Fig. 35.1
Generic closed-loop scheme
The most ubiquitous controller is the Proportional-Integral-Derivative (PID) controller [11]. This controller calculates the difference between the measured output and the setpoint to adjust input value. The algorithm takes into account the present (proportional), past (integral), and future (derivative) error to adjust the correction. The artificial neural network controller is an adaptive control algorithm with a dynamic learning strategy [12]. The adaptive model-based controller [13] approximates or simulates the target system and predicts the observed response. The relationship between the propofol effect site concentration and the BIS value is determined during the induction phase and is used to construct patient-specific pharmacokinetic (PK) and pharmacodynamic (PD) models. This approach was abandoned later in favor of a Bayesian approach that optimizes the model [14]. The rule-based controller uses a set of instructions to perform its actions. A fuzzy logic controller is a system wherein the logical values true or false are not necessarily accurate but may be affected to some degree of truth. Fuzzy logic allows the imprecise information that humans use in decision-making to be formalized. This system requires testing because of the possible arbitrariness within sets. All these algorithms can be combined with each other (cascade structure) to create a specific controller to modify drug infusion rates or drug concentrations using a target-control-infusion (TCI) system.
The evaluation of the controller’s performances by simulation or “in silico” studies is the first step before clinical evaluation. But, the relationship between performance obtained during simulation studies and anesthetized surgical patients is weak. Simulation studies can only determine whether the controller follows the instructions encoded in the software program [15]. It cannot always prove that the actions are logical or appropriate in anesthetized patients, many actions and results are context and time-dependent. For example in a surgical patient, a 20 mg bolus of propofol alone has virtually no effect before induction of anesthesia. After induction and during the maintenance phase, the same bolus can a significant decline in the BIS value, whereas during surgical noxious stimulation the same bolus may be inadequate so the BIS value increase despite it. Finally, controller performance can only be assessed by clinical studies because the transfer function remains unknown. In Table 35.1 we list the definitions of the most important terms used in control engineering.
Table 35.1
Definitions of engineering control terminology
Input | The variable entered into a function that modifies the output. In medical closed-loop systems, the inputs are the interventions of the controller (drug administration, for example) |
Output | The end result of the input. In medical closed-loop systems, the outputs are the monitored patient parameters (heart rate and blood pressure, for example) |
Open loop | A system wherein the measured output has no impact on the inputs |
Closed-loop | A system wherein the measured output is used by a controller to determine a new input to the system. The controller which closes the loop could be manual or automated |
Setpoint | Set point preset value that the control system is supposed to target |
Error | The difference between the setpoint and the measured output value |
PID | Proportional-integral-derivative controller. The three terms account for the present, past, and future error |
Model-based | A model that approximates or simulates the target system |
Rule-based | A set of defined rules organize and execute specific actions according to output changes |
Artificial neural networks | An adaptive control algorithm with a dynamic learning strategy |
Fuzzy logic | Systems wherein the logical values true or false are not exact and degrees of truth are permitted |
Automated Intravenous Anesthesia with a Single Controller in Adult Patients
This chapter will discuss different clinical studies of a single automated controller. In particular, it will highlight randomized controlled trials but also describe observational studies.
Randomized Controlled Studies of BIS-Propofol Controllers During General Anesthesia
Since the first clinical observational study of automated titration of anesthesia using the EEG as output [1], various signals such as the median frequency of the EEG power spectrum [16] and auditory evoked potentials [17] have been used to guided automated titration of methohexital [16], propofol [13, 17], or alfentanil [18]. The first randomized study reported for the use of the BIS monitor as output of automated anesthesia was performed in Hong-Kong with the collaboration of a team from New Zealand [19]. In this randomized control trial, 30 female ASA I-II patients were enrolled. In the automated group, anesthesia induction was performed manually using a mixture of propofol and alfentanil followed by a bolus of vecuronium. After tracheal intubation, the anesthesia was maintained by automated infusion of a propofol/alfentanil mixture with a setpoint of 50 for the BIS. The performance of this PID controller was similar to the manual administration of the mixture to maintain the BIS at the setpoint. No clinical advantage was found and light anesthesia episodes were more common in the closed-loop group probably related to the fact that the propofol/alfentanil ratio was fixed. This was, however, the first randomized study which demonstrated the clinical feasibility of automated intravenous titration of anesthesia guided by the BIS.
Different studies have reported the feasibility of automated propofol administration guided by the BIS during maintenance, but not induction of general anesthesia [19–21]. The induction phase is considered as a busy period during general anesthesia because the clinician needs to simultaneously control the airway, monitor and maintain hemodynamic and titrate anesthesia. A PID controller which controlled the calculated effect site concentration with the model of Schnider [22] was evaluated in a randomized control study of 40 patients specifically during the induction phase [23]. The control group received manual titration of propofol using a TCI with the model of Marsh [24]. In both groups the target BIS was 50 and a continuous infusion of remifentanil was administered. Automated induction titration of general anesthesia decreased the BIS overshoot (i.e., propofol overdosing), and reduced the mean duration of induction, whereas hemodynamic stability and propofol consumptions were similar between the two groups. This study, which also included patients ASA III status and those taking cardiovascular medications, was the first reported of automated induction guided by the BIS. The same PID controller was used for the automated titration during induction and maintenance of general anesthesia [25]. This randomized controlled trial was performed in three centers, 83 patients were included in the automated group and remifentanil TCI was adjusted manually in both groups. The goal of the automated controller was to reach a BIS target of 50 and to maintain it in the range 40–60 which was considered to represent adequate anesthesia. During induction, the automated controller decreased propofol consumption but induction duration was longer. The mean duration of automated propofol titration was 134 min, the percentage of time of adequate anesthesia was higher with the decrease of deep anesthesia (BIS value <40) or light anesthesia (BIS > 60) during maintenance of general anesthesia. The incidences of unwanted somatic events or hemodynamic instability were similar and the time from discontinuation of propofol to tracheal extubation was shorter in the automated group. In contrast to previous studies [19–21], automated titration of propofol was performed during induction and maintenance of general anesthesia and patients undergoing major surgery, those with ASA III or worse and the elderly were not excluded. There are several physicians and anesthesia nurses who have used the controller in different centers, which demonstrates the applicability and reproducibility of the method.
Another PID controller was developed and evaluated in a study performed in India [26]. This randomized controlled study included 20 patients ASA I-II patients per group; in both groups, patients received an initial bolus of fentanyl followed by a continuous infusion. The automated controller administered less propofol and caused less overshoot of the BIS during induction. During maintenance, the automated controller decreased propofol consumption, outperformed manual titration for maintaining the BIS in the range 40–60, and facilitated earlier tracheal extubation was shorter. The same system has been used successfully in various clinical situations [26–31]. Indeed, all these studies were evaluated in the same center with the same investigators. To demonstrate the applicability and the reproducibility of the system, the authors performed a new controlled study involving multiple investigators in different centers [6]. In this randomized controlled trial the authors describe the comparison between automated and manual propofol administration guided by the BIS. This trial was performed on 242 patients undergoing elective surgery in six centers. The single closed-loop controller allowed the automated titration of propofol during induction and maintenance of GA. The automated controller outperformed a manual control to maintain the BIS around the setpoint of 50 and to maintain the heart rate and mean arterial pressure within 25 % of the baseline. The authors succeeded in delivering propofol automatically during induction and maintenance in realistic conditions.
A rule-based adaptive controller has been developed to steer propofol guided by the BIS [32]. In a controlled trial 20 ASA I-III patients, aged 54 ± 20 years, undergoing minor and major surgeries were included in the automated group. In both groups fentanyl or rocuronium boluses and propofol for induction were administered manually. The setpoint for the controller was 45 for the BIS which was deeper than in some previous studies [19, 23, 25, 26]. Propofol consumption was similar between manual and automated administration of propofol. The automated system was better able to maintain a target of BIS 45 than manual administration. Tracheal extubation was possible sooner in the automated group. Electromyogram activity measured by the monitor was integrated into the algorithm allowing the detection of artifacts.
The current trend is to evaluate automated systems in multicenter trials is to perform multicenter trial including a significant number of patients to improve the applicability and reproducibility of the results and decrease the risk of fragility of the results.
Observational Studies of BIS-Propofol Controllers During General Anesthesia
Observational clinical studies are necessary initially to assess the stability of the controller in realistic conditions such as in the operating room and to determine the proof of concept.
A study performed on female patients evaluated an adaptive model-based controller [20]. In this study a control group was available but the propofol was administered by a continuous infusion according to clinical criteria without the use of a brain monitor and therefore the control group was not considered as useful [33]. The initial patient-specific pharmacodynamic parameters were calculated during induction with a heuristic controller which had previously been used for automated sedation [34]. Propofol target concentration was increased by 0.5 μg/ml every 50 s. Once the BIS target had achieved, the system was switched to automatic feedback control, using the model parameter calculated during induction. During feedback control, the controller minimized the difference between the measured BIS and desired effect site concentration. All subsequent adjustments in propofol administration were based on the sigmoid “Hill” curve estimation. A total of 10 ASA I-II patients per group undergoing open hysterectomy were included, a continuous remifentanil infusion was administered before intubation (0.50 μg/kg/min) and during the maintenance (0.25 μg/kg/min). A bolus of rocuronium was used to facilitate tracheal intubation in both groups. During a mean duration of 113 min in the automated control group, the BIS values were better controlled using the automated controller and this method was reported to be clinically acceptable. However, the induction curve probably cannot always be obtained and this controller cannot be used in already anesthetized patients. The adaptive model-based-controller was finally improved by Bayesian optimization which overcomes the previous limitation [35]. Using a simulation protocol, all parameters of model-based adaptive controller were adjusted using specific weight calculated by the Bayesian variances. These variances determine how the model-based parameters can deviate from the population model. This algorithm was tested clinically for the automated sedation of propofol [14].
A PID controller previously tested for automated sedation of patients also receiving lumbar epidural analgesia [36] was evaluated in 20 patients undergoing body surface surgery [21]. The initial controller has a cascade structure including a PID controller which modifies target of plasma propofol concentration. In this second study the controller was modified to target the calculated effect site concentration to reduce the problem of oscillation related to the delay in equilibration between the plasma and effect site compartment. Manual induction was performed using a TCI system of propofol and remifentanil to target loss of consciousness and to tolerate the insertion of the laryngeal mask airway. The remifentanil concentration was fixed at 4 ng/ml during the maintenance phase of anesthesia. The median duration of automated administration of propofol was >27 min, the median BIS setpoint for the controller was 50 and this setpoint varied between 29 and 79. Anesthesia conditions were satisfactory for all patients except one patient who moved after the remifentanil was switched off by error. This study highlighted that targeting the effect site concentration by the controller was associated with fewer BIS oscillations.
In contrast to a PID controller , a neural network controller takes into account the nonlinearity, time variance or hysteresis, asymmetric control, delay of peak effect and uncertainty of the biological systems [12]. A comparison between a PID controller and a neural network controller using as a reinforcement learning algorithm was performed in silico [12]. In this simulated study reinforcement learning had the potential to maintain the BIS more accurately than a PID controller. Unfortunately, the relationship between controller performances during an in silico study and performance in an anesthetized patient is unknown. A neural network adaptive controller for propofol guided by the BIS has been developed and evaluated clinically [37]. This controller was tested in seven patients and was able to effectively administer propofol for induction and maintenance anesthesia during non-cardiac surgery. However, the authors reported BIS oscillation related to electromyographic noise in the BIS signal.
An adaptive proportional integral BIS control algorithm for propofol administration was developed and tested in 14 patients (30–60 years) [38]. The algorithm takes into account the present error between the setpoint and the desired BIS value and the past error. Anesthesia was induced by manual administration of propofol, remifentanil , and rocuronium. The authors reported that BIS oscillation during subsequent automatic control was probably related to the hysteresis between the infusion rate and the peak of the clinical effect. The addition of dead time or dead-time compensation after each propofol modification attenuated the BIS.
A disadvantage of these different single BIS-propofol controllers is that they only control the hypnotic component of anesthesia and the analgesic component, whereas the level of noxious stimulation varies continuously throughout surgery. During an intense noxious stimulus a single feedback propofol controller would respond by increasing the propofol to maintain the BIS at the setpoint, whereas the more appropriate action is to increase the analgesic component. Thus, tests of single propofol controller performance are in reality strongly influenced by the quality and the adequacy of analgesia policy.
Automated Intravenous Analgesia During General Anesthesia
The automated titration of opioid administration is the main challenge currently in the development of closed-loop loop anesthesia systems . At present, there is no specific parameter to directly measure analgesia in an anesthetized patient. Opioids are known to suppress the hemodynamic responses to noxious stimulation but even high opioid concentrations cannot guarantee complete control of hemodynamic responses to surgical stimulation. In clinical practice, opioid administration is titrated to hemodynamic changes after noxious stimulation which reveals the depth of antinociception.
Hemodynamic changes have been used for automated administration of alfentanil [39]. In an observational study including 11 patients, an alfentanil infusion was automatically titrated with a setpoint of mean arterial blood pressure of 70 mmHg obtained by an invasive measurement method. The controller calculated the plasma alfentanil concentration and made adjustments on the basis of a model-based predictive control algorithm. Isoflurane was titrated manually during the maintenance of anesthesia guided by the BIS. This study demonstrated the feasibility of the controller in healthy patients, during minor surgeries and with invasive mean arterial blood pressure.
By using noninvasive mean arterial blood pressure and heart rate, the Analgoscore, a score of nociception was developed allowing the automated titration of remifentanil [40]. The algorithm calculated a score related to the difference between the measured hemodynamic data and the setpoints, according to which the infusion rate of remifentanil was modified. Depending on the type of surgery and the patient’s co-morbidities the clinician defined target values for mean arterial pressure and heart rate. Automated titration of remifentanil was performed in 16 patients and compared to 11 patients for whom the infusion rate of remifentanil was modified manually depending on the score given by the controller. The automated controller outperformed skilled manual titration to maintain the hemodynamic score in the desired range. This observational study reported the feasibility of this controller during minor surgeries in a limited number of healthy patients.
There are certain limitations to the use of hemodynamic changes as output for a control system. Heart rate, mean arterial pressure, and heart variability changes are not specific to analgesia needs and so are not optimal for analgesia titration. Hemodynamic status and responses can be modified by chronic hypertension, various treatments, blood loss, fluid administration, heart failure, arrhythmia, manipulation of great vessels, and a variety of drugs (inhalational and intravenous anesthetics, vasopressors, β blockers, vasodilators, etc.). An alternative to hemodynamic criteria for control of opioid administration is titration according to changes in electrocortical activity after noxious stimuli [41].
The median EEG frequency was used in 11 patients to steer alfentanil administration [18] and more recently the BIS has been used as output for a controller to steer a fixed mixture of propofol and alfentanil [19]. This latter randomized controlled trial reported no clinical advantage between automated and manual control. Use of an isoboller controller has also been described in which propofol and fentanyl administration was based on the mild-latency auditory evoked potential and a fuzzy logic algorithm that combined heart rate and mean arterial pressure, but it was tested on only one dog [42]. Currently, one of the most frequently published methods for the automated titration of remifentanil is the use of the BIS as output combined with the automated titration of propofol [43, 44]. The description of these studies will be further developed in the next section.
Multiple Automated Intravenous Controllers in Anesthesia
General anesthesia is a dynamic balance between hypnosis, analgesia, and muscle relaxation. Currently, several prototypes for the automated titration of propofol are available. The clinical relevance of automated administration of neuromuscular blocking agents is limited since the introduction of Sugammadex a specific antidote of rocuronium [45]. However, a study has reported the feasibility of combining automated titration of propofol and mivacurium [46]. In this observational study including 20 patients, the propofol was titrated automatically using a fuzzy logic-PID controller during the maintenance of general anesthesia. The control variable was the BIS, with a setpoint of 40 which was lower than in many previous studies [19–21, 25–28, 32, 35, 36]. The automated administration of mivacurium was started after tracheal intubation and neuromuscular blockade was guided by single twitch stimulation of the ulnar nerve. The setpoint for the neuromuscular controller was a train-of-four ratio of less than 10 %. Analgesia was maintained by a continuous infusion of remifentanil (0.25 μg/kg/min). No manual intervention was necessary during automated administration and operating conditions were satisfactory for all patients. The same propofol controller was combined with automated titration of a remifentanil TCI [47]. The fuzzy logic controller has a cascade structure for the automated titration of the target concentration of a remifentanil TCI. Heart rate variability, heart rate, and noninvasive blood pressure were used as output for the controller. Two fuzzy systems were implemented: one system can decrease the infusion rate during bradycardia or hypotensive episodes, the second system adjusts remifentanil administration according to tachycardia, mean arterial pressure, and heart rate variation. This controller was tested in ten patients during orthopedic surgery, was able to maintain satisfactory intraoperative conditions, and remifentanil was administered with adequate precision [47].
Recently, a randomized controlled trial reported the use of a commercial device allowing the automated titration of propofol and rocuronium during maintenance of general anesthesia [4]. In the automated group, 89 ASA I-II patients were included in three centers. The controller collected and calculated a mean BIS value during 3 min. An algorithm then used, the calculated BIS values to control the target plasma propofol concentration using the PK model of Marsh [24]. The plasma remifentanil concentration was calculated using the PK model of Minto [41] and was titrated manually in both groups. For feedback control of rocuronium, the ulnar nerve at the wrist was stimulated every 20 s to obtain the train-of-four ratio. This study demonstrated that this new closed-loop infusion system could automatically regulate propofol and rocuronium. The automated controller achieved adequate anesthesia more accurately than manual control but no clinical advantage was found.
A controller integrating the three components of general anesthesia was evaluated and validated clinically [48]. The controller included a rule-based adaptive algorithm for the automated titration of propofol with a setpoint of 45 for the BIS value [32]. A pain score or Analgoscore® was calculated according to heart rate and mean arterial pressure and an expert-based rules algorithm allowing the automated titration of remifentanil administration [40]. The train-of-four ratio was measured every 15 min at the adductor pollicis muscle and rocuronium boluses were administered by the controller to maintain the train-of-four ratio of 0.25. A total of 186 patients were randomized and 93 ASA I-III patients were included in the automated group. This study demonstrated the feasibility of simultaneous control of hypnosis, analgesia, and muscle relaxation. The automated controller was better able to maintain the BIS around the setpoint and to maintain a target pain score and extubation time was faster than in the manual control group.
Hemodynamic changes during surgery are not always specific to analgesia and for the calculation of the pain score, the investigators need to determine the correct setpoint of heart rate and mean arterial pressure and modify the setpoint during the procedure [40]. The lack of specificity or setpoint for hemodynamic criteria during surgical procedure has given rise to other criteria. Noxious stimulus may cause cortical electrical activation with an increase of BIS values [49, 50], which indirectly reflect the level of antinociception [51]. In a randomized study including four centers, the BIS was used as output for the automated administration of propofol [25] but also for the second controller which was implemented for the automated titration of remifentanil [43]. The principle of this controller was based on the assumption that rapid BIS increase during a surgical procedure is likely to be secondary to noxious stimulation and is related to inadequate antinociception. A total of 196 patients were included in this trial and in 83 patients a PID controller with a setpoint of 50 was evaluated. The controller automatically administered both propofol and remifentanil during induction and maintenance of general anesthesia. In the automatic group, adequate anesthesia (BIS in the range 40–60) was present for a greater proportion of time, the incidence and duration period of excessively deep anesthesia and burst suppression ratio was decreased and the time to tracheal extubation was shorter than in the manual control group. However, remifentanil consumption was greater with the automated controller.
Several other clinical studies have confirmed that analyses of EEG activity can be a reasonable surrogate measure of depth of antinociception. One study involved 1494 ASA I-III patients in whom the dual controller was used [44]. In this study the initial dual PID controller [43] was also modified allowing a decrease of remifentanil administration by 20 %. The decrease of remifentanil administration by the automated controller was confirmed by another prospective study including more than 600 ASA I-III patients [52]. That these studies [43, 44, 52] were multi-centers and with many investigators supported the applicability of the controller in hands other than that of the inventors.
Previously a dual PID controller [43] used the M-Entropy analysis (GE Healthcare, Helsinki, Finland) of the EEG as output for the automated co-administration of propofol and remifentanil [7]. This randomized study reported that State Entropy can steer the automated administration of propofol, and that the difference between Response and State Entropy was a surrogate measure of depth of antinociception that can be used to steer the automated administration of remifentanil . Thirty patients were assigned to the automated group, for whom the automated controller was able to provide induction and maintenance of general anesthesia, with adequate anesthesia for a greater percentage of time , where adequate anesthesia is defined by a State Entropy value in the range 40–60. Propofol and remifentanil consumption and the incidence of hemodynamic or somatic events were similar between the two groups.
Controlled clinical studies for multiple controllers have now been performed [4, 43, 48] and the automated co-administration of propofol and remifentanil appears to be the most clinically relevant approach [43, 48]. Further evaluations are needed to determine whether hemodynamic changes or cortical electrical activation can be the most robust parameter for the automated titration of analgesia.
Automated Intravenous Sedation
Application for Surgery
The first study of automated sedation guided by the BIS was reported in Belgium [19]. It was an observational study including ten patients undergoing orthopedic surgery under spinal anesthesia. Initially, propofol administration was titrated manually to target an Observer Assessment of Alertness and Sedation scale rating 1. After that the current BIS value was used as setpoint for the automated controller. During automated sedation no episode of apnea or hypoxemia with SpO2 below 90 was observed. Some patients exhibited movements but no case of awareness was reported. Automated sedation was performed using a model-based adaptive controller, for a mean duration of 29 min with a maintenance dose of propofol 5.2 ± 2.7 mg/kg/h.
A PID controller initially developed for use with an auditory evoked potential monitor [17] was modified by the use of the BIS monitor instead as output allowing the feedback control of plasmatic propofol concentration [36]. The controller was evaluated in ten patients undergoing hip and knee surgery under combined epidural analgesia and sedation during a mean duration of 72 min. A lumbar epidural catheter was initiate with bupivacaine before the start of sedation. The plasma target propofol concentration was manually titrated to allow the insertion of the laryngeal mask airway and the patient breathed spontaneously. This observational study reported that the performance of the controller for different BIS setpoints (mean setpoint value was 48) was adequate and that it was able to provide clinically adequate sedation in nine patients. However, one patient moved 10 min before the end of the procedure after a noxious stimulus and in three patients BIS oscillations were observed. BIS oscillations were related to the time taken for equilibration between the calculated plasma concentration and the effect measured by the BIS. To take into account of this hysteresis between plasmatic and effect site concentration the controller was modified to steer the effect site concentration instead of the plasma concentration [21].
A model-based adaptive controller [20] was modified by Bayesian optimization [35] allowing the automated titration of propofol during induction and maintenance of sedation. This Bayesian-based controller was evaluated in 20 female patients undergoing ovarian puncture for infertility [14]. The controller continuously modified the parameters of a specific sigmoid model for the effect of propofol, and used the model to select appropriate target concentration to minimize the difference between measured BIS and the setpoint. Before the start of automated intravenous propofol administration, boluses of midazolam and alfentanil were administered. The BIS setpoint was 50 and patients maintained spontaneous ventilation during 17 min. Ability of the automated controller to maintain the BIS in the range 40–60 and to maintain cardiopulmonary stability was similar to manual control.
A new controller was developed for automated sedation of patients under spinal anesthesia [53]. This randomized controlled trial included 75 ASA I-III patients undergoing elective orthopedic lower limb surgery. An initial bolus of propofol was injected by the controller at a rate of 250 μg/kg over a period of 2 min. Afterward, an infusion rate of 50 μg/kg/min was given for 3 min and the automated maintenance sedation was started. The BIS setpoint was 65 for the automated titration of propofol and the controller included a Decision Support System which decreased automatically propofol infusion by 50 % when low respiratory rate (<8 per min) or peripheral oxygen saturation (<92 %) was detected for more than 2 min. In the automatic group, “Excellent” (BIS value 65 ± 10 %) or “Good” sedation (BIS value 65 ± 20 %) was present for a greater proportion of time.
Application for Colonoscopy
The effectiveness of a PID controller developed for sedation during surgery [36] was evaluated for sedation during colonoscopy. In an observational study automated titration of propofol for sedation was performed during maintenance in 16 adult patients ASA I-III undergoing colonoscopy. The propofol was titrated manually using a TCI system until an Observer Assessment of Alertness/Sedation rating of 3 was reached (Response only if name called loudly or repeatedly). At this point automatic control was started with the current BIS value as the setpoint for the controller [54]. The median [range] duration of automated propofol administration was 19 [7–50] min, the median setpoint for the BIS value was 80 [75–85]. The controller maintained the BIS values within 10 % of setpoint during 80 % of the maintenance duration. No episodes of apnea, hypoxemia, excessively deep sedation, or airway obstruction requiring airway support were observed. Four patients reported recall without dissatisfaction and all the endoscopists were satisfied. This study demonstrated that a PID controller combined with TCI system and the BIS allowed the automated titration of propofol during colonoscopy.
Another EEG monitor, the NeuroSense™ has been used in pediatric and adolescent patients for the automated induction and the maintenance of propofol sedation [55].
Probably the most interesting system has been the development and Food and Drug Administration approval of a commercial device: the Sedasys® (Johnson & Johnson, Los Angeles, California, USA). Sedasys® received premarket approval in 2013. This device designed for non-anesthesiologist use, to facilitate the intravenous administration of 1 % propofol [56] for intravenous sedation of adult ASA physical status I or II patients undergoing colonoscopy and esophagogastroduodenoscopy. The device continually monitors and records patient’s vital signs, including oxygen saturation, respiratory rate, heart rate, noninvasive blood pressure, end-tidal carbon dioxide and patient responsiveness. The controller can automatically increase oxygen delivery when oxygen desaturation is detected, and can decrease or stop propofol administration when the controller detects signs of oversedation (oxygen desaturation, low respiratory rate/apnea or unresponsiveness to auditory prompts). However, while the user (healthcare provider) can set the system to provide additional propofol, the system itself cannot initiate an increase in infusion rate or any additional dosing of propofol at any time. An initial evaluation of this device was performed in 48 patients in America and Belgium and the study demonstrated the proof of the concept [57]. In a randomized controlled multicenter study including 1000 patients the controller was compared to a control group [3]. After a bolus of fentanyl, the propofol was administered continuously until minimal to moderate sedation was achieved, and then the controller was started. The Sedasys® decreases the occurrence of desaturation as compared to manually administered sedation with midazolam and meperidine or fentanyl for moderate sedation for routine colonoscopy and is associated with faster recovery from sedation [3]. The Sedasys® enabled effective and safe propofol administration by non-anesthesia professionals. Those using this device are required to first undergo full training to manage the potential adverse cardiopulmonary events related to propofol. Also, an anesthesiologist must always be immediately available for clinical assistance. However, Johnson & Johnson has stopped selling the device in March 2016 related to unexpectedly slow sales and company-wide-cost-cutting (there were no safety concerns).
Application for Intensive Care Units
Sedation is an integral part of patient therapy in the intensive care unit (ICU). Mechanically ventilated patients require sedation to improve comfort, to prevent metabolic imbalance, psychological stress or post-traumatic disorders, to decrease pain in the postoperative period or during nursing procedure [58, 59]. Inadequate sedation is related to specific morbidities such as nosocomial pneumonia and withdrawal syndrome, and it also prolongs mechanical ventilation and ICU length of stay [60–62]. The amount of sedation required by instable ICU patients is related to the underlying illness and co-morbidities. It changes over time and there is a high variability of PD response to the drugs. ICU physicians and nurses have many specific responsibilities and the time allocated to sedation titration is still limited and as opposed to the operating room, there is not a dedicated anesthesiologist for administration of sedative agents. Thus, closed-loop or automated sedation has the potential to improve patient care by continuously adjusting the dosage of sedative agents to the minimum required for efficacy, thereby potentially avoiding side effects associated with overdosage. Moreover, appropriate sedation also prevents drug accumulation which facilitates neurological assessment.
In ICU patients EEG activity provides an evaluation of sedation depth. The EEG analysis given by the BIS has also been used to guide sedation in critical care patients [63, 64]. The BIS has been empirically demonstrated to correlate with behavioral measures of sedation [65]. However, there is some controversial literature about BIS in ICU patients, related to a poor agreement between BIS index and subjective sedation scales [66, 67]. In fact BIS measures deep sedation levels (BIS < 60) more accurately than the Ramsay scale which attributes a value of 5 or 6 [68]. A BIS target between 40 and 60 thus seems to be a surrogate measurement of deep sedation in the ICU [69] and is commonly targeted. This setpoint has the further advantage of decreasing the impact of muscular activity on BIS [70] and improves agreement between BIS values and sedation scales [71–73].
The feasibility of automated ICU sedation guided by EEG activity was reported in 1999 [74]. A quantitative measure of EEG activity (median frequency of the EEG power spectrum) was used for the automated titration of propofol in 21 ICU ventilated patients. The use of a BIS monitor as output of an automated controller for postoperative sedation was reported in patients admitted to a surgical ICU after cardiac surgery [31]. In this randomized controlled study, the controller targeted a BIS value of 70 and demonstrated the feasibility of a closed-loop propofol controller guided by BIS for sedation maintenance following cardiac surgery. The authors reported a high rate of modifications per hour (above 30 per hour) required to maintain the BIS in the range but no sparing effect of propofol was found. The controller has also been validated in a pediatric cardiac surgery patient [30].
The benefit of implementing a neural network controller in an automated sedation system was suggested but never reported in ICU patients [37]. In a randomized controlled trial, our controller for the automated titration of propofol and remifentanil [43] was compared with manual control of deep sedation in ICU patients [75]. Propofol and remifentanil were guided either manually or by a computer to maintain the BIS value in the range 40–60 considered as deep sedation in the ICU. Patients were severely ill in both groups as described by the usual critical illness scores and the controller significantly outperformed manual control. The closed-loop system maintained BIS in the range 40–60 or adequate sedation for 77 % [59–82] of the total duration in compared to 36 % [22–56] in the manual group. The median duration of automated sedation was 18 h [8–24]. No adverse events were recorded during the study. Propofol consumption was 0.8 mg/kg/h, this was reduced by twofold in the automated group and automated sedation decreased the sedation drug costs. The propofol consumption was lower than that reported for sedation in cardiac surgery [31] while the setpoint was higher for the controller (BIS = 70) probably related to synergic effect with remifentanil and lower than reported during maintenance of general anesthesia [26, 43].
Reduction in propofol administration may protect patients from the risk of propofol infusion syndrome as previously described in children and more specifically in the ICU. Propofol infusion syndrome was reported in patients receiving more than 5 mg/kg/h for a prolonged period. We note a safe administration of propofol as the total regimen ranged between 0.8 and 2.6 mg/kg/h [31, 75, 76]. The use of an automated controller may decrease the duration of excessive sedation (BIS < 40) and this may improve hemodynamic stability and other adverse affects due to hypnotic overdosing. This study [75] has also reported a significant decrease of vasopressor use in the automated sedation group probably because of better hemodynamic stability or because the decrease of the workload gave the staff more time to optimize the volume status of the patients. The decrease in workload was clearly demonstrated in this study. The number of infusion rate change is notably and significantly different between closed-loop and manual control with 39 ± 29 changes per hour versus 2 ± 1, respectively, for propofol and 40 ± 9 changes for remifentanil versus 1 ± 1, respectively (p < 0.001). These results confirm that the continuous titration of sedation is a very difficult goal for physicians and nurses. In contrast, an automatic system reduces workload and can free time for direct patient care [77]. This study also demonstrated that the administration of anesthetic agents by ICU’s nurses with a limited knowledge of propofol and remifentanil might be associated with adverse effects, whereas continuous feedback of drug effects by the controller avoids drug side effects and finally improves patient safety .
Automated sedation for ICU patients is a new concept. Clinical studies have reported the feasibility and accuracy for the automated titration of propofol and remifentanil guided by EEG activity. At present, studies have demonstrated that at least intermediate variables are improved by automated sedation. The challenge is to confirm such benefit and assess its impact on patient outcome with randomized multicenter studies.
Automated Intravenous Anesthesia in Specific Circumstances
Application for Pediatric Patients
Studies of the use of automated control of anesthesia in the pediatric population are still limited, although two randomized controlled trials in this population have now been published [30, 78]. Automated and continuous titration of intravenous anesthesia is particularly suitable in pediatric patients. Indeed the majority of published pediatric PK models have a limited predictability [79] related to the wide inter-patient variability observed in children [80]. The first successful use of an automated controller in the pediatric population was performed on a 9-year-old child (ASA IV) requiring an emergency lung reduction in 2007 [81]. Automated titration of propofol and remifentanil was performed during induction and maintenance of general anesthesia. The duration of maintenance was 115 min and the BIS was in the range 40–60 during 77 % of the procedure despite the use of the adult PK model of Schnider for the propofol administration [22] and the adult model of Minto for the remifentanil administration [41].
The first controlled study in children involved 20 pediatric patients undergoing cardiac surgery with cardiopulmonary bypass [30]. This study compared a controller allowing the automated titration of propofol during induction and maintenance of general anesthesia with manual titration of propofol. The controller, developed initially for the adult population [27], was modified to take into account the pharmacokinetic differences of pediatric patients. In particular the distribution volume and the clearance of propofol in the model were increased as well as dosage and the maximum allowed propofol infusion rate. The mean age was 11 ± 5 years in the closed-loop group . Fentanyl was titrated manually. The automated controller decreased propofol consumption during induction and the off-cardiopulmonary bypass period and avoided overshoot of BIS during induction. Moreover in the closed-loop group the hemodynamic stability was improved with decreased phenylephrine use in the pre-cardiopulmonary bypass period.
An observational study reported automated titration of propofol guided by the NeuroSense® (NeuroWave System., Inc, Cleveland Heights, OH) in 108 pediatric patients with a median age of 12 (6–17) years undergoing gastrointestinal endoscopy [55]. A remifentanil bolus was administered followed by a continuous infusion, whereas propofol administration was automatically administered during induction and maintenance of sedation. The NeuroSense® index was in the adequate range (within 10 units of the targets) during 89 % of the time. In 85 % of the patients, no manual propofol adjustments were necessary and spontaneous breathing was maintained without manual intervention. However, as for adult patients, the control of analgesia is a strong requisite for adequate controller performance in anesthesia.
A dual controller was evaluated in pediatric and adolescent patients for the automated titration of propofol and remifentanil during induction and maintenance of general anesthesia [78]. In 23 patients with a median age of 12 [10–14] years the dual controller outperformed the ability of manual control to maintain the BIS in the range 40–60. The controller also decreased the period of deep anesthesia. Although remifentanil consumption was higher in the closed-loop group, no increase in pain scores or morphine requirements was observed in the postoperative period. In this study the controller was similar to the controller evaluated in the adult population [43], using the adult pharmacokinetic models of Schnider [22] and Minto [41], and only the upper limits of target were increased for both drugs. Finally these different studies demonstrated the feasibility and the accuracy of automated titration of propofol [30, 55, 78] or remifentanil [78] in children older than 6 years guided by EEG activity. By a continuous titration of drug administration according to the effect measured by the EEG activity, the controller compensates for the errors when using adult pharmacokinetic models in children .
Application for Cardiac Surgery
A PID controller previously validated during orthopedic, urologic, or general surgery [26] was tested in adult patients undergoing open heart surgery with cardiopulmonary bypass [27]. In this randomized controlled trial, the single automated controller BIS-propofol was tested in 19 adult ASA I-III patients during induction and maintenance of general anesthesia and fentanyl was administered manually in both groups. The overshoot of BIS and the decline in mean arterial pressure during induction were lower with the automated controller. This was related to the decrease of propofol dose to achieve induction. During maintenance the automated controller decreased propofol consumption. The automated controller outperformed manual titration to maintain the BIS in the range of adequate anesthesia despite the use of cardiopulmonary bypass which alters propofol PK. More interestingly, this study reported a better hemodynamic stability in the automated group associated with a decrease in phenylephrine requirements. The automated controller was described as a valuable tool which improved hemodynamic stability. The same controller was also evaluated in pediatric patients [30].
Application for Obese Patients
In morbidly obese patients, the titration of intravenous anesthesia is a challenge because of difference in PK/PD parameters compared with lean patients [82]. Whether to dose the drugs based on the total body weight or the ideal body weight with or without “fictitious height” is hotly debated [83]. Moreover, the use of the PK models validated in lean patients can generate propofol overdosing during maintenance of general anesthesia in obese patients [84]. An alternative strategy is to titrate directly to a measure of depth of anesthesia or by the use of BIS-guided automatic drug administration. An automated controller is based on individual patient responses, the amounts of propofol and remifentanil given being based on individual requirement. The titration does not rely on an accurate underlying PK model or weight adjustment methods .
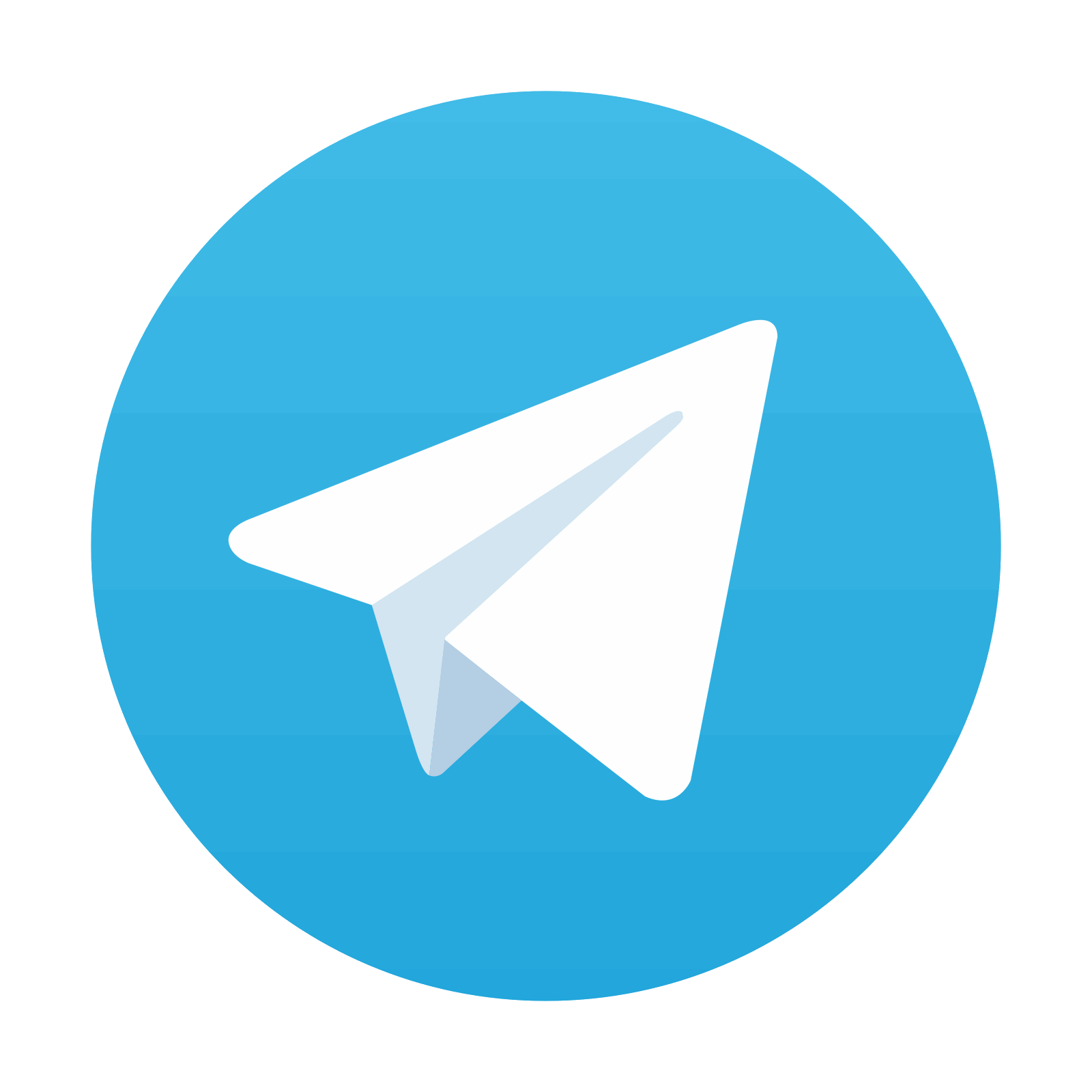
Stay updated, free articles. Join our Telegram channel

Full access? Get Clinical Tree
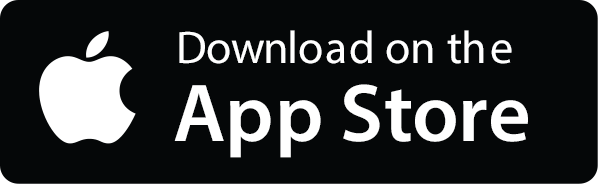
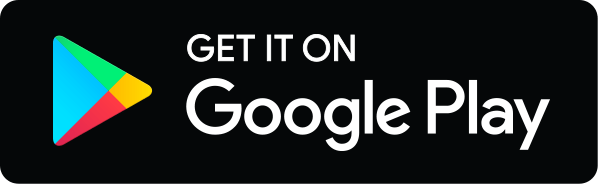