12 Critical Care of Acute Spinal Cord Injuries Melissa Y. Macias and Dennis J. Maiman In the United States, 160,000 new traumatic vertebral column injuries occur annually, 10 to 30% with concomitant spinal cord injury (SCI), yielding an average of 10,000 new cases of SCI per year.1 Currently, an estimated 225,000 to 288,000 individuals live with paralysis. In order of decreasing frequency, the most common level of neurologic injury is cervical (51.0%), thoracic (34.3%), and lumbosacral (10.7%). The most frequently injured regions are cervical C4-C6 (39.4%) and thoracolumbar T12-L1 (11.6%).1 The majority of spine and spinal cord traumas are caused by motor vehicle accidents (MVAs), followed by falls, acts of violence (most commonly gunshot wounds), and recreational sports activities (e.g., diving and contact sports). Interestingly, the proportion of sports-related and violence-related spinal cord injuries has decreased since 2000, whereas those due to falls have increased. The average age at injury is 37.6 years, an increase likely reflecting the rise in the median age of the general population since the mid-1970s.1 Accordingly, SCI in the elderly population (> 60 years of age) is most commonly caused by falls and has doubled since the 1980s. More common causes of SCI in younger populations remain MVAs, violence, and sports.1 Initial management of SCI is focused on basic emergency and trauma management protocols that institute immediate immobilization, stabilization, and safe transport to a high-level care facility. Improved medical management of SCI has facilitated a 60 to 90% reduction in the high mortality associated with SCI2 and remains crucial for the improvement in quality of life for SCI patients.2-4 Management of acute SCI patients requires knowledge of the postinjury pathophysiologic changes specific to this patient population and is optimized in an intensive care unit (ICU) setting, as both morbidity and mortality after acute SCI have been shown to be improved with continuous monitoring in the ICU.5 Though the evidence is at best anecdotal, some authors believe that timely surgical stabilization of an unstable spinal column injury with associated SCI appears clinically beneficial, facilitating more rapid mobilization and treatment of associated traumatic injuries, potentially reducing medical complications and reducing the duration of the stay in the ICU and the duration of the overall hospitalization.6-8 However, instability due to undiagnosed associated trauma is increased.9,10 This chapter identifies key medical considerations for management of acute SCI patients in the posttraumatic phase of care. The preeminent goals of ICU management are anticipation of acute complications and the prevention of intermediate and long-term complications of SCI. A systems approach is recommended, with specific attention to respiratory, cardiovascular, gastrointestinal, urologic, and integument systems with utilization of pharmacologic agents where appropriate. High-dose steroid infusion, primarily with methylprednisolone (MP), is the main pharmacologic treatment that is widely utilized following SCI, and the decision to initiate treatment is made during the initial resuscitation phase, but treatment remains rather controversial. When treatment is started within 8 hours of injury, beneficial effects at 6 weeks, 6 months, and 1 year were reported in the National Acute Spinal Cord Injury Study (NASCIS II) in 1990.11 The protocol included a bolus dose of 30 mg/kg intravenous (IV) over 15 minutes followed by 5.4 mg/kg/h IV for 23 hours if administered within 3 hours and for 47 hours if administered after 3 hours but before 8 hours of injury (Table 12.1). Though the mechanism of action remains incompletely understood, the beneficial effects are potentially mediated by their ability to reduce postinjury vasogenic edema and inflammation. Once considered the standard of care, more recent studies advocate not using methylprednisolone, primarily due to a critique of several shortcomings of the initial NASCIS II study, including lack of reproducibility.12–17 In other studies, including NASCIS III, high-dose MP failed to provide clinical benefit but rather demonstrated significant morbidity, which included prolonged ventilator dependency, hyperglycemia, pneumonia, gastrointestinal (GI) hemorrhage, acute corticosteroid myopathy, infection, and sepsis.12,18,19 The subsequent medical evidence fails to support high-dose MP treatment following SCI; however, the possibility of some clinical benefit following a catastrophic SCI injury has led to its continued use by some clinicians. Generally, treatment that commences during the resuscitation phase should be continued to completion in the ICU setting, with awareness and attention to its potential adverse systemic effects. Contraindications to high-dose MP usage include cauda equina syndrome, gunshot wounds to the spine, pregnancy, pediatric population (<13 years of age), prior use of maintenance steroids, and narcotics addiction.20 Table 12.1 Methylprednisolone Protocol
Pharmacologic Considerations
Methylprednisolone
Dosage | |
Bolus | 30 mg/kg over 15 minutes |
Maintenance | 5.4 mg/kg/h |
Duration of treatment 45 minutes after bolus | |
23 hours | Initiation <3 hours from injury |
47 hours | Initiation >3 but < 8 hours from injury |
Source: Bracken, et al. N Engl J Med 1990;322:1405–1411
Respiratory Considerations
Ventilation
Respiratory sufficiency and pulmonary function may be profoundly altered following neurologic dysfunction, especially following SCI at the cervical or thoracic levels.3,21 After ensuring airway protection during initial resuscitation following acute SCI, the adequacy of ventilation must be maintained. The key muscles of respiration are the intercostal, diaphragm, abdominal, and cervical accessory muscles.3,22,23 Intercostal muscles (T1-T12) stabilize the chest during expiration, and paralysis leads to a functional flail chest phenomenon that contributes to loss of adequate tidal volume (Vt). The diaphragm (C3-C5) generates approximately 50 to 60% of the inspiratory force of respiration or forced vital capacity (FVC). Diaphragmatic dysfunction may occur either by high cervical SCI or from fatigue following low cervical or high thoracic SCI where the diaphragm has assumed 100% of the workload of respiration. In either situation, lung volumes become diminished.3,23,24 As the primary muscles of expiration, loss of abdominal muscle function (T7-L1) may lead to increased end-tidal volumes and compromised lung capacity.3,25 Cervical accessory muscles, the scalene muscles (C3-C8), and the clavicular portion of the pectoralis major muscle (C5-C7) may be lost in patients with high quadriplegia. More often, however, these muscles augment respiratory mechanics following acute SCI.
Marked reductions in tidal volume, FVC, and expiratory volumes may lead to the development of hypercarbia, hypoxemia, atelectasis, and inability to mobilize secretions. Generally accepted parameters for positive pressure ventilation include hypoxemia (PaO2 <80 mm Hg), hypercarbia (PaCO2 >50 mm Hg), or elevated respiratory rate (>35 breaths per minute).3,22 In the awake, cooperative patient, ventilation-perfusion abnormalities may be assessed with serial measurements of FVC, which is the maximal volume that may be exhaled after maximal inhalation. FVC incorporates tidal volume and inspiratory and expiratory reserve volumes, and is normally 65 to 75 mL/kg of ideal body weight. A drop to less than 15 mL/kg is indicative of impending respiratory failure.26
Continuous pulse oximetry monitoring, supplemental oxygen, and frequent arterial blood gas measurements are the mainstays in respiratory management of the SCI patient. Caution must be used, however, when interpreting arterial blood gas values, as they may be poor indicators of adequate ventilation, changing only after pulmonary and cardiovascular reserves are depleted.3 Early hypocap-nia may reflect activation of primary pulmonary receptors innervated by vagal afferents producing dyspnea and an increased ventilatory drive rather than a carotid chemoreceptor response to hypoxemia.27 Subsequently, normal to low PaCO2 may be misinterpreted to reflect adequate respiratory reserve in the face of respiratory distress. Treatment of supplemental oxygen may mask ensuing hypoxemia, further exacerbating altered pulmonary pathophysiology. Thus, as fatigue inevitably ensues with progressive tachypnea, exacerbation of profound ventilation-perfusion abnormalities may lead to acute respiratory arrest. Consequently, close attention to the respiratory rate is essential in the SCI patient as it may reflect impending respiratory failure.3,28
High cervical cord injuries often result in respiratory mechanical insufficiency requiring intubation. Even so, lower cervical, thoracic, or lumbar cord injured patients may require intubation in the setting of trauma to the chest or respiratory complications secondary to impaired cough or mobilization of respiratory secretions. The form of mechanical ventilation varies according to the level of alertness and respiratory effort. Altered pulmonary physiology in the SCI patient results in inadequate lung expansion. Therefore, positive pressure ventilation is preferred and can be provided with volume-cycled mechanical ventilation using either synchronized intermittent mandatory ventilation (SIMV) or assist control ventilation (ACV).28 The former allows spontaneous and unassisted breathing between mechanical breaths. With SIMV, pressure support is advised to minimize fatigue that may result from an increased work of breathing through the high resistance of the ventilatory circuit. Extrinsic positive end-expiratory pressure (PEEP), which ceases expiratory flow at a preselected pressure, may be added to IMV settings. By preventing alveolar collapse, PEEP improves lung compliance and overall gas exchange, allowing inspired oxygen (FIO2) to be reduced to less toxic levels (<60%). The latter, ACV, fully assists all spontaneous breaths through a full cycle and provides breaths at a pre-selected rate and by the ventilator. Hyperinflation is an undesirable feature of ACV, resulting from a decreased time for exhalation, and is accompanied by intrinsic or auto-PEEP, leading potentially to hypercarbia, alkalosis, and tachypnea. Patients with sufficient respiratory effort but at risk of fatigue benefit most from SIMV, whereas ACV is appropriate in those with minimal respiratory effort.3,28
No single protocol for weaning a SCI patient off ventilator support appears to have a greater advantage over another. General criteria indicative that a patient may be successfully weaned include a vital capacity >10 mL/kg, tidal volume >5 mL/kg, peak inspiratory pressure >20 cm H2O, and a respiratory rate <30 breaths per minute.22,26 A gradual wean may be accomplished by slowly decreasing the rate of intermittent mandatory ventilation, continuous positive airway pressure support, or extending periods off ventilator support by using a T-piece trial for increasing periods of time. The latter is commonly used in our institution; there is clinical evidence that the diaphragm can be “trained.”3,21 Quadriplegic patients from high cervical cord injury are least likely to be weaned off ventilator support and may require a tracheostomy,29 the timing of which should be coordinated with the possible necessity of surgical stabilization. Yet any patient requiring prolonged mechanical ventilation, usually more than 14 days, with inability to be successfully weaned off the ventilator should be considered for tracheostomy.
Pneumonia
Although postural changes, sterile nasotracheal suctioning, and aggressive chest physiotherapy remain important in the armamentarium for minimizing atelectasis and bronchial secretions, pulmonary complications are the most common cause of morbidity and mortality following SCI, with the majority of them due to pneumonia.2,26,30 Pneumonia occurs in 5 to 20% of cervical-level SCI patients.2,30,31 Even so, the use of prophylactic antibiotics is not recommended owing to the increased risk of antibiotic-resistant infection.25,30 Early placement of a nasogastric tube for removal of stomach contents is indicated to minimize the risk of aspiration pneumonia in the right lower lobe resulting from gastric atony with SCI.32 Nonetheless, the vicious cycle of poor secretion clearance, progressive bronchiole obstruction, ventilation-perfusion abnormalities, and atelectasis is the more common cause of pneumonia in SCI patients.3,22,31 Combined with these factors and the acute angle of the left bronchus compared with the right bronchus, pneumonia is more frequently observed in the left lower lobe following SCI.29 The microorganisms involved are likely similar to those found in most nosocomial pneumonias, which are polymicrobial and derived from the flora of the oropharynx.28,31 The most common isolates are gram-negative aerobic pathogens.28 In mechanically ventilated patients, development of ventilator-associated pneumonia (VAP) is usually caused by Streptococcus pneumoniae or Haemophilus influenzae if within the first 4 days of ventilation or Pseudomonas aeruginosa or Staphylococcus aureus after 4 days.28
The clinical signs of pneumonia in SCI patients include fever, leukocytosis, hypoxia, and purulent sputum associated with infiltrates evident on chest radiographs.31 Chest radiographic diagnosis remains challenging owing to the likely presence of atelectasis that predisposes patients to pneumonia.3,31 Further caution is noted in interpretation of these clinical signs as fever and leukocytosis may accompany atelectasis alone. Tracheal suctioning or bronchoscopy may be used to obtain a sampling of tracheal secretions for Gram stain and culture analysis. Antibiotic therapy should be directed at the most likely organisms until final culture results are available, but it should not be withheld during progressive infection while awaiting culture results. Alternatively, antibiotic treatment should not be changed if the patient shows improvement concomitant with radiographic evidence of clearance based solely on a culture report suggesting different or additional organisms.3
Thromboembolic Disease
Following SCI, venous stasis secondary to decreased vascular resistance and the loss of muscle contraction potentiates thromboembolic disease. The incidence of thromboembolic complications in the acutely SCI patient population may be as high as 100% based on radiolabeled fibrinogen scanning.3,20,33,34 The risk for deep vein thrombosis (DVT) may be nearly ninefold greater in SCI patients compared with the generalized trauma population.34,35 Likewise, the risk for catastrophic pulmonary embolism (PE) following DVT is elevated in the SCI population, 2 to 10%, compared with the general surgical population, 0.1% to 0.8%.36 Yet the incidence of clinically recognizable DVT in the SCI patient has been reported at 15% and for PE only 5%.37 The risk of either DVT or PE appears greatest in the acute phase of injury and up to at least 3 months following injury.22,33,38 Not surprisingly, substantial morbidity and mortality is associated with the development of thromboembolism.2,33,35 Consequently, a high index of clinical suspicion and aggressive prophylactic measures are recommended for 3 months’ duration.
Choices for prophylactic treatment in patients with SCI and severe motor deficits include mechanical devices, such as external pneumatic compression devices, compression stockings, rotating beds or electrical stimulation, and anticoagulants, such as low-dose heparin, low molecular weight heparin (LMWH), and Coumadin.3,20,33,39 Clearly, mechanical devices carry little if any risk; however, they do not appear to provide sufficient prophylaxis in isolation. Similarly, anticoagulation modalities, while potentially increasing the risk of hemorrhage within the injured spinal cord, particularly within the first 72 hours of injury, do not provide optimal thromboembolic prophylaxis when used alone.3,20,33 Instead, combined therapy appears to provide the greatest protection against thromboembolism employing the use of LMWH or adjusted-dose or low-dose heparin in conjunction with pneumatic compression devices, electrical stimulation, or rotational beds.3,20,33,40,41 Neither low-dose heparin nor oral anticoagulation alone is recommended for prophylactic treatment of thromboembolic disease.20,33 Thus, a reasonable ICU management strategy would be to employ mechanical devices in the acute phase followed by the addition of low-dose heparin (5000 units subcutaneously every 8 hours), adjusted-dose heparin (titration of subcutaneous heparin every 12 hours to a partial thromboplastin time [PTT] of 1.5 times control), or LMWH once primary hemostasis is evident and the risk of hemorrhage is significantly reduced, approximately 72 hours after SCI.3,33,35,40 At our institution, we routinely employ Lovenox (LMWH) as our anticoagulation agent of choice, concomitant with pneumatic compression devices.
In the SCI patient, the diagnosis of DVT should be suspected with the development of an unexplained fever, leg edema, or redness, or an increase in leg circumference or skin temperature.22,39 Mortality from DVT in SCI patients is 9%.20 Diagnostic tests for DVT include duplex Doppler ultrasound, venous occlusion plethysmography (VOP), fibrinogen scanning, D-dimer analysis, and venography.20,33 Once the “gold standard,” venography is invasive and costly; therefore, it is reserved in the setting of high clinical suspicion with a negative ultrasound study.33 Duplex Doppler ultrasonography is the mainstay test because of its availability, low cost, and accuracy in detecting DVT with a sensitivity of approximately 90%.3,33 D-dimer measurement is highly sensitive but not specific,42 and fibrinogen scanning is not widely employed.3,20,21,33
Upon confirmatory diagnosis of DVT, full anticoagulation with heparin followed by oral warfarin for 3 to 6 months with international normalized ratio (INR) levels of 1.5 to 2.5 is recommended.40,43 In patients with confirmed DVT but in whom anticoagulation therapy is contraindicated or ineffective, a vena cava filter should be placed.3,40,43–45 Subsequently, the PE must be attentively monitored, as the typical symptoms of tachypnea, tachycardia, or pleuritic pain may be obfuscated from the underlying respiratory pathophysiology observed following SCI. Although pulmonary angiography remains the gold standard in diagnosis of PE, more often chest radiography, ventilation-perfusion scans, or, more commonly, spiral computed tomography46 with acute changes in arterial blood gases and electrocardiogram may be useful in establishing the presence of PE.3,21,22,40 The arterial blood gas changes may be difficult to interpret with the pretext of underlying pulmonary compromise, yet decreases in oxygen saturation or arterial oxygen with a concomitant increase in arterial carbon dioxide should raise the suspicion of pulmonary emboli. The electrocardiogram may demonstrate signs of acute cor pulmonale, especially with a large PE. The classic triad on the electrocardiogram, though seldom observed, includes a large S wave in lead I, a large Q wave in lead III, and an inverted T wave in lead III (“S1Q3T3”). More commonly observed is sinus tachycardia. In addition to full anticoagulation as with DVT treatment described above, the placement of a vena cava filter is recommended to prevent further emboli44 (Table 12.2).
Table 12.2 Thromboembolic Disease
Prophylaxis | |
Mechanical devices* | |
External pneumatic compression devices | |
Compression stockings | |
Rotating beds | |
Electrical stimulation | |
Anticoagulation* | |
Low molecular weight heparin | 1 mg/kg SC q12h |
Low-dose heparin | 5000 units SQ q8h |
Adjusted-dose heparin | Titration of SQ heparin to partial thromboplastin time (PTT) 1.5 X normal |
Deep vein thrombosis (DVT) diagnosis | |
Clinical | |
Unexplained fever | |
Leg swelling or redness | |
Diagnostic | |
Duplex Doppler ultrasound (most common) | |
Venous occlusion plethysmography | |
Fibrinogen scanning | |
D-dimer analysis | |
Venography (gold standard but rarely used) | |
Treatment of DVT | |
Anticoagulation | |
First phase: | 24 to 48 hours with full anticoagulation with heparin |
Second phase: | >48 hours, warfarin for 3 to 6 months with goal international normalized ratio (INR) of 1.5 to 2.5 |
Pulmonary emboli diagnosis: | |
Clinical findings | |
Tachypnea | |
Tachycardia | |
Pleuritic pain | |
Diaphoresis/anxiety | |
Diagnostic findings | |
Acute changes in arterial blood gases and ECG with confirmatory study: | |
Spiral computed tomography (most common) | |
Ventilation-perfusion scan | |
Pulmonary angiography (gold standard) | |
Treatment | |
Full anticoagulation usually for 3 to 6 months (as above for DVT but INR goal of 2-3) | |
Anticoagulation with vena cava filter |
* Combination therapy recommended, neither proven effective in isolation; initiate anticoagulation once risk of hemorrhage is decreased, approximately 72 hours postinjury.6,16,22,27
Some centers advocate placement of vena cava filters in all SCI patients as a principal prophylactic measure.2,47 Others, however, have demonstrated significant complications particular to SCI patients with compromised abdominal muscle tone that include distal migration, intraperitoneal erosion, and symptomatic IVC occlusion.48–50 Therefore, current recommendations for caval filters are for patients with thromboembolic events despite anticoagulation or for those in whom anticoagulation or pneumatic compression devices are contraindicated.2
Cardiac/Hemodynamic Considerations
Hemodynamic complications frequently accompany acute SCI and may include hypotension, dysrhythmias, reduced peripheral vascular resistance, and reduced cardiac output.51–53 This compilation of symptoms manifests as neurogenic shock and are principally the result of interrupted sympathetic pathways with unopposed vagal activity.3,53 Loss of sympathetic outflow causes vasodilation (leading to the pooling of blood in the periphery and third spacing of fluids) and the inability to generate tachycardia to increase cardiac output, subsequently relying mostly on increased stroke volume, which may or may not be obtained. The inevitable outcome is relative hypovolemia and hypotension.3,45,52 Hypotension with systolic blood pressure <90 mm Hg and a decreased heart rate of <90 beats per minute are observed in approximately 25% of patients following acute SCI,52 and may persist even after 4 to 6 L of fluid resuscitation.3 Clearly, volume depletion is not the cause of hypotension. Thus, in the absence of hemorrhagic hypovolemia from associated injuries, judicious administration of fluids is advised secondary to the risk of iatrogenic congestive heart failure, pulmonary edema, and hyponatremia.3,21,45,51
Table 12.3 Vasopressors in Spinal Cord Injury
Drug | Dose | Effects |
---|---|---|
Epinephrine | 1-8 μg/min | α- and β-agonist: prominent vasoconstriction but also activates B2-receptors causing vasodilatation and ↓ diastolic blood pressure; + chronotropic and inotropic cardiac effects |
Norepinephrine | 1-20 μg/min | α- and β2-agonist minimal effect on B2-receptors; prominent increase in peripheral resistance with increases in systolic and diastolic blood pressure but may get reflex bradycardia; + chronotropic and inotropic effects |
Phenylephrine | 40-100 μg/min | Purely α-sympathomimetic; noninotropic; use in SCI is highly cautioned due to reflex bradycardia |
Dopamine | 0.5-2.0 μg/kg/min | Dopaminergic with renal, mesenteric, coronary, and cerebral vasodilatation; + inotropic cardiac effect |
2-10μg/kg/min | α- and β1-agonist; vasoconstriction with ↑ systolic and diastolic blood pressure; + inotropic cardiac effect | |
>10μg/kg/min | α-, β-agonist and dopaminergic effects | |
Dobutamine | 2.5-10.0 μg/kg/min | Primarily β1– selective increasing cardiac output; + inotropic cardiac effect |
Continuous electrocardiographic monitoring of hemodynamic parameters is essential in the ICU setting. Once the diagnosis of hemorrhagic hypovolemia is excluded or adequately addressed, the placement of a pulmonary artery catheter is useful to monitor peripheral resistance, which may be <50% normal, and cardiac output.3,21,52 Treatment with vasopressors is often required. Optimally, a vasopressor with both α- and β-adrenergic components is used because of the desired vasoconstrictive effect to the peripheral vasculature and chronotropic/inotropic effects to the heart (Table 12.3). Either norepinephrine (0.05 to 0.20 μg/kg/min) or dopamine (1 to 10 μg/kg/min) is commonly used as a single agent. The former has prominent α1-/β1– with mild β2-adrenergic effects, resulting in prominent increases in peripheral resistance and both chronotropic and inotropic support to the heart, but may also be associated with undesired reflex bradycardia. The latter, on the other hand, is the pressor of choice. Dopamine, in a dose-dependent manner, primarily activates α1– and β-adrenergic receptors, resulting in optimal chronotropic cardiac and peripheral vascular improvement. Dobutamine (5–15 μg/ kg/min), selective for β-receptors, augments cardiac function but may reduce systemic blood pressure and is not widely used for patients with SCI. Although peripheral vascular resistance increases effectively with pure α-adrenergic agonists, such as phenylephrine, they are seldom used independently secondary to ensuing reflex bradycardia that is detrimental to cardiac function.3,21
The preeminent goal of therapy is the maintenance of adequate organ perfusion, which may be demonstrated by urine output of ≥ 0.5 ml/kg/h, prevention of acid/base abnormalities, and appropriate mentation.3,21,52 The optimal mean arterial pressure (MAP) to achieve this goal is unknown and may vary among patients. In the acute phase of injury, disrupted perfusion to the injured spinal cord may be further compromised by systemic hypotension, thereby potentially exacerbating secondary ischemic cord injury. Maintenance of a MAP at high to normal levels, >85 to 90 mm Hg for a minimum of 7 days, has been suggested to enhance neurologic outcome and reduce morbidity and mortality following acute SCI.51,52,54
Gastrointestinal Considerations
The spectrum of GI dysfunction following disruption of sympathetic innervation following SCI includes gastric atony, stress ulcerations, bowel obstruction, constipation, abdominal bloating, and fecal incontinence. Nutritional requirements and timing of enteral feeding are additional management considerations in the SCI patient population.3,22,55 Early placement of a nasogastric tube for decompression of stomach contents and secretions reduces the risk of vomiting and acute gastric dilatation, either of which may compromise pulmonary function through aspiration or decreased lung capacity, respectively.3 A decrease in nasogastric output typically occurs 2 to 3 days after admission and usually indicates a return of gastric motility.22,56 Additionally, the return of flatus and bowel movements provides more direct evidence of regained GI motility. Once gastric motility has returned, enteral nutrition should be slowly introduced and may play a key role in the prevention of stress ulceration.41 The key complication of early enteral feedings is high gastric residuals with an associated risk of aspiration.56 Additional concerns with premature feeding include exacerbation or recurrence of GI ileus, especially when vomiting is observed after prior tolerance of enteral feeding.3
Nutritional Support
Nutritional support is a key consideration for the hyperme-tabolic and hypercatabolic state of SCI patients. The resting energy expenditure calculated by the Harris-Benedict equation overestimates the caloric needs of patients with isolated SCI, likely attributed to the flaccidity of denervated musculature.57,58 The loss of muscle tone and activity also contributes significant nitrogen losses, resulting in prolonged overall negative nitrogen balance. Nutritional goals are to meet the caloric and nitrogen needs of the patient but not to restore nitrogen balance. Consequently, indirect calorimetry is the energy expenditure assessment method of choice, especially in the early postinjury period.55,57,58 Providing nutritional support in this early period of injury, within 7 days, is essential to minimize hormonal alterations manifest as increased plasma corti-sol and adrenocorticotropic levels, nutritional deficiencies, and immune dysfunction, all of which may lead to increased susceptibility to infection and delayed wound healing.3,55,59 Enteral feedings are the preferred route, providing maintenance of GI integrity and function. Parenteral nutrition should be reserved for patients with GI injury, prolonged ileus, or mechanical obstruction.3,55
Many commercial solutions are available for enteral feedings. Initially, continual rather than bolus feeding is better tolerated in the critically ill patient, with starting rates of 20 to 30 mL/h, incrementally increased to deliver the final goal amount as tolerated by the patient, approximately 30 to 40 kcal/kg/d in the acute postinjury setting.45,60,61 Enteral nutrition may be provided by nasogastric, orogastric, or, in the presence of high gastric retention volumes, small-bore nasoduodenal or nasojejunal tubes.28,31,60
Stress Ulceration/Gastrointestinal Hemorrhage
Stress ulcerations may occur in the GI tract due to an unopposed, persistent vagal tone accompanying the sympathectomy effect of SCI; they peak at 4 to 10 days postinjury.3,22,62 Patients with cervical injuries are the most prone to stress ulceration. High-dose steroid treatment may18 or may not63 increase the risk. Prophylactic treatment with antacids, H2-receptor antagonists, or sucralfate has been proven effective against stress-related mucosal damage.62,64 Maintenance of gastric pH >4 is associated with decreased incidence of GI hemorrhage.63 Antacids, such as magnesium hydroxide, aluminum, and aluminum-magnesium combinations, quickly neutralize gastric pH but have untoward side effects with the required dosing regimens, such as diarrhea, constipation, hypophosphatemia, and metabolic alkalosis.22,62 Intravenous H2-antagonists (cimetidine, ranitidine, famotidine) may be administered instead; for example, ranitidine, 50 mg IV q6h, reduced the risk of GI hemorrhage from 43 to 17%.64 Possible side effects of H2-antagonists include hypotension, thrombocytopenia, and inhibition of cytochrome P-450 enzyme system with subsequent interference with the hepatic metabolism of other drugs, including phenytoin, antibiotics, and warfarin.62,65 A third potential agent to consider is sucralfate, a mixture of sucrose, sulfates, and aluminum hydroxide that binds to gastric mucosa, increasing its viscosity, mucin content, and hydrophobicity.22,62,66 Beneficial effects of sucralfate, including stimulation of prostaglandin, protection of the mucosal proliferative zone, with facilitation of mucosal regeneration and healing, combined with no major adverse effects, make this agent an attractive choice for the prevention of stress ulceration.62 Indeed, sucralfate, 1 g PO/NG q6h, was found to be more effective that IV ranitidine, 50 mg IV q6h, in preventing GI ulceration or hemorrhage.67 Further, a meta-analysis study reports sucralfate to be as effective as antacids, absent the deleterious side effects, and slightly more protective than IV H2-antagonists administered in the prevention of clinical bleeding68 (Table 12.4).
Declining hematocrit and platelet levels on serial cell blood counts should raise the suspicion of GI hemorrhage, especially when no other etiology may account for blood loss. Elevations in blood urea nitrogen (BUN) with adequate urine output and stable creatinine may indicate a hemorrhage within the GI tract.63 In patients in whom a GI ulceration/hemorrhage is highly suspected, endoscopic evaluation is diagnostic and may provide hemostatic intervention. In recurrent or refractory GI bleeding, urgent surgical intervention is warranted.3,62
Table 12.4 Stress Ulceration Prophylactic Agents
Category | Example Agents | Effects |
---|---|---|
Antacids | Magnesium hydroxide | Maintain gastric pH >4.5 and inactivates pepsin |
Aluminum hydroxide | ||
Aluminum-magnesium combinations | ||
H2-antagonists | Cimetidine | Inhibit parietal cell, histamine |
Famotidine | type 2, receptors; ↓ acid production | |
Ranitidine | ||
Other | Sucralfate | Protects GI mucosa |
Abdominal and Bowel Dysfunction
The spectrum of bowel disorders in SCI patients ranges from acute life-threatening complications such as splenic rupture or liver laceration to more insidious issues such as bloating, bowel obstruction, constipation, fecal incontinence, and abdominal pain.60,61,69 Acute intra-abdominal problems should be identified by computed tomography of the abdomen and pelvis during the early assessment phase following SCI and managed accordingly.
Although vagal efferent innervation remains intact, loss of sympathetic outflow from the bowel often results in impaired colonic transit. Progression to ileus with concomitant constipation is not uncommon. If untreated, resultant fecal impaction may precipitate a more severe bowel obstruction with an associated distention and risk of perforation. Initiating a daily bowel regimen is paramount in the early phase of SCI. A standard regimen consists of a combination of stool softeners, rectal suppositories, and enemas.3 Paradoxically, although these agents improve colonic motility, looser and more frequent bowel movements may exacerbate the incidence of fecal incontinence, already manifest secondary to loss of sphincter control. Subsequently, supplying a source of fiber and maintaining adequate hydration are important considerations in the bowel regimen following SCI.69 Functional bowel disorders persist outside the window of ICU management, and have been found to be an unfortunate yet understandable source of anxiety and psychological distress.61,69 Therefore, early bowel retraining must be incorporated into the early management of SCI patients.
In injuries below C5, diaphragmatic irritation from abdominal distention may manifest as referred pain to the shoulder regions.3 Yet visceral pain may be perceived below the sensory level of injury, possibly secondary to an imbalance of sensory channels, loss of spinal inhibitory tone, or the presence of a central pain generator.70 Irrespective of the mechanism, elevated anxiety has been associated with SCI patients experiencing visceral pain symptoms.60
Urologic Considerations
Bladder hypotonia or flaccidity accompanies acute SCI during the period of spinal shock, lasting, on average, 3 weeks postinjury. Initial management involves the placement of an indwelling Foley catheter for accurate volume monitoring during hemodynamic stabilization and bladder decompression. A direct correlation between length of catheterization and risk of urinary tract infection (UTI) has been shown;71 however, evidence is lacking for support of prophylactic antibiotic treatment as catheter bacteriuria is common.71,72 The diagnosis of a UTI in SCI patients remains difficult as signs and symptoms may be occult due to loss of sensation. Clinical features may include fever, discomfort over the back or abdomen, increased urinary incontinence or spasticity, autonomic dysreflexia, malaise, and urine cloudiness or odor.31,71,72 Gram-negative bacilli (Escherichia coli, Pseudomonas, Klebsiella) and commensal organisms of the bowel (Enterococcus) are the predominating causative microorganisms of UTIs in SCI patients.31,71 Diagnosis should be confirmed with a urine culture. Empiric treatment may be started with broadspectrum antibiotics and then tailored once the microorganism is identified and susceptibilities are known.
Once medically stable, consideration of removing the indwelling catheter and initiating a bladder regimen may commence; usually this occurs beyond the time of initial ICU management and is done after transfer to SCI rehabilitation. Return of bladder function after spinal shock has resolved is dependent on the level of SCI. Most commonly observed in SCI patients, hypertonic bladder dysfunction occurs with injury above the sacral spinal cord and is categorized as upper motor neuron dysfunction. Resultant bladder-sphincter dyssynergia may lead to incomplete bladder emptying and progressively increasing urinary volumes and distention.22,73 Stimulated by excessive bladder distention, autonomic dysreflexia, a condition of massive sympathetic overdrive, may have life-threatening consequences. Symptoms of autonomic dysreflexia include sweating, headache, hypertension, and bradycardia.22 Detrussor areflexia, or hypocontractility, occurs following injuries that interrupt the local reflex arc and is the hallmark of lower motor neuron bladder dysfunction.73 Associated symptoms include saddle anesthesia, absent bulbocavernous reflex, and loss of both anal sphincter tone and control73 (Table 12.5).
Urodynamic studies may guide appropriate management regimens. Overall, intermittent, clean, straight catheterization is the treatment of choice because of lower risk for UTI.71–73 In a hypertonic bladder dysfunction regimen, goals include adequate drainage with low postvoid residuals, low-pressure storage, and low-pressure voiding. This can be accomplished numerous ways, including intermittent straight catheterization, external condom catheterization, functional electrical stimulation via an implanted device, volitional voiding, and anticholinergic pharmacologic agents such as oxybutynin and tolterodine tartrate.3,73 In detrussor areflexia, the goal is the prevention of overdistention, which may be accomplished with scheduled bladder emptying using abdominal pressure, the Credé maneuver, or intermittent straight catheterization.22,73 In either upper or lower motor neuron bladder dysfunction, long-term indwelling catheters are the choice of last resort, as they are associated with increased abnormal intravenous pyelograms, calculi, urethral lesions, leakage, and previously mentioned UTIs.3,31,71,73 Nonetheless, if necessary, indwelling catheters may be implemented through either transurethral or suprapubic placement.71,73
Table 12.5 Bladder Dysfunction Categories and Treatments
Category | Manifestation/Treatment |
---|---|
Upper motor neuron dysfunction | |
Complications | Ureteral reflux, hydronephrosis pyelonephritis, renal dysfunction |
Detrussor-sphincter dyssynergia (elevated bladder pressures) | Anticholinergics, α-antagonists, antihypertonics, transurethral sphincterotomy, urethral stent placement, functional electrical stimulation |
Detrussor hyperreflexia (urge incontinence) | Anticholinergics, afferent desensitization agents, functional electrical stimulation |
Lower motor neuron dysfunction | |
Complications | Saddle anesthesia, reduced anal sphincter tone, loss of sphincter control and bulbocavernous reflex |
Detrussor areflexia (overdistention) | Intermittent straight catheterization, chronic, indwelling catheter (treatment of last resort) |
Priapism following acute SCI in men is nonischemic but rather appears to be in consequence of the sympathectomy effects following cord interruption. Conservative management by means of observation may be preferred over intracorporeal phenylephrine irrigation without interference of long-term erectile function in patients who regain erectile function after SCI.74
Integument Considerations
Decubitus ulcers are an unfortunate yet frequent problem in SCI patients. Immobility, insensate integument, low blood pressure, and resultant decreased skin perfusion predispose the skin to necrosis and breakdown.3,22 For example, an SCI patient may develop decubiti even if left on a spine board for less than 6 hours.3 The level and completeness of the injury appear to be proportional to the risk of decubiti formation. The most common sites for decubiti include the ischial tuberosities, sacrum, lateral malleoli, greater trochanter, heels, and coccyx.3,22,31 Vigilant attentiveness to susceptible areas with frequent inspection and hygienic care is paramount in prevention, which remains the primary goal of treatment. Padding of pressure points, positioning, frequent turning with spine precautions as necessary, and utilizing air flotation beds constitute the mainstay of preventative measures currently employed.3,8,22 Management of pressure ulcers once formed involves immediate relief of pressure and wound débridement. Antibiotic therapy is indicated in the presence of infection or bacteremia. Bacteroides, E. coli, Proteus, Enterococcus, and anaerobic streptococci are most prevalent in decubiti associated with necrotic tissue; P. aeruginosa and S. aureus are more frequent isolates from healing ulcerations.31 Once formed, infection may result in cellulites, abscess formation, or osteomyelitis, with potentially catastrophic progression to bacteremia and sepsis.
References
3. Benzel EC. Spine Surgery. 2nd ed. Philadelphia: Elsevier Churchill Livingstone; 2005:512–571
8. Management of acute central spinal cord injuries. Neurosurgery 2002;50:S166-S172
13. George ER, Scholten DJ. Failure of methylprednisolone to improve the outcome of spinal cord injuries. Am Surg 1995;61:659–665
20. Greenberg MS. Handbook of Neurosurgery. 6th ed. New York: Thieme; 2006:702–707
21. Ball PA. Critical care in spinal cord injury. Spine 2001;26:S27-S30
22. Andrews BT. Intensive Care in Neurosurgery. New York: Thieme; 2003:137–141
28. Marino PL. The ICU Book. 2nd ed. Philadelphia: Lippincott Williams & Wilkins; 1998:421–448
51. Blood pressure management after acute spinal cord injury. Neurosurgery 2002;50(suppl):S58-S62
55. Nutritional support following spinal cord injury. Neurosurgery 2002;50(suppl):S81-S84
59. Cruse JM, Lewis RE, Dilioglu S, et al. Review of immune function, healing of pressure ulcers, and nutritional status in patients with spinal cord injury. J Spinal Cord Med 2000;23:129–135
65. Tryba M. Side effects of stress bleeding prophylaxis. Am J Med 1989;86:85–93
< div class='tao-gold-member'>
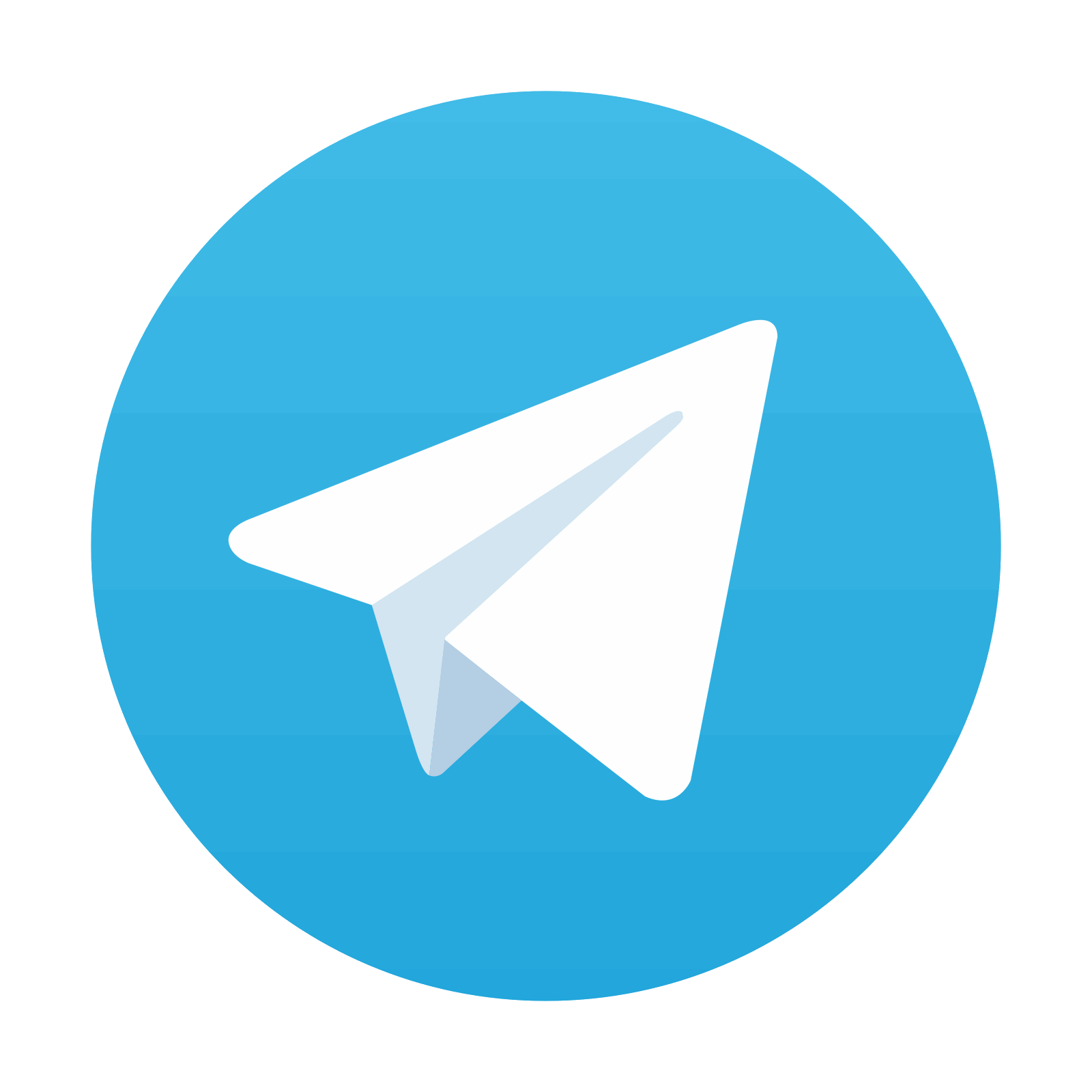