Congestive Heart Failure and Cor Pulmonale
Christopher I. Doty
CONGESTIVE HEART FAILURE
Congestive heart failure (CHF) is a complex clinical syndrome that represents a broad spectrum of disease stretching from stable pedal edema to fulminant cardiogenic shock. CHF can result from any structural or functional cardiac disorder that impairs the ability of the ventricle to fill with or eject blood. Ultimately, CHF is the inability of the heart to pump enough blood to meet the body’s needs. Often this manifests as increased left ventricular filling pressures. The common causes of CHF are listed in Table 83.1.
TABLE 83.1
Functional Classification and Causes of Left Heart Failure
Acute cardiogenic pulmonary edema or acute decompensated heart failure (ADHF) is characterized by the development of dyspnea associated with the rapid accumulation of fluid within the lungs’ interstitial and alveolar spaces, which is the result of these acutely elevated left ventricular filling pressures (1).
The burden of CHF morbidity and mortality for the US health care system is staggering with over 6 million patients in the United States alone. The yearly incidence is over 500,000 new patients, making CHF a very deadly disease (2). The prognosis after the onset of clinical signs and symptoms of CHF has not changed substantially over the last 15 years; the 1-year mortality rate for severely symptomatic CHF (class III or IV) is about 45% (3,4). A new diagnosis of CHF carries a 5-year mortality risk of approximately 50%, which is worse than most solid tumors (1).
CHF continues to be predominantly a disease of the elderly. It is estimated that 80% of patients who present to the emergency department (ED) with ADHF will be hospitalized, and repeat admissions are very common.
In CHF patients, there are three principal compensatory mechanisms that maintain systemic perfusion: (a) the Frank–Starling principle that relates preload to contractile force, (b) ventricular hypertrophy, and (c) central neural and peripheral neurohumoral responses to maintain systemic perfusion pressure and effective intravascular volume. These compensatory responses, unfortunately, are often maladaptive. These responses may further impede ejection of blood from the compromised ventricle. Therefore, manipulating the compensatory response to the fall in cardiac output (CO) (e.g., by the use of β-blockers, angiotensin-converting enzyme inhibitors [ACEIs]) has become a therapeutic focus in the management of chronic CHF (5).
The neurohumoral compensatory mechanisms for CHF actually result in an increase in systemic vascular resistance (SVR) and maintenance of effective intravascular volume through increased endogenous levels of biogenic amines, angiotensin, aldosterone, arginine vasopressin, B-type natriuretic peptide (BNP), prostaglandin E2, and prostacyclin. This increase in SVR and intravascular volume exacerbates the baseline pathology in CHF and worsen the patients symptoms. The pathophysiology of cardiogenic pulmonary edema is given in Figure 83.1.
FIGURE 83.1 Pathophysiology of cardiogenic pulmonary edema.
CLINICAL PRESENTATION
The clinical symptoms of CHF usually present through one of two mechanisms. The first is by increasing of hydrostatic pressures in capillary beds. Often, this is in the capillary beds of the lung where the increased hydrostatic pressures in the capillaries lead to transudation of fluid into the alveolar air spaces. This inhibits effective gas exchange and leads to the feeling of dyspnea and to hypoxia. This increased hydrostatic pressure may also present in the capillaries of the systemic circulation. This would be most evident in dependent areas and is usually manifested by pedal edema, or in severe cases, ascites.
The second mechanism of clinical symptoms is through a decrease in CO or forward flow from the heart. This leads to the classic signs and symptoms of a low flow state. These include weakness, altered mental status, hypertension, and easy fatigue. Often these two mechanisms will often present simultaneously.
Patients with left-sided heart failure (LHF) usually present with dyspnea and exercise intolerance, whereas patients with right-sided heart failure often present with peripheral edema and ascites. No single feature or physical examination finding reliably distinguishes patients with CHF resulting from systolic dysfunction (decreased ejection fraction) from those with diastolic dysfunction (increased left heart filling pressures and pulmonary capillary wedge pressures [PCWPs]). It is important to remember that only a little over half of CHF patients have a systolic dysfunction (6). Patients with systolic dysfunction often have increased LV filling pressures owing to a decrease in compliance and a decrease in ejection fraction.
Rales, one of the classic findings in heart failure, may also be found in pneumonia, interstitial lung disease, ARDS, or chronic obstructive lung disease. Wheezing, or “cardiac asthma,” is caused by interstitial edema and is common in ADHF. However, wheezing has poor sensitivity for CHF as it is present in many other diseases.
Acute pulmonary edema is LHF in its most extreme form and is a life-threatening emergency. Dyspnea and respiratory distress are the major presenting symptoms. A past history of heart failure has a specificity of 90% for the present dyspneic episode being caused by CHF, and patients with prior heart failure are approximately four times more likely to have ADHF when presenting to the ED with acute dyspnea (6). The typical patient is anxious, agitated, diaphoretic, in a tripod position, and will often appear as if they are in shock. There is usually hypertension caused by intense vasoconstriction, tachypnea hypoxia, and tachycardia. Clinical findings usually include (a) rales, (b) peripheral edema, (c) jugular venous distention, (d) a third heart sound, and (e) sometimes a fourth heart sound. Peripheral edema and hepatomegaly may not be seen when acute decompensation develops abruptly, unless it is superimposed on chronic fluid overload.
DIFFERENTIAL DIAGNOSIS
The differential diagnosis includes pneumonia, asthma exacerbation, cor pulmonale, chronic obstructive pulmonary disease exacerbation, pulmonary embolism, and other conditions that cause dyspnea. Volume-overloaded states such as renal failure, nephrotic syndrome, and hypoproteinemia (e.g., due to liver failure) may also mimic heart failure. Table 83.2 outlines conditions that may precipitate or worsen heart failure.
TABLE 83.2
Precipitating Causes of Heart Failure
ED EVALUATION
A thorough history and physical examination is the best diagnostic tool the emergency physician has in evaluating a patient for ADHF. ADHF often presents as a muddled picture in patients with the clinician having to weigh several diagnostic possibilities. However, there are several other diagnostic modalities that can help confirm the diagnosis. The chest plain radiograph (CXR) is readily available and often useful (7,8). Radiographic stages reflecting the severity of LHF have been described, but the classic radiographic progression is often not seen. In addition, the chest radiograph may not correlate temporally with the patient’s immediate condition; that is, there may be a lag of as much as 12 hours from the onset of CHF to the appearance of diagnostic changes on X-ray. Similarly, after treatment of LHF, there may be a lag of up to 4 days from clinical resolution of CHF until radiographic resolution. The specificity of the CXR for CHF (67% to 78%) is low enough that it cannot exclude left ventricular dysfunction; however, it can often indicate other diagnoses, such as pneumonia or COPD (8). These causes of dyspnea can have very different appearances from CHF on CXR.
The earliest radiographic finding of increased preload is redistribution or “cephalization” of blood flow. Normally, there is more blood flow to the dependent portions of the lungs. As PCWP increases, flow to the lower lung fields is reduced because of the vasoconstriction and the flow to the upper lung fields increases. Cephalization is best detected on an upright film and is most commonly seen in the setting of chronic CHF. As PCWP continues to rise, fluid accumulates in the interstitial spaces of the lung, producing Kerley B lines. Often the radiographic shadows of the pulmonary vessels begin to blur. As PCWP rises further, the increased hydrostatic pressure causes alveolar edema, and a classic butterfly pattern of bilateral perihilar infiltrates is seen on CXR. In almost all patients with chronic CHF, the cardiac silhouette is enlarged (cardiothoracic ratio >50%) and pleural effusions are common. The frequency of CXR findings that confirm CHF increases with the severity of diastolic dysfunction. A systematic review of the utility of CXR in the diagnosis of LV dysfunction concluded that redistribution and cardiomegaly were the best predictors of increased and reduced ejection, respectively (7).
The availability of point-of-care ultrasound is quickly becoming ubiquitous in EDs. This modality allows the physician to rapidly and repeatedly assess the dyspneic patient without sending the patient to the radiology suite nor exposing the patient to ionizing radiation. There is increasing literature about the reliability and the ease of using point-of-care ultrasound in the evaluation of ADHF patients. Sonographic evidence of interstitial edema called B-lines or “lung comets” has been shown to be very sensitive for CHF and even predictive of PCWP (9). Liteplo et al. showed that rapid bedside thoracic lung sonography had very good likelihood ratios (+OR = 4.7, –OR = 0.3) for predicting or eliminating CHF if examined in two lung zones bilaterally (10). This evaluative technique for lung sonography has been shown to be easy to learn and very reproducible for novice ultrasound practitioners (11).
The electrocardiogram (ECG), although of limited diagnostic value, should be performed on every ADHF patient to rule out acute coronary syndrome as a precipitating cause, which requires CCU admission and a more aggressive approach to treatment. However, chronic ischemic changes are very common and can make new ischemia difficult to diagnose. In chronic CHF, the ECG usually shows enlargement or hypertrophy of one or more chambers.
Arterial blood gas analysis reliably shows hypoxemia caused by impaired gas exchange, but it is of no value in diagnosis and of limited use in guiding therapy. Other blood tests show typical but nondiagnostic abnormalities (e.g., abnormal liver function tests caused by passive hepatic congestion or hyponatremia caused by of excess free water retention).
BNP and NT-proBNP levels correlate well with the presence and severity of heart failure (HF). BNP is secreted by the myocardium in response to increased wall stretch and an increase in LV end-diastolic pressure (preload), both of which are hallmarks of ADHF. Pre-pro BNP is synthesized within myocytes and is cleaved to proBNP. ProBNP is released into the circulation and then cleaved to the active BNP and an inactive N-terminal fragment, called NT-proBNP. The two peptides have a high degree of correlation (12). BNP is a compensatory peptide with venous, arterial, and coronary vasodilator properties but has no direct inotropic or chronotropic effects. Plasma BNP levels of <100 pg/dL and NT-proBNP levels of <300 pg/dL make HF highly unlikely; BNP levels >500 pg/dL and NT proBNP levels >1,000 pg/dL make HF very likely. In patients with a BNP level of 100 to 500 pg/mL, the baseline BNP level may be elevated owing to a stable underlying dysfunction, including right ventricular failure from cor pulmonale, acute pulmonary embolism, or renal failure. BNP level has been shown to be affected by age, gender, drug use, and several comorbidities such as liver cirrhosis or primary pulmonary hypertension. Conversely, BNP levels are lower than expected in flash pulmonary edema, obesity, and CHF secondary to mitral valve dysfunction. BNP levels should not, therefore, be used as the sole diagnostic tool in assessing patients with suspected heart failure (2). A plasma BNP >100 pg/mL diagnosed HF with a sensitivity and specificity of 90% and 76%, respectively (12,13).
Data from the BNP Multinational Study indicate that BNP levels can be used to improve diagnostic accuracy beyond clinical judgment. A rapid BNP immunoassay test is currently available and has been shown to be better than the clinical examination in detecting CHF in ED patients and identifying CHF as the etiology of dyspnea of unclear etiology (13). Coupling thoracic sonography with NT-proBNP has shown to increase the physician’s diagnostic accuracy for ADHF tremendously (10). However, as BNP will not add significant diagnostic value in patients who have clear-cut findings, the test should not be used when the clinician has a very high or a very low pretest probability of ADHF.
Cardiac enzymes, including troponin, CBC, and a complete metabolic profile should be obtained in patients presenting with ADHF. Other testing may be indicated depending on the patient’s medications and the patients past medical history.
KEY TESTING
• Chest radiograph
• EKG
• Transthoracic lung ultrasound
• Transthoracic echo
• NT-proBNP or BNP if unclear cause of dyspnea
• Troponin (I or T)
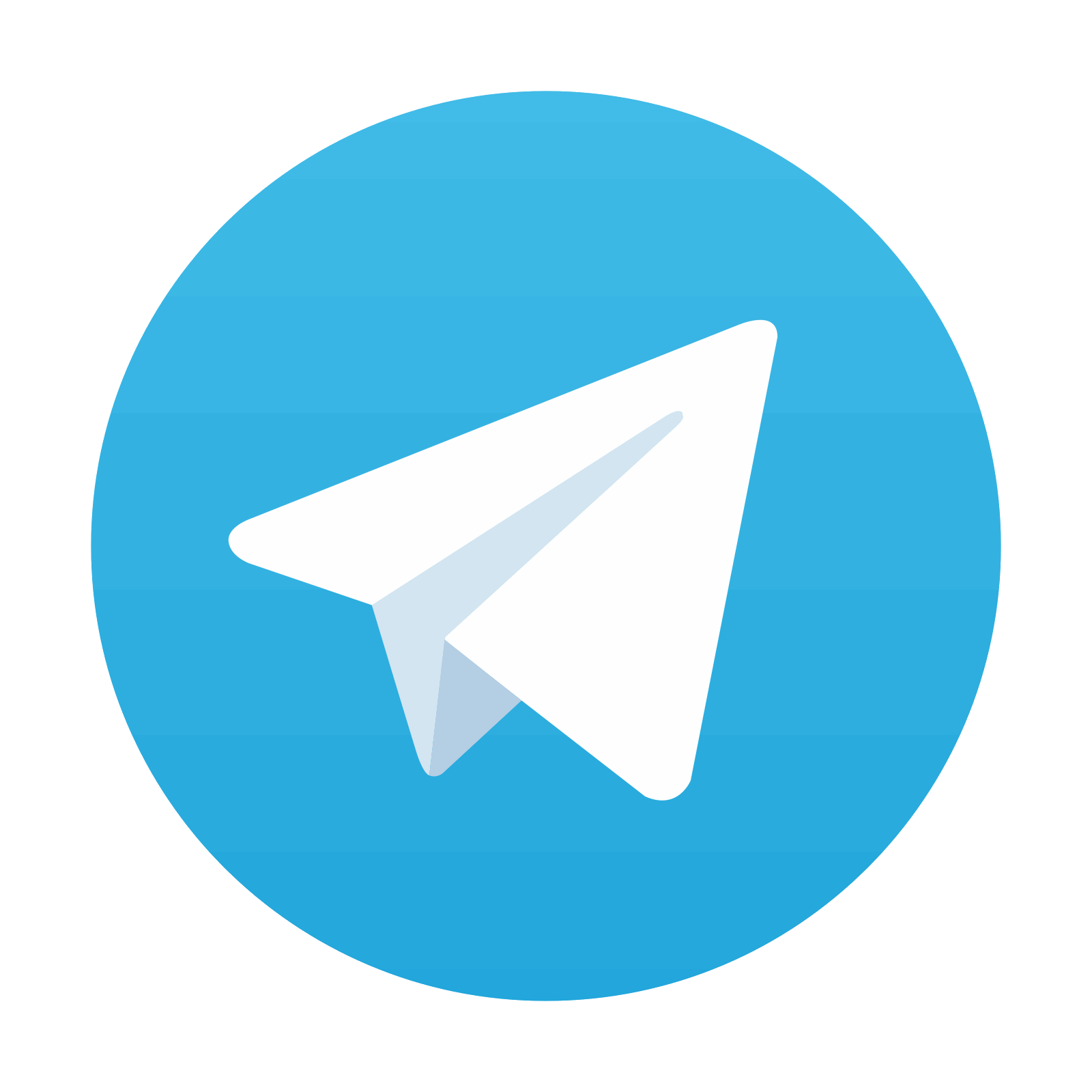