Chapter 77
Deep Venous Thrombosis and Pulmonary Embolism 
Deep venous thrombosis (DVT) and pulmonary embolism (PE) are different manifestations of the disease process known collectively as venous thromboembolism (VTE). The presentation of PE in particular can be notoriously variable and nonspecific, especially in critically ill patients in the intensive care unit (ICU) with preexisting reasons for cardiopulmonary compromise. These protean presentations may partly explain why PE remains one of the most frequently unsuspected autopsy findings in deceased ICU patients. This chapter describes the pathophysiology, diagnosis, prognosis, and therapy of VTE in critically ill patients, with extended consideration of the management of massive and submassive PE.
Pathophysiology
Venous thrombosis, whether in the lungs or the deep veins, begins as a microthrombus formation at a site of venous stasis or injury. Clot impedes blood flow, which promotes further vascular injury and extension of the thrombus. Upon propagating to a proximal vein, classically defined as at or above the popliteal vein, the clot may embolize to the pulmonary circulation. The presence of thrombus causes chiefly a mechanical obstruction to circulation, but it also triggers the release of vasoactive and, in the case of PE, bronchoreactive substances like serotonin that can exacerbate ventilation-perfusion () mismatch. Obstruction in the pulmonary circulation increases right ventricular (RV) afterload, raising RV wall tension and potentially leading to RV dilatation, dysfunction, and ischemia with diminished coronary perfusion. Concomitantly, the PE-induced
mismatch in the pulmonary circulation contributes to hypoxemia. This combination of increased RV oxygen consumption in the setting of reduced oxygen supply can lead to acute right heart failure, which is the likely cause for sudden death in patients with massive PE.
Acute changes in RV pressure and volume can also compromise the left heart. As the RV distends and stiffens, the interventricular septum can flatten or even bow into the chamber of the left ventricle (LV), altering the LV diastolic pressure–volume characteristics. This phenomenon can be detected echocardiographically, and it is often referred to as the “D sign” because the normally round parasternal short axis view of the LV takes on a “D” shape that results from the flattened interventricular septum. In patients with a patent foramen ovale (PFO), the acute rise in right atrial and ventricular pressures can result in shunting of blood across the atria from right to left. This situation may manifest as profound oxygen-refractory hypoxemia or as a paradoxical embolus from clot originating in the venous circulation traveling across the PFO to cause an arterial occlusion (such as an embolic stroke).
Although the most life-threatening complications of PE typically stem from cardiovascular compromise, PE also impairs gas exchange. This occurs chiefly through the creation of increased physiologic dead space in the unperfused or underperfused segments of the pulmonary circulation. Rising dead space typically manifests as an increase in minute ventilation and tachypnea, but it may also be recognized by a rise in the arterial partial pressure of carbon dioxide (Paco2) or a Paco2 that is abnormally “normal” despite increased minute ventilation. For example, an arterial blood gas (ABG) with a “normal” Paco2 = 38–40 mm Hg when a patient is breathing more than 30 times per minute should be recognized as abnormal and highly consistent with PE or another process increasing dead space. mismatch results in hypoxemia that tends to respond well to modest supplemental oxygen. Severe, oxygen-refractory hypoxemia is rare and suggests either significant circulatory compromise (shock) causing a low mixed venous oxygen saturation, right-to-left shunting across a PFO, or a superimposed pulmonary process such as chronic obstructive pulmonary disease (COPD) or pneumonia.
Risk Factors
Critical illness, with its resultant immobility and frequent vascular injury, is a significant risk factor for VTE and a recognized indication for prophylaxis, discussed at length in Chapter 12. In addition, well-defined acquired risk factors (Table 77.E1 ) include advanced age, malignancy, vascular injury resulting from surgery or trauma, spinal cord injury, pregnancy and the postpartum period, obesity, COPD, immobility, certain medications, indwelling vascular catheters, and prior VTE. Risk factors may also be heritable thrombophilias, such as genetic deficiencies in protein C or S, any of the antiphospholipid antibody syndromes, or the factor V Leiden mutation, which is the most common genetic risk factor. The presence of one or more risk factors is highly relevant in assessing patients with possible VTE and is included in diagnostic scoring systems.
Clinical Presentation
DVT symptoms are relatively straightforward. Pain or swelling in the calf or thigh are the hallmarks of lower extremity DVT. For DVT of the upper extremity, which is far less common in the overall population with VTE but constitutes a noteworthy proportion of DVT in critically ill patients, most cases (> 75%) are associated with indwelling catheters, cardiac devices (pacemakers/defibrillators), or cancer. Upper extremity swelling or erythema may be present, but occasionally upper extremity clots only come to attention by an inability to catheterize a central vein or by the failure of a central line to infuse or draw back. Although a smaller proportion of patients with acute upper extremity DVT, as compared to lower extremity DVT, present with symptomatic PE, the incidence of PE during the subsequent 3 months is similar regardless of DVT location, and thus the duration of anticoagulation treatment should be the same regardless of location.
The clinical presentation of PE is notoriously variable and nonspecific. Nonetheless, results from the Prospective Investigation of Pulmonary Embolism Diagnosis II (PIOPED II) trial, involving more than 800 subjects with suspected PE, point to dyspnea, pleurisy, and leg pain as the most common presenting symptoms. Most patients with PE present with dyspnea, frequently rapid in onset, while for almost 20% of patients, dyspnea is only present on exertion and for others it is most pronounced while lying supine (orthopnea). Pleuritic pain is frequent (40% of patients), but nonpleuritic chest pain may also occur. Wheezing or cough is present in almost a third of patients and is more common in patients with preexisting cardiopulmonary disease. Hemoptysis is uncommon, and when it occurs it tends to be scant; none of the almost 200 subjects with PE in the above-mentioned study had more than one teaspoon of blood. Calf or leg symptoms were present in ∼40% of subjects, similar to the first PIOPED study. Compared to those under 70 years of age, patients 70 years or older with PE are more likely to report pleuritic chest pain and leg symptoms. Notably, critically ill patients were excluded from the PIOPED II trial, and are more problematic to characterize with PE as they tend to have competing explanations for dyspnea, chest pain, or cardiopulmonary embarrassment.
The most common signs of PE include tachypnea ≥ 20 breaths per minute (∼half of patients), tachycardia ≥ 100 beats/minute (25%), and calf or leg findings (swelling, erythema, tenderness, or a palpable cord; 45%). More than one third of PE patients in the emergency room will have a normal Pao2 (≥ 80 mm Hg). Most patients demonstrate an increased alveolar-to-arterial (A-a) gradient when measured, but severely widened A-a gradient is rare. Respiratory alkalosis caused by hyperventilation is typical, although this finding is difficult to adapt to the critically ill population presenting with respiratory failure. Presentation with shock or circulatory collapse is infrequent, occurring in fewer than 10% of all PIOPED II subjects. Interestingly, presentation with shock was no less frequent in subjects without preexisting cardiopulmonary disease compared to those with such comorbidities. Diaphoresis, cyanosis, or fever ≥ 38.5° F are uncommon presentations for PE. In contrast, an abnormal cardiac examination (elevated jugular venous pulsation, increased pulmonic component to the second heart sound [P2], or an RV lift), though present in only one fifth of patients, helped to discriminate patients with PE from those without. Lung examination is abnormal in approximately a third of patients with PE, with crackles or decreased breath sounds being the most common findings and pleural rubs being distinctly uncommon. Lung exam did not help to distinguish those with PE from those without, unlike abnormal cardiac examination findings.
Because the symptoms and signs of PE remain nonspecific, the history and exam alone are rarely sufficient to adequately make or exclude the diagnosis, even when the patient is an excellent historian. By virtue of their critical illness, ICU patients are frequently unable to communicate their symptoms and have numerous potential explanations for observed tachypnea or tachycardia, making the diagnosis even more challenging. In addition, unstable patients are often poor candidates for immediate transport to a more definitive diagnostic procedure, and they may have organ dysfunction such as renal insufficiency that make such procedures problematic. The contributions of various tests in evaluating suspected PE are described in this chapter. Figure 77.1 presents an algorithm for approaching the diagnosis of VTE in critically ill patients.
Diagnosis
Clinical Prediction Rule: Wells Criteria
Failure to diagnose PE is a serious management error, as a portion of untreated patients may die. A clinical decision rule (Table 77.1) known as the Wells criteria helps to risk stratify patients with suspected PE to avoid unnecessary testing in low-risk patients. This rule has been extensively tested and validated in stable patients, both hospitalized and ambulatory. The Wells criteria assign points based on clinical signs and symptoms of DVT or PE, risk factors of the patient (prior VTE, malignancy, immobility), and the presence or absence of an alternative explanation for the patient’s clinical deterioration. When anticoagulation was withheld based on a low (< 2) Wells score and a negative D-dimer level, the incidence of VTE in the subsequent 3 to 6 months was < 1%. Although the Wells criteria have not been tested in critically ill populations, its central tenets—assessing the patient’s global risk for PE based on patient historical factors, clinical presentation, and differential diagnosis—remain a rational approach to initial risk stratification.
Plasma Biomarkers: D-dimer, Troponin, and Brain Natriuretic Peptide (BNP)
In low-risk patients with suspected PE, a normal D-dimer level identifies a subgroup in whom it appears safe to withhold anticoagulation. In 3000 noncritically ill subjects with suspected PE, withholding further testing for PE and anticoagulation for a Wells score < 2 and a normal D-dimer level led to a rate of VTE occurrence in the subsequent 3 months of only 0.5%. In subjects considered to be at moderate or high risk for PE, D-dimer testing has not proven helpful and thus is not recommended. In addition, just as D-dimer is frequently elevated by surgery, pregnancy, or a comorbid illness such as cancer, critical illness and activation of the coagulation system may elevate D-dimer in the absence of thromboembolic phenomena. Use of a D-dimer in evaluating an ICU patient’s risk for PE should thus be restricted to low-risk patients. Cardiac-specific biomarkers such as troponin or brain natriuretic peptide (BNP) are neither sensitive nor specific enough to be used to diagnose PE, but troponin in particular may be useful in risk stratifying a patient and deciding on the level of therapy, as discussed later.
Other Non-invasive Studies
In critically ill trauma patients, the combination of a 10% fall in pulse oximetry saturation and preserved static lung compliance proved to be both sensitive and specific in evaluating patients for PE. Electrocardiogram (ECG) is helpful in suggesting other potential etiologies for chest pain or dyspnea and may demonstrate signs of acute RV strain (incomplete or complete right bundle-branch block, T-wave inversions in the anterior precordium, or an S wave in lead I with a Q-wave and T-wave inversion in lead III [S1Q3T3 sign]), though these findings are not specific for PE. (For more information, go to www.expertconsult.com.)
Ultrasonography
Both ultrasonography and impedence plethysmography (IP) are highly sensitive and specific tests for symptomatic DVT. Ultrasonography has essentially supplanted IP, with widespread use and reported positive and negative predictive values approaching 100% in patients with symptomatic lower extremity DVT. In asymptomatic patients, including those with no leg complaints but presenting with PE, clots are more likely to be distal; serial ultrasound examinations (separated by ∼1 week) may document clot extension. The utility of ultrasound for upper extremity DVT seems to be equally good when clots are symptomatic; contrast venography is the diagnostic standard if ultrasound is inconclusive.
Echocardiography
Echocardiography is an attractive tool for the critically ill patient with suspected PE given its portability and non-invasiveness. Unfortunately, echocardiography is insensitive in the detection of PE and cannot be used as an individual diagnostic study. In prospective trials of unselected patients with suspected PE, the sensitivity of transthoracic echocardiogram (TTE) ranged from 29% to 52%. However, certain echocardiographic findings are specific for PE, the best of which is the McConnell sign, described as RV-free wall hypokinesis or akinesis coupled with normal or hyperkinetic RV apex performance. At least two studies have reported specificities ≥ 96% for this finding. However, this sign is uncommon and carries only 16% sensitivity, so failure to detect this finding does not exclude PE. Likewise, echocardiographic visualization of clot in the right atrium, RV, or pulmonary artery (via the transesophageal probe) clinches the diagnosis of PE, though this occurs infrequently. Other helpful criteria include elevations in the ratio of RV to LV end diastolic dimensions (≥ 0.7), the ratio of RV area: LV area ≥ 0.66, or the “D sign” or interventricular septal shift, with specificities in the 75% to 85% range. It is worth emphasizing that ventilated patients with severe hypoxemia and widespread hypoxic pulmonary vasoconstriction (e.g., those with the acute respiratory distress syndrome [ARDS]) also can demonstrate echocardiographic criteria of acute RV strain or failure, so that this finding is not specific. Because the chest radiograph for patients with PE is rarely associated with the dense airspace consolidation characteristic of hypoxemic respiratory failure, a good practice is to interpret right ventricular echocardiogram findings in the context of the chest radiograph. If the right heart strain is marked and the chest radiograph reveals dense consolidation and the patient is hypoxic, hypoxemic respiratory failure is the likely cause of the echocardiographic findings. In contrast, severe right heart strain in the absence of airspace filling on chest radiograph is highly suspicious for a PE.
As discussed in further detail later, the echocardiographic demonstration of RV dysfunction reliably predicts an increased risk of fatal PE and thus plays a role in risk stratification for the management of critically ill patients with PE. However, controversy remains as to whether the detection of RV dysfunction should change or escalate the management of PE. There is near consensus that the documentation of RV strain in the setting of PE should at a minimum prompt ICU management of the patient with close observation for possible deterioration.
Chest Radiographic Studies
Chest Radiograph and Chest Computed Tomography
While the chest radiograph is often abnormal in the presence of PE, there is no sensitive or specific finding that confirms or rules out PE. The three “classic” findings of Hampton’s hump (a peripheral wedge-shaped opacity resulting from infarction), Westermark’s sign of focal oligemia, or Palla’s sign of an enlarged right descending pulmonary artery are all notably uncommon. Platelike atelectasis, subtle areas of volume loss, or indistinct opacities are common, but the chest radiograph may also be completely normal.
Computed tomographic angiography (CTA) (i.e., CT angiography) has largely become the diagnostic standard for PE. Although not perfect, a CTA can be very helpful when used in the context of a patient’s pretest clinical probability for PE. As demonstrated by the PIOPED II study, when the clinical probability for PE is intermediate or high, a positive CTA has a very high positive predictive value of 92% to 96%. Similarly, a negative CTA has an excellent negative predictive value of 89% to 96% when the clinical probability is either low or intermediate.
However, the diagnostic accuracy falls when the finding of a CTA is discordant with the clinical probability. Among low probability patients, 42% of positive CTAs are actually “false positive” with respect to the reference standard. Consensus opinion is to treat such patients only if they have main or lobar pulmonary arterial involvement and to consider additional testing (repeat ultrasonography, ventilation-perfusion scan, or CT venography) for segmental or subsegmental defects. Among high-probability patients, 40% of negative CTA scans were “false negative” (i.e., positive by the reference standard). Thus, when clinical probability is high (Wells score > 6), a negative CTA essentially mandates further testing to exclude PE. In this instance, PIOPED II investigators recommend anticoagulation based on the high clinical probability while obtaining additional testing (extremity ultrasonography, magnetic resonance venography, potentially ventilation-perfusion scanning, or digital subtraction angiography).
Another potentially useful parameter is the alveolar dead space fraction (AVDSf), calculated by measuring the difference between arterial and end-tidal CO2: [AVDSf = (Paco2 – ETCO2)/Paco2]. As dead space rises, ETCO2 no longer approximates Paco2 because no gas exchange is occurring across the vascular beds distal to the embolus, and thus ETCO2 falls. Unless minute ventilation increases, Paco2 will rise progressively until a new steady state is reached (see Chapter 1). One study of hospitalized but noncritically ill patients reported that an AVDSf < 0.15 excluded PE with a sensitivity of > 97% and a negative predictive value of 98%. However, using AVDSf as a diagnostic test for PE is not recommended for ICU patients because they often have other reasons for increased alveolar dead space (see Appendix B for examples) and questions arise related to the accuracy and precision of measurements of ETCO2 as there often is no alveolar plateau in the expiratory capnographs. Nevertheless, an increased AVDSf lends support to a potential PE diagnosis, whereas a very low AVDSf argues against a large perfusion defect.
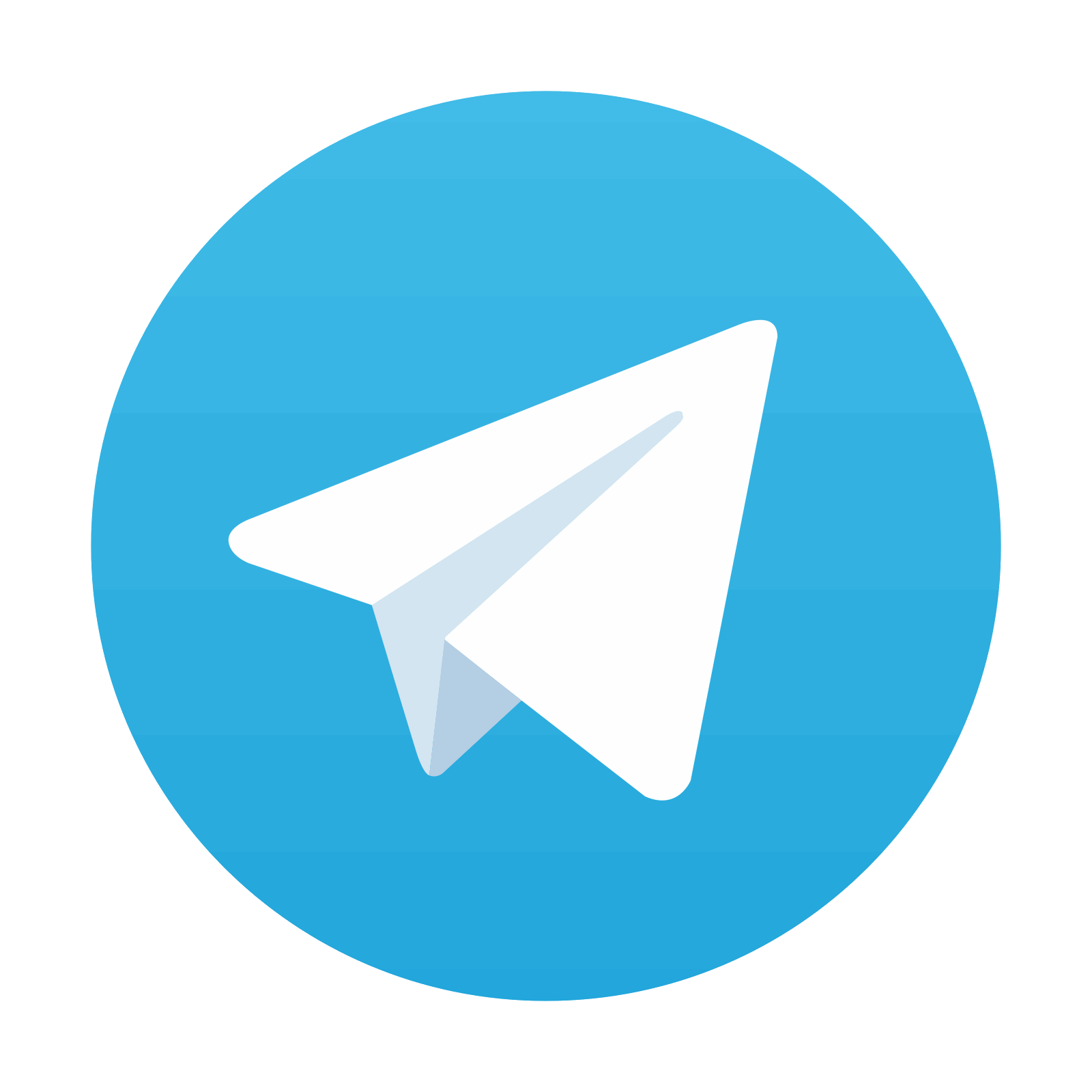
Stay updated, free articles. Join our Telegram channel

Full access? Get Clinical Tree
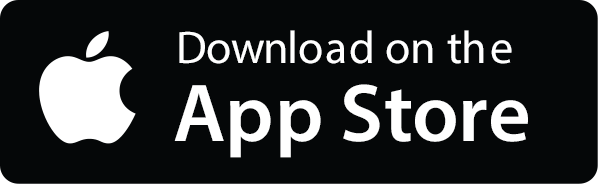
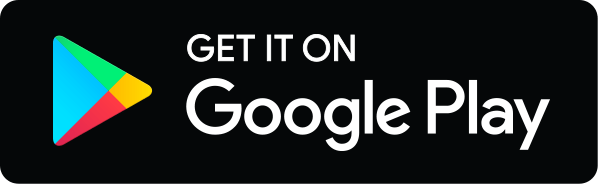