div class=”ChapterContextInformation”>
5. Remote Damage Control Resuscitation
Keywords
AustereEvacuationForwardFar-forwardHemostatic adjunctsOxygen debtPrehospitalRemoteRemote damage control resuscitation (RDCR)TourniquetWalking blood bank (WBB)Introduction
While the term remote damage control resuscitation (RDCR) may be relatively young, the principle is not [1]. For traumatically injured patients, providing basic care at the point of injury focused on controlling hemorrhage and transporting them to medical care as quickly as possible has been practiced in military conflicts for centuries. However, in order for the patient to receive advanced treatment, they must survive long enough to reach medical care. While many have recognized that limiting blood loss was key, the extent of what could be done in a prehospital setting remained very limited even in the twentieth and early twenty-first centuries. Interventions have typically centered around common sense; applying pressure, bandages, or basic first aid. In 1966, the National Academy of Sciences released “Accidental Death and Disability: The Neglected Disease of Modern Society” which focused significantly on the need to improve prehospital care and transport of injured patients in the United States [2]. In addition to recommendations concerning trauma system development, improving communications, and coordinating transportation programs to get patients to hospitals faster, it also highlighted the need for early bystanders to provide care to the injured patient. “Beyond the fifth grade of elementary school, every American citizen should be trained in basic first aid.” As the trauma care in hospitals evolved starting with damage control surgery (DCS) in the late 1980s and early 1990s, transforming into the principles now known as damage control resuscitation (DCR) , it was immediately recognized that these principles must also be implemented before patients reach the hospital to improve their morbidity and mortality [3–6]. This need for improved prehospital care has evolved to include programs such as the American College of Surgeons’ Advanced Trauma Life Support (ATLS) training, the National Association for Emergency Medical Technicians’ PreHospital Trauma Life Support (PHTLS) program, and the Department of Defense’s (DoD) Tactical Combat Casualty Care (TCCC). The new “Stop the Bleed” campaign sponsored by the American College of Surgeons goes one step further, targeting training to anyone, so that bystanders can apply basic principles of hemorrhage control before first responders reach the scene [7]. The modern basis of the bleeding control strategies taught in these programs, often without using the term, comes from RDCR and carries immense potential for impacting the lives of the traumatically injured in both the military and the civilian settings.
Sutton’s Law and Opportunities in RDCR
“Go where the money is,” the phrase known as Sutton’s law , has been used in medical teaching for decades to remind medical students and residents to focus on the area with the greatest likelihood to answer a question or make an impact. In trauma care, this is addressing hemorrhagic shock early and efficiently. Shock is the state when there is inadequate oxygen delivery to maintain aerobic metabolism in cells. This leads to the development of an oxygen deficit. Oxygen debt is a term that has been proposed to reflect the accumulation of an oxygen deficit over time as a way to quantify an individual’s degree of shock [8]. It has been known since the 1960s based on animal models that development of an oxygen debt following hemorrhage can lead to states where shock becomes irreversible and fatal [9]. Among survivors, the degree of debt and time to repayment correlate to morbidity including development of multiple system organ failure and coagulopathy [10, 11]. Therefore, prevention of building an oxygen debt, and repaying what has developed as early as possible, can improve patient outcomes [8, 12, 13]. The idea of reaching a state of irreversible shock following hemorrhage has been central to our understanding of when and why trauma patients die. When Dr. Trunkey first described the “trimodal” distribution of death following traumatic injury, targets for improvements in trauma care began to emerge [14]. Over 40% of trauma deaths occurred within the first hour, many before arriving to receive medical care , while the other “peaks” occurred at 1–4 hours and at >1 week due to late complications, primarily infection. In the time since, significant focus on the care we provide in the hospital has led to a change in this distribution. While the late peaks have flattened out or disappeared, early death remains responsible for the bulk of mortality [15, 16]. Data from the recent conflicts in Iraq and Afghanistan have shown the same in military systems. Nearly 90% of injury-related mortality occurs before casualties reach a medical treatment facility (MTF), with estimates that 25% of those injuries were potentially survivable as they are primarily due to hemorrhage [17]. As Sutton’s law suggests, the money is in intervening early, before the patient accrues an oxygen debt that cannot be repaid. This is where RDCR takes center stage.
The Distinct Field of RDCR
The Trauma Hemostasis and Oxygenation Research (THOR) Network is a multidisciplinary collaborative effort made up of clinicians, medics, educators, and researchers whose focus is to improve outcomes for the traumatically injured [18]. The group has taken a central role in not only defining RDCR but promoting the importance of research, education, and training on the topic. While some groups have advocated the inclusion of prehospital care as part of what is encompassed by standard DCR principles [19], the THOR Network has supported the distinction of RDCR from DCR for multiple reasons and has employed scientific principles to support its development [20]. DCR principles include rapid compressible hemorrhage control, rapid control of surgical bleeding, hypotensive resuscitation , balanced hemostatic resuscitation, avoidance of the overuse of crystalloid fluids, preventing and correcting hypoperfusion, acidosis, coagulopathy, hypothermia, and hypocalcemia. When DCR principles are applied in the prehospital setting, those providing the care and the resources available to them are different [21]. The practitioners are typically first responders and emergency medical personnel in civilian systems and combat medics, paramedics, hospital corpsmen, and even physicians in some military and civilian settings. Many of these providers can lack experience with patients in severe hemorrhagic shock. In the prehospital arena , there is also limited availability of many of the diagnostic (monitoring, laboratory) and therapeutic (blood component therapy, surgical capabilities) resources that are standard of care for hospital-based DCR. Airway management may also differ in the prehospital phase as the risks of intubation and positive pressure ventilation are magnified in the prehospital setting due to the inability to volume load and monitor for reduced preload and cardiac output in an intubated patient [22]. The threshold for intubation for RDCR is therefore different than when DCR is practiced in hospital. Management strategies may also differ in resource-rich areas with short evacuation times (e.g., urban cities) compared to austere environments with prolonged evacuations. Although the ultimate goal of reduced morbidity and mortality is the same in RDCR as in DCR, the short-term goal is to deliver a patient to a hospital, MTF, or forward surgical team with physiology that is salvageable and with the best chance of recovery. Another important reason to separately define DCR and RDCR is to appreciate the differences needed in future research efforts. We must study the application and outcomes of prehospital interventions with the different challenges and goals in mind. RDCR-specific data should ultimately be what drives, or tempers, widespread adoption of moving hospital therapies to prehospital settings or unique prehospital therapies themselves. Though the principles of DCR will be the framework for the remainder of this chapter, the goal is to highlight the unique considerations in RDCR.
Definitions
Remote damage control resuscitation terminology
Term | Definition |
---|---|
Remote or forward | The pre-hospital setting or phase of resuscitation |
Far-forward or austere | The environment where professional healthcare providers normally do not operate and basic equipment and capabilities necessary for resuscitation are often not available |
Delayed evacuation | >60 minutes from wounding until reaching a medical treatment facility that is capable of providing DCS and DCR |
Prolonged evacuation | >6 hours from wounding until reaching a medical treatment facility that is capable of providing DCS and DCR |
Acute coagulopathy of trauma | Endogenous coagulopathy that occurs as a result of severe traumatic injury |
Trauma-induced coagulopathy | Describe both endogenous and exogenous causes of coagulopathy following trauma |
Compressible Hemorrhage Control and Rapid Control of Surgical Bleeding
Hemostatic adjuncts in remote damage control resuscitation
Category | Adjunct | Mechanism of action |
---|---|---|
Mechanical | Gauze | External compression or wound packing directly to site of injury |
Topical hemostatic | External compression or wound packing directly to site of injury with gauze impregnated with substances with hemostatic properties (i.e., kaolin, chitosan) | |
Extremity tourniquet | Direct or proximal external compression on arterial supply to extremity | |
Junctional tourniquet | Proximal external compression to axilla or groin for injuries not amenable to extremity tourniquets | |
Abdominal aortic tourniquet | Proximal external compression to the abdominal aorta for pelvic or junctional injuries not amenable to extremity tourniquets | |
Pelvic binder | Prevention of mobility of pelvic fractures resulting in ongoing bleeding, decrease potential space for blood loss | |
Expandable hemostatic agents | Percutaneously injectable expandable substance for temporary control of non-compressible deep wound or abdominal hemorrhage | |
REBOA | Endovascular occlusion of aorta for non-compressible truncal or pelvic hemorrhage | |
Injectable | TXA | Antifibrinolytic agent which inhibits plasminogen |
PCC | Plasma-derived compound of coagulation factors II, IX, X, +/− VII | |
rFVIIa | Activated form of coagulant factor VII | |
Cryoprecipitate | Plasma preparation of coagulant factors, primarily fibrinogen | |
Fibrinogen concentrate | Standardized concentration of fibrinogen in lyophilized powder form |
Mechanical hemostatic adjuncts are really the first line of treatment available to control hemorrhage. They function by applying direct compression to, in, or proximal to bleeding injuries. Gauze, with or without impregnation of hemostatic substances, and extremity tourniquets are the most frequently utilized mechanical hemostatic adjuncts available. They are also among the oldest. Gauze with direct manual compression or wound packing can be one of the best and most efficient ways of controlling hemorrhage. While fairly straightforward to teach and perform, a potential downside is that it prevents the person applying pressure from continuing on with any other responsibilities, such as providing care to additional victims or to continue to fight/protect themselves during military conflicts or in civilian mass casualty scenarios. More recently, the addition of topical hemostatic agents such as kaolin (a white clay mineral) or chitosan (sugar molecule derived from shellfish exoskeleton) to gauze has been introduced. Data supports the hemostatic ability of these impregnated gauze products; however, most are single-arm studies without direct comparison to conventional gauze or among the various hemostatic compounds [27]. Injectable sponges for non-compressible junctional hemorrhage have also been adopted into TCCC training [28]. Tourniquet use dates back to the Roman era with more detailed descriptions in the 1700s [29]. While robust military data exists supporting the use of extremity tourniquets at the point of injury by nonmedical personnel, civilian adoption had been much slower even as recently as 5–10 years ago due to concern for the perceived risks of incorrect tourniquet placement and inappropriately selected patients. Fortunately, these concerns have been proven unfounded [30, 31]. Subsequently, tourniquet use has been adopted and incorporated in the civilian ATLS and PHTLS as well as “Stop the Bleed,” aimed at nonmedical personnel. This may finally meet the challenge from the National Academy of Sciences by increasing awareness and comfort of performing hemorrhage control by bystanders equivalent to performing cardiopulmonary resuscitation [32].
More advanced mechanical hemostatic adjuncts are also available. Junctional tourniquets are designed for injuries near to the axilla, shoulder, or groins that would otherwise not be controlled by a standard extremity tourniquet. They tend to be bulkier and are more difficult to apply, so specific training is needed. However, the addition of junctional tourniquets to the prehospital armamentarium theoretically expands the number of potentially controlled extremity hemorrhage by an additional nearly 20% [17]. Though limited data currently exists on their efficacy outside of cadaver and animal studies [33–35], several products are available and training is included in military programs including TCCC [36]. Abdominal aortic tourniquets apply external pneumatic compression to the abdominal aorta, targeting otherwise non-compressible pelvic or lower extremity junctional hemorrhage. These have yet to see widespread adoption as they require more training to place and provide limited additional hemorrhage control to the previously described tourniquets and have the potential to limit respiration due to abdominal compression. Further evidence on their utility is needed as swine model reports remain mixed and other data remains limited to case reports [33, 37–39]. Pelvic binders, however, have been widely used for decades and have been incorporated in ATLS and TCCC [40]. Unlike a tourniquet, the principle behind a pelvic binder is to limit and prevent additional motion that can cause ongoing bleeding and to decrease the potential space for blood loss, not to directly compress the arterial supply proximal to a site of active hemorrhage. While widely applied, the actual data supporting their use is also limited, and risk for skin necrosis with prolonged placement is real. As such, trauma organization guidelines do recommend their use but with caution [41]. Two additional emerging mechanical agents warrant mention. Retrograde endovascular balloon occlusion of the aorta (REBOA) has already generated significant interest and growing adoption in hospital settings for DCR [42]. Japan has implemented it in limited settings by Emergency Physicians, prior to the availability of surgical support, with success in a small series [43]. While still in its infancy in many ways, the fact that it is deployable percutaneously, with the primary technical skill being placement of a standard femoral arterial line, the idea that at some point in the future the technology could be taught to combat medics and prehospital providers for field deployment for select patients holds significant interest [44]. Another emerging option for non-compressible hemorrhage is in percutaneously injectable expandable hemostatic material. The primary goal would be to cause rapid hemostasis in otherwise non-compressible infra-diaphragmatic abdominal hemorrhage. Such an expandable foam-based product is in development with DoD support [45]. The goal of all these products is to provide temporary control to sites that are otherwise not accessible externally allowing transport to a site where surgical capabilities exist, or as an adjunct for surgical control of massive bleeding. While data is limited, it seems the potential of these types of products is promising.
Avoiding the Overuse of Crystalloids
Another central principle of DCR that has significant applicability in RDCR is the concept of hypotensive resuscitation. Other terms in the literature that may be encountered include permissive hypotension, balanced resuscitation, or delayed resuscitation. Some variation may exist when the terms are applied however, the overarching concept is the same. As discussed above, prevention of ongoing hemorrhage is critical to the traumatically injured patient. An injured victim’s initial response to bleeding is to form a platelet plug as part of primary hemostasis. As such, this initial clot that forms can be tenuous. Part of the original thought process behind hypotensive resuscitation was to avoid “popping” the clot that had formed by aggressively resuscitating a patient to a “normal” blood pressure with crystalloids [46]. Targeting a palpable radial pulse and appropriate mentation, typically equivalent to a systolic blood pressure of roughly 90 mmHg, has been the resuscitation strategy adopted by the US military [47]. Data reported by civilian trauma centers indicates hypotensive resuscitation decreases transfusion needs and improves mortality in penetrating torso trauma upon arrival to the hospital [48, 49]. The principle, however, has been found to have additional benefits, as targeting lower blood pressures also means less fluid being administered. In austere settings, this allows for better resource utilization, but beyond that in situations with limited access to blood or blood components, this also prevents overuse of crystalloid fluids, which has been shown to contribute to coagulopathy, abdominal compartment syndrome , acute respiratory distress syndrome, and increased mortality in trauma patients [50–53].
Despite significant support in the literature, there are several major limitations that must be further studied in relation to hypotensive resuscitation in RDCR. First is that most studies have been in the setting of relatively short evacuation times. Data to support its safety in prolonged or even delayed evacuations is lacking. Additionally, hypotensive resuscitation is contraindicated in patients with, or patients suspected to have, severe traumatic brain injury. Since hypotension and hypoxia are known to worsen outcomes following severe traumatic brain injury, incorporating hypotensive resuscitation in prehospital management of multisystem trauma patients requires thoughtful application and more research into patient selection, since some patients may have severe TBI without it being appreciated by prehospital providers. Additionally, as elderly patients become a larger proportion of the trauma patients seen at civilian trauma centers, appreciation of the changes in physiology due to comorbid conditions, medications, and aging require thought regarding the risk to benefit ratio for the application of hypotensive resuscitation in this cohort as decrease from the patient’s baseline systolic blood pressure of just 10% on arrival to a trauma center has been shown to be more predictive of mortality in elderly injured patients than traditional fixed criteria physiologic parameters in a retrospective cohort. This data is supportive of a report by Eastridge that indicated an admission systolic blood pressure of <110 mmHg was associated with increased mortality. These knowledge gaps leave us with many questions about the optimal prehospital resuscitation endpoints we currently use, including the safe target systolic blood pressure. As a result, a THOR position statement published in 2018 concluded that for patients without severe traumatic brain injury, the goal of resuscitation for patients at risk of traumatic hemorrhagic shock should be a SBP of 100 mm Hg, recognizing that a range of 90 mm Hg to 110 mm Hg may be more practical. The position paper also states that for patients with severe TBI the goal for resuscitation should be for a SBP >110 mmHg. Future investigation centering on the amount and types of fluid, correct endpoints of resuscitation, and long-term outcomes such as end organ failure and survival to hospital discharge with acceptable neurologic outcomes represent needed areas of RDCR research.
These questions of application also lead to interest in other means of measuring and monitoring the severity of injury and efficacy of resuscitation. Markers of oxygen debt may be more appropriate targets as endpoints of resuscitation during RDCR. Markers such as lactic acid and base deficit, both now available as point of care testing, may be worthwhile additions to the available tools for those providing in transit care [8, 12, 13]. While both are feasible, limited data exists in well-designed clinical studies as markers to guide resuscitation, rather than to predict outcomes [54]. Other endpoints of resuscitation under exploration that have been used in the hospital setting but may have application to RDCR, include point-of-care ultrasound which has multiple potential prehospital uses and non-invasive Tissue Saturation Oxygen Monitoring (StO2) [55, 56]. StO2 is a technology that utilizes a commercially available infrared spectrometry probe to measure real-time tissue perfusion. Current literature has shown tissue saturation levels <70% or over 90% have better correlation to multiple clinical outcomes in trauma patients than standard physiologic markers such as tachycardia, base deficit, and lactate, correlation to the need for blood product transfusion and mortality [57, 58]. Another emerging technology is Compensatory Reserve Index (CRI) which utilizes non-invasive photoplethysmography sensors to tracks analog arterial waveforms and has shown better correlation to early signs of blood loss than traditional physiologic parameters [59]. Research on the utility of these and other emerging technologies in RDCR, as well as their potential advantage to provide continuous real-time feedback for response to intervention, is currently ongoing and would greatly support expansion of their use.
Prevent and Correct Acidosis, Hypothermia, and Hypocalcemia
Directly addressing and preventing the development of the lethal triad of acidosis, hypothermia, and coagulopathy must be a central focus of RDCR. Hypothermia , while seemingly simple to address is critical and can be challenging in prolonged evacuations from austere environments where both time and the elements may be working against the patient and those providing care. Even in urban settings with short transport ties, preventing/treating hypothermia is important. A recent study published from Norway identified that >70% of trauma victims were hypothermic (defined as a body temperature of <36° C) on arrival of emergency medical service providers [60]. All attempts should be made to prevent hypothermia through removal of wet clothing, passive, and active rewarming efforts including utilizing heated blankets and administration of warm fluids or ideally blood products when needed. Commercially available hypothermia prevention kits have been utilized by the US DoD since 2004 and are considered a central part of prehospital care and the teaching of TCCC [61]. Acidosis is the result of hypoperfusion and shock due to generation of acidic metabolites, lactate, and other unmeasured anions, during anaerobic metabolism, with the degree of acidosis correlating with mortality and prognosis [62]. While adaptive to some degree as it aids in offloading of oxygen from hemoglobin at the cellular level, it also has multiple negative effects on the body including increasing minute ventilation as compensation, decreasing responsiveness to catecholamines , and worsening coagulopathy. Directly correcting acidosis with base compounds, most commonly sodium bicarbonate, while logical intuitively should be avoided unless extreme states of acidosis are present resulting in severe hemodynamic instability. An additional factor that can contribute to acidosis is aggressive crystalloid fluid resuscitation. Normal saline has a pH of 5.5 and due to its chloride content it can cause a hyperchloremic metabolic acidosis. In addition, no benefit has been found with the use of hypertonic fluid. It was originally thought to have enhanced intravascular volume expansion ability with decreased volume administered. Data on hypertonic saline for hemorrhagic shock has been mixed, although in general, there is no benefit with its use over traditional crystalloids in a prehospital setting [63]. Lastly, though hypocalcemia is not part of the lethal triad, it is worth discussing. Hypocalcemia in patients with traumatic hemorrhagic shock may occur due to consumption as a co-factor for hemostasis and also due to citrate within blood product storage solutions. A review of military patients from the conflicts in Iraq and Afghanistan revealed that 70% of combat casualties that received blood were noted to be hypocalcemic on arrival to the MTF [64]. Hypocalcemia can contribute to several physiologic abnormalities, including hypotension, and has been reported to occur after as little as 2 units of red blood cells (RBCs). Some studies have found association between severe hypocalcemia, <0.9 mmol/L, and increased mortality in trauma patients, though at present no studies have shown that correcting this improves outcomes [65]. However, many institutions and organizations recommend calcium replacement in the setting of massive hemorrhage [65, 66]. Hypocalcemia should be directly addressed via the intravenous administration of calcium chloride or calcium gluconate. When ionized calcium concentrations cannot be measured, it is reasonable to empirically administer calcium intravenously for every 2–4 units of RBCs transfused.
Hemostatic Resuscitation
The final principle of DCR applied to RDCR is hemostatic resuscitation , which is the use of whole blood or a “whole blood equivalent” with individual blood components in a 1:1:1 unit ratio. Inherent in the principle of hemostatic resuscitation is avoidance, or at the very least limitation, of crystalloids and colloids. Military data strongly supports the use of fresh whole blood (FWB) for resuscitation [67, 68]. Walking blood banks are well suited for austere environments as they eliminate the significant challenges of storage while providing the pinnacle of resuscitative fluids. Unfortunately, they also carry with them unique logistic challenges, issues with screening for infectious diseases, and maintaining adequate donor pools [69]. Prescreening programs and the expansion of low-titer O+ whole blood use, which has shown to be safe as a universal donor, improve some of these issues [70, 71]. However, transition of WBB programs to civilian settings has remain limited, with the Royal Caribbean Cruise Lines having perhaps the most successful large-scale implementation of a civilian walking blood bank program, with 37 transfusion cases reported [72]. Expansion of the use of low-titer cold-stored type O whole blood is starting to gain favor as the benefits of whole blood resuscitation continue to be reported [73–75]. For RDCR, especially in settings of delayed or prolonged evacuation whole blood may be preferred compared to the use of component therapy (RBC, plasma, platelet units) as it is logistically very challenging to appropriately store and transport all three blood components. Patients who require shorter evacuation times may be adequately temporized with just RBCs or even RBCs and plasma before hemostasis is achieved at an MTF, but for severely bleeding patients with longer transport times the most beneficial component may be platelets, and the only feasible way to resuscitate a trauma patient with all aspects of blood is to use whole blood. Whole blood for prehospital use has been shown to be feasible in well-developed trauma systems through coordinated efforts between trauma surgeons, blood banks, and emergency services [76–79]. The use of advanced technology such as drones will further enhance the availability of blood products in remote settings [80].
To supplement whole blood, or when whole blood is not available, use of freeze-dried plasma can be advantageous. Its use has been successfully implemented by the Germans, Norwegians, French, and Israelis [81–83]. The availability of plasma prehospital is also important because data indicates that its early use is associated with improved outcomes for patients with severe TBI [84]. A recent RCT published by Sperry et al. also indicates the use of plasma prehospital improved survival compared to the use of crystalloids [85]. Freeze-dried platelets are currently in development and have the potential to dramatically improve RDCR hemostatic resuscitation capabilities in austere environments [86, 87].
Lastly, injectable hemostatic adjuncts have potential for significant impact in the prehospital setting as either a complement to blood products or when blood products are not available. Tranexamic Acid (TXA) is an anti-fibrinolytic agent that inhibits plasminogen and plasmin, which are key factors for clot lysis. Both the MATTERs and CRASH-2 trials report decreased mortality when administered within the first 3 hours following trauma though potential for increased thrombotic complications exists [88, 89]. Prehospital TXA use in both military and civilian settings has shown the practice to be feasible with ongoing prospective randomized trials currently in progress [90–92]. Other potential injectables include Prothrombin Complex Concentrate (PCC) , a plasma-derived compound coagulation factors II, IX, X +/− VII, activated recombinant factor VII (rFVIIa) , fibrinogen concentrates, and cryoprecipitate. While each has gained interest in empiric use in trauma, little data supports their use. rFVIIa has significantly fallen out of favor despite some evidence that it may decrease the need for massive transfusion, when given early [93]. Similarly, the utility of empiric PCC seems limited to reversal of warfarin and some of the newer oral anticoagulant agents as an adjunct to FFP [94]. These agents potentially could play a larger role as direct factor replacements based on functional coagulation studies such as Thrombelastography (TEG) or Rotational Thromboelastometry (ROTEM) . The RETIC study attempted to analyze if ROTEM-directed coagulation factor concentrates affected outcomes compared to the use of plasma. Unfortunately, this trial was limited by differences in the time to treatment in both groups that could have been avoided with the use of thawed plasma [95]. Currently, there is no high-quality data to support the use of these hemostatic adjuncts based on TEG or ROTEM data.
Conclusion
“You may delay, but time will not.”
Benjamin Franklin
Poor Richard’s Almanack, 1758
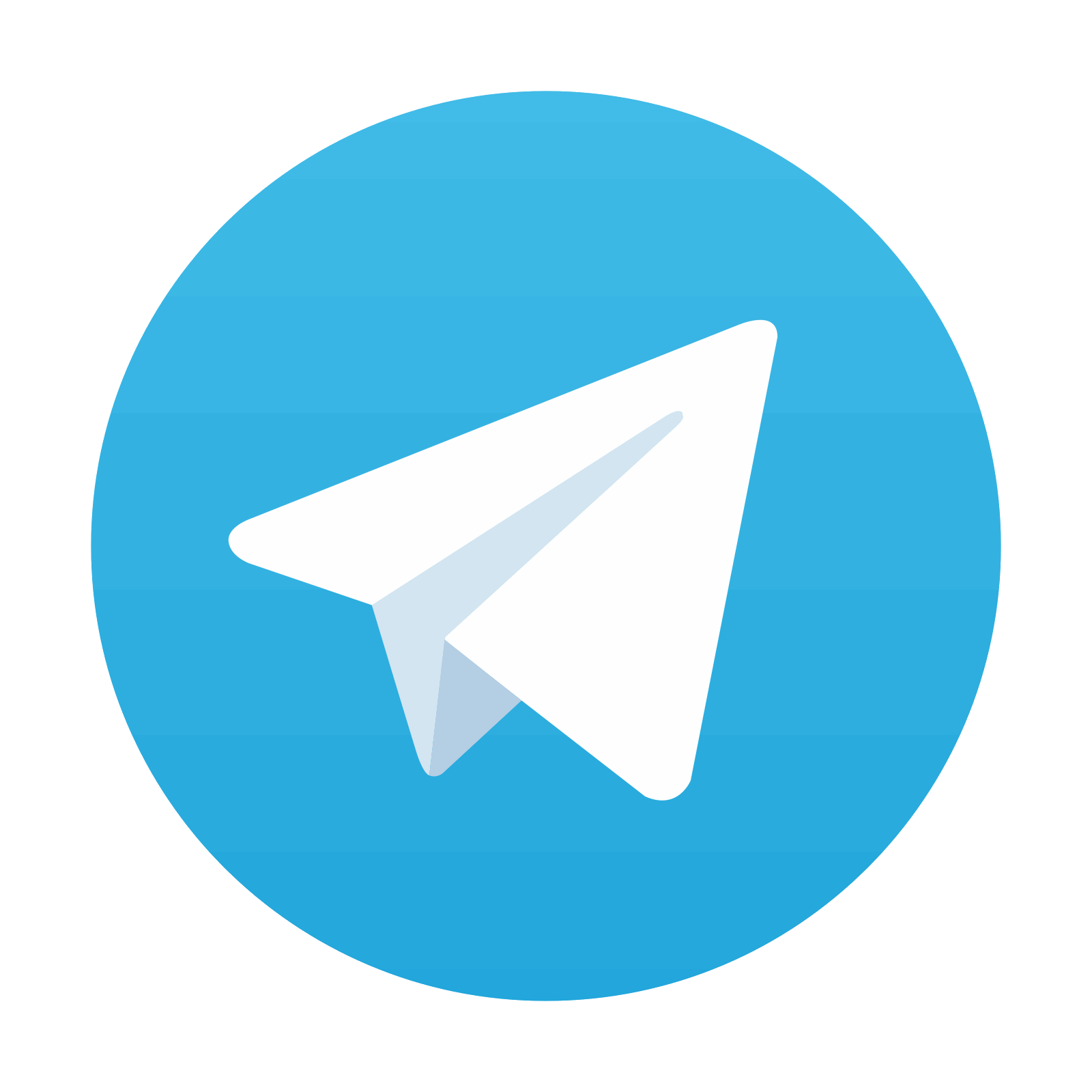
Stay updated, free articles. Join our Telegram channel

Full access? Get Clinical Tree
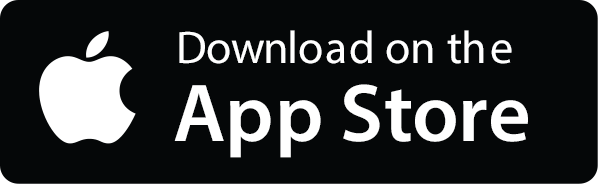
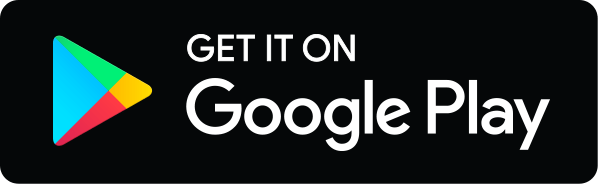