics of each system as well as the patient’s underlying pathology establish the overall risk. Anticoagulation with warfarin is required with all assist devices except the Heartmate IP/XVE, which appears to have the lowest overall thromboembolic rate [15,16].
D. Infection. Device-related infection is the most common cause of morbidity in the chronically supported patient. Driveline and device “pocket” infections occur in up to 40% of these patients. The vast majority of these infections may be managed with chronic antibiotic therapy until transplantation. Infection of the blood-contacting surfaces of the device (valves or diaphragm) necessitates device change.
E. Device malfunction. Catastrophic device malfunctions occur infrequently but can be life-threatening. These include mechanical device failure, device separation or fracture, graft or valve rupture, and console failure. Minor device malfunctions occur more frequently and are usually addressed at the bedside. These include driveline cover tears and controller malfunctions. All of these malfunctions are becoming increasingly rare as yearly device modifications and software upgrades are introduced.
VIII. Weaning from VAD support
All the support systems (except the IABP) function independent of the cardiac cycle, and the arterial waveforms will not correspond to the patient’s QRS complex. For patients in whom the heart recovers, the heart will be able to eject and the arterial waveform will begin to display pulses corresponding to the QRS complex. When the device flows are weaned downward and cardiac preload conditions move toward normal, these ejections will become more prominent. If the device can be weaned to 1 L/min flow and the patient can maintain adequate perfusion with reasonable inotropic support, explantation can be considered. This evaluation can be performed in the ICU. TEE can once again be very helpful in assessing cardiac function as the heart is loaded. Final weaning from the device should be accomplished only in the operating room, as CPB is usually needed for device explantation.
IX. Management of the VAD patient for noncardiac surgery
These patients can be very ill if surgery is contemplated early after MCSD implantation. However, patients will often recover physiologically over the ensuing weeks and months. Patients who are weeks to months on support may safely undergo noncardiac surgical procedures more routinely [17]. The optimal approach involves asking some basic questions:
10
A. What chamber(s) are being assisted (LVAD, RVAD, BIVAD, or TAH)?
B. What type of pump is being used? Is it a pulsatile pump (generally afterload insensitive) or a continuous-flow pump (afterload sensitive)?
C. Is technical support personnel available to help troubleshoot the pump? This is critical for transport as well as intraoperative management.
D. How does one determine flow through the pump? This can be quantitative or qualitative, depending upon the pump, but knowledge of pump flow clearly assists in determining systemic vascular resistance and PVR, which in turn guide anesthetic drug selection, pharmacologic support, and volume management.
E. Will TEE be helpful? If major volume shifts are anticipated, use of TEE can be very helpful in assessing preload, valvular function, and intracardiac shunts.
F. What is the patient’s clotting status? For most pumps, multidrug anticoagulation may be required, whereas the Heartmate XVE systems do not require any anticoagulation or antiplatelet agents. This has major implications for reversal of anticoagulation (if safely possible) and blood component use. Reversal of warfarin and conversion to intravenous heparin may be indicated. In general, be prepared for transfusion of RBCs and other components.
G. What is the patient’s intravenous access? The more and the larger the intravenous cannulae, the better. Central venous access is desirable in most situations.
H. What pharmacologic support is the patient receiving? This will vary from no support to multiple inotropes, antiarrhythmics, vasoconstrictors, and pulmonary vasodilators. If nitric oxide is in use, this will require planning for transport and operating room use.
I. Will electrocautery affect the assist device? For most devices, this will not be a problem, but excessive electrocautery can intermittently interfere with the function of some pumps. If electrocautery poses a problem, either short (1 s) bursts may suffice or the use of an ultrasonic scalpel can be considered.
J. If defibrillation or electrical cardioversion is needed, how will it be most safely applied? In some cases, the pump may need to be separated from the driveline and operated manually during cardioversion, though most will tolerate DC cardioversion.
X. Future considerations
A. Totally implantable LVAD. The totally implantable LVAD will need to employ a replenishable source of power with an internal battery reserve. A transcutaneous energy transfer system (TETS) system has been employed in the AbioCor as well as the discontinued LionHeart (Arrow International, Inc., Reading, PA, USA). This has the potential advantage of improved infection risk, as it obviates the percutaneous driveline. Many of the current systems could adopt this technology.
B. TAH. Use of a TAH is gaining traction in the field of MCSD. There has historically been a psychosocial reluctance to pursue this technology as well as a perceived lack of need for this type of MCSD. The highly publicized struggle of the first TAH implant patient, Dr Barney Clarke, accounts for some of the psychological impact, as does the thought of excising one’s heart (often perceived as one’s soul) to replace it with a manmade pump. In addition, many physicians believe that the use of BIVADs provides a good alternative to TAH. Nevertheless, the higher flow rates that can be achieved with a TAH offer an advantage over BIVADs in large patients or in those with multisystem organ dysfunction.
C. “Platform” therapy. Many patients with end-stage congestive heart failure who are chronically supported by VADs will demonstrate some level of functional myocardial recovery. On rare occasions, recovery may be robust enough for removal of the device [18–20]. This phenomenon is currently being studied, as it remains unclear in whom this phenomenon will occur. The use of long-term mechanical support as a therapy for de novo myocardial recovery is a very promising area of investigation. Therapies could be instituted to enhance myocardial recovery such as clenbuterol [21,22], cellular transplantation [23], myocardial exercise, or vascular regeneration.
XI. IABP circulatory assistance
A. Indications for placement. Thought by some to be reserved for placement after one or more failed attempts at separation from CPB, the number of IABPs placed in the cardiac catheterization laboratory approaches or exceeds the number placed intraoperatively. Interventional cardiologists often place IABPs when high-grade lesions of proximal coronary vessels supplying large regions of myocardium are diagnosed, or when myocardial ischemia persists or MI occurs after an intervention such as coronary stent placement. Retrospective outcome studies for preoperative versus intraoperative placement of IABP for coronary artery bypass graft (CABG) patients suggest that preoperative IABP placement improves outcome and shortens hospital stay, especially for patients with low ejection fractions or those undergoing urgent or emergent CABG [24]. Indications for intraoperative IABP placement vary widely among centers and even among or within surgical teams. LV failure despite moderate-to-high-dose inotropic support and/or evidence of ongoing regional myocardial ischemia that is not amenable to surgical revascularization constitute the primary intraoperative indications. Moderately severe LV failure despite reasonable inotropic support that is thought to derive from an injury that will resolve (or greatly improve) within 24 to 48 hrs (e.g., LV stunning) constitutes the most common intraoperative indication. The definitions of “LV failure,” “maximal inotropic support,” and “ongoing regional myocardial ischemia” may vary widely among surgeons and anesthesiologists. Independent predictors of death among patients with intraoperative IABP insertion include New York Heart Association Class III or IV symptom level, mitral valve replacement or repair, prolonged CPB, urgent or emergent operation, emergent reinstitution of CPB, preoperative renal dysfunction, diabetes mellitus, RV failure, complex ventricular ectopy, pacer dependence, and IABP placement via the ascending aorta [24]. IABP assistance can be used during high-risk off-pump CABG as well, where the support of coronary perfusion pressure may be especially helpful while the heart is placed in positions that compromise cardiac filling or emptying. Rarely, prophylactic IABP placement may be appropriate for high-risk urgent noncardiac surgery. As an example, suppose a patient who has unstable angina pectoris and compromised LV function requires an urgent radical nephrectomy for cancer.
11
B. Contraindications to placement
1. Aortic insufficiency. Use of the IABP is relatively contraindicated in patients with AI. As the IABP inflates in the descending aorta during diastole to promote retrograde flow into the ascending aorta, this potentially increases aortic valvular regurgitation, further distending the LV at the expense of coronary perfusion.
2. Sepsis. As with any prosthetic intravascular device, bacteremic infections are difficult to treat if the prosthetic surfaces become seeded with bacteria.
3. Severe vascular disease. Placement of an IABP may be technically difficult in patients with atherosclerosis or other vascular pathologies. Such patients are more prone to arterial thrombosis during use of an IABP. Patients with abdominal aortic aneurysms are at increased risk for aortic rupture, although balloons have been successfully passed and used in such patients. For patients with severe aortoiliac or femoral arterial disease, another option is to place the balloon directly into the descending thoracic aorta. Alternatively, placement may be performed through a small vascular graft sewn to the subclavian artery. These options require a subsequent trip to the operating room to remove the device when such support becomes unnecessary.
C. Functional design. The IABP consists of an inflatable balloon at the end of a catheter that is typically advanced into the descending thoracic aorta percutaneously from the groin (Fig. 22.4). The balloon inflates during diastole, displacing blood from the thoracic aorta and increasing aortic diastolic pressure. Balloon inflation improves coronary perfusion pressure, increasing coronary blood flow to both the LV and the RV. During early systole, rapid balloon deflation reduces LV afterload and wall tension. IABP can improve myocardial energy balance at most by 15%. The IABP drive console consists of a pressurized gas reservoir that is connected to the balloon supply line through an electronically controlled solenoid valve. The gas used to inflate the balloon is either CO2 or helium. The advantage of CO2 is its high blood solubility, which reduces the consequences of balloon rupture with potential gas embolization. The advantage of helium is its low density, which thereby decreases the Reynolds number and allows the same flow through a smaller driveline. A tube with a smaller diameter decreases the potential for injury to the artery.
Figure 22.4 Placement of the IABP in the aorta. The IABP is shown in the descending aorta, with the tip at the distal aortic arch. During systole the balloon is deflated to enhance ventricular ejection. During diastole the balloon inflates, forcing blood from the proximal aorta into the coronary and peripheral vessels.
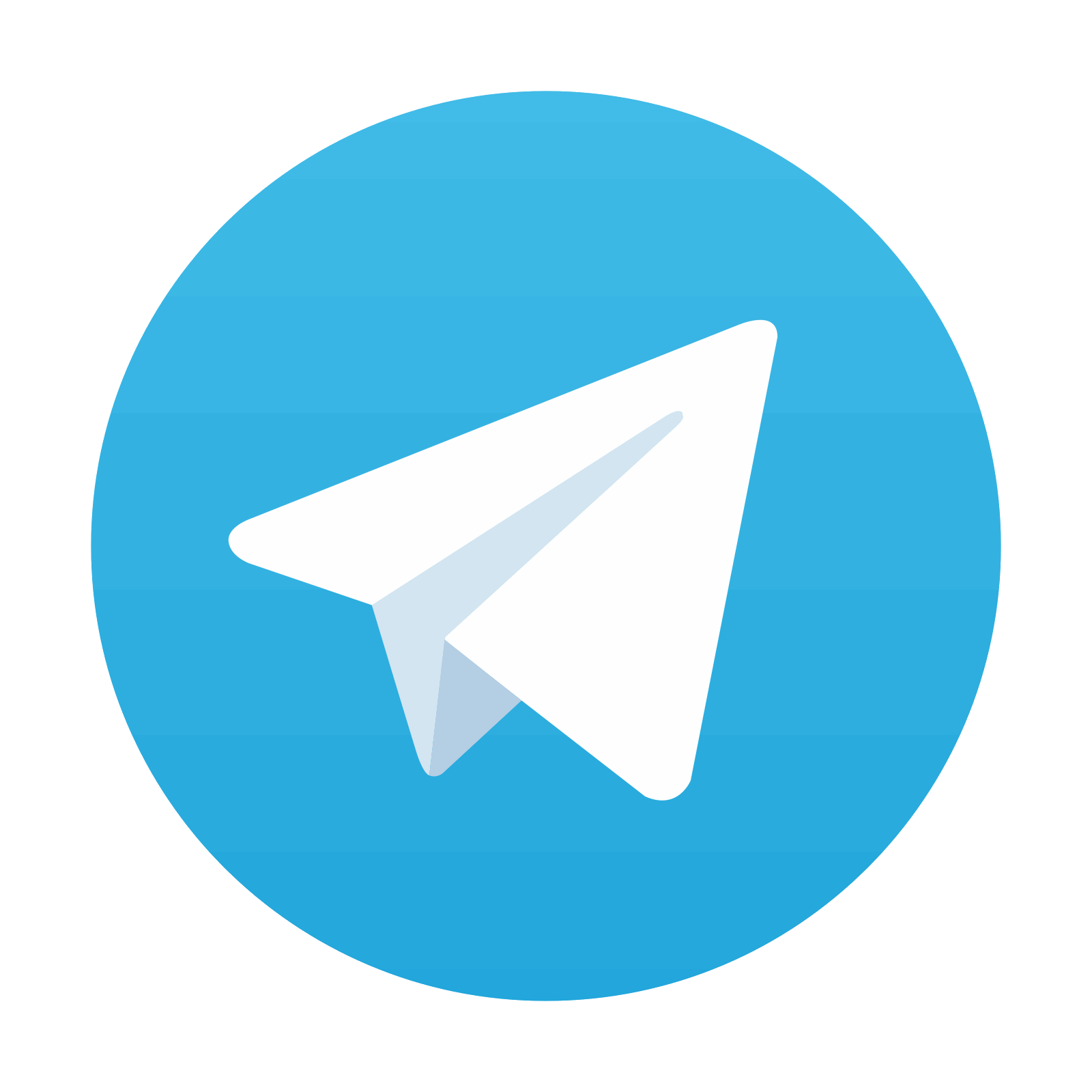
Stay updated, free articles. Join our Telegram channel

Full access? Get Clinical Tree
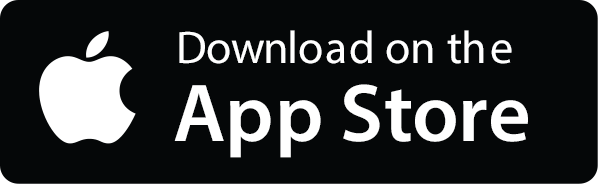
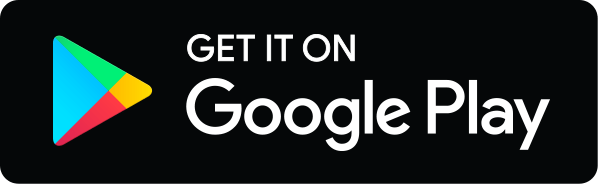