Routes of Administration
Intravenous. The intravenous route is the most certain route to deliver drugs to the bloodstream under all conditions and is the principal route for all anesthetic drugs given parenterally. Be very careful to check all drugs (i.e., read the label ) and doses before administration. In the case of less commonly used drugs (e.g., antibiotics), ensure that the manufacturer’s directions as to route of administration, speed of injection, and dilution are carefully followed. Rapid injection of some drugs (e.g., vancomycin) may cause severe physiologic effects (e.g., cardiac arrest, “red man syndrome”). Administration of drugs using tuberculin syringes or when diluted is very important in neonates and infants. It is essential to eject all air bubbles from the syringe before administration. Be very careful that all intravenous tubing is flushed after all drugs are administered to ensure that no drugs remain in the tubing or the dead space of the injection port. Serious incidents have occurred when neuromuscular blocking drugs or anesthetics/opioids have been flushed into the child after a procedure has ended.
N.B. Drugs should not be injected into infusions of hyperalimentation fluids because infection or thrombosis of the line may result. However, some children will have had a central line inserted for the specific purpose of providing for repeated anesthesia/sedation procedures. In this case, access the line using appropriate connectors and with careful aseptic technique. In other cases, it may be appropriate to “Hep-Lok” a peripheral IV for use in subsequent procedures.
Intramuscular. Drugs administered intramuscularly are rapidly absorbed, especially in small children and preferably should be given in the lateral aspect of the thigh. (Absorption is more rapid from the arm [deltoid] muscle than from the leg muscle; however, it carries a risk of nerve damage). Intramuscular injections are much less reliable in children in shock or who are hypovolemic, and there is a danger that repeated doses may have a cumulative effect when muscle tissue perfusion improves. Intramuscular injections are painful and generally avoided in conscious children.
Intralingual. Injections into the tongue have been recommended for use in an emergency (e.g., succinylcholine) when parenteral access is limited. Systemic absorption is rapid via this route. However, we have never found a need for this technique and do not recommend it (see page 69 ).
Intratracheal. Drugs sprayed into the trachea through a tracheal tube are quite rapidly absorbed, and this may be a useful in an emergency if an intravenous route is not available (e.g., to administer atropine or epinephrine during cardiopulmonary resuscitation). However, this route is no longer recommended as a first choice for resuscitation; if it is used, larger drug doses are required. It is important to either dilute the drug to a larger volume or flush the drug into the tracheobronchial tree with 3 to 5 ml of saline to ensure delivery to a mucosal surface. Beware that local anesthetics sprayed into the trachea are rapidly absorbed; always check to ensure that the total dose given is safe.
Rectal. Use of suppositories (acetaminophen) or rectal administration of drugs (e.g., pentobarbital, methohexital) is usually well accepted by children under 3 years of age. Absorption is less certain than with other routes, in part because of the partial first-pass effect of the liver when absorption occurs through the inferior/middle hemorrhoidal veins and the variable volume and pH of the rectal milieu. Unanesthetized children older than 3 years of age may be upset if medications are administered via this route.
Oral. Preoperative medication and postoperative analgesics may be given to selected children by this route. The oral route cannot be used if vomiting or other gastrointestinal dysfunction exists. Many drugs are very rapidly and predictably absorbed across the oral mucous membrane (e.g., fentanyl, midazolam) if administered as a lozenge or placed under the tongue. The oral transmucosal route is a potentially very useful route for drug therapy in children.
Intranasal. Some drugs are well absorbed across the nasal mucous membrane and are rapidly effective by this route (e.g., sufentanil, midazolam). However, many children are upset at having nose drops instilled, and this route has not gained wide acceptance. There is also a concern that some drugs or their preservative (e.g., midazolam) might be neurotoxic if they penetrate the cribriform plate into the brain.
Intraosseous. This route is recommended for use during resuscitation in all age groups when an intravenous route is not available. Drugs are rapidly absorbed by this route and may be given in the usual doses. This route is now advocated for pediatric resuscitation if initial attempts at venous access fail (see Chapter 4, page 128 ).
DISTRIBUTION OF ADMINISTERED DRUGS
In infants and young children, the relative sizes of the body fluid compartments differ from those in the adult. The extracellular fluid compartment is large; hence, drugs that are distributed throughout this space (e.g., succinylcholine) are required in larger doses.
Protein binding is less in neonates because of reduced total serum protein concentrations and reduced concentrations of specific proteins (e.g., α 1 -acid glycoprotein). More of the administered drug is free in the plasma to exert a clinical effect. For this reason, reduced doses of such drugs as barbiturates and local anesthetics are indicated.
The composition of the body also has an influence on drug distribution; neonates have little fat or muscle tissue. Drugs normally distributed throughout these tissues will have greater plasma concentrations and prolonged duration of action.
METABOLISM AND ELIMINATION OF DRUGS
The half-lives of drugs that are metabolized in the liver in the neonate and small infant are generally greater than in the adult (e.g., opioid analgesics). Hepatic blood flow and hepatocellular enzymatic activity are the primary determinants of the rate of metabolism of a drug by the liver. Hepatic blood flow may be reduced in the small infant because of increased intraabdominal pressure and congestive cardiac failure. In the first few postnatal days, blood may shunt past the liver via a patent ductus venosus. The conjugation pathways for drug metabolism are immature in preterm infants and are not fully active until several months of age. Hence, the elimination half-life of drugs such as morphine in this age group is increased. In addition, alternative pathways for drug metabolism may result in the accumulation of metabolites, some of which may be pharmacologically active (e.g., morphine-3- and 6-glucuronide).
Older infants and young children demonstrate a rapid elimination of some drugs, reflecting the greater hepatic blood flow and enhanced metabolic activity in the child’s liver.
Drugs excreted via the kidney (e.g., most antibiotics) depend on the glomerular filtration rate or tubular secretion capacity, both of which are reduced during the first few weeks of postnatal life, thus necessitating greater intervals between drug dosing.
Suggested Reading
Drugs Used in Anesthesia
Inhalation Agents
The concentration of inhaled anesthetics in the alveoli increase more rapidly with decreasing age: infants > children > adults. This is the result of the increased alveolar ventilation to functional residual capacity ratio, the greater proportion of vessel-rich tissues that rapidly equilibrate with blood concentrations, and the reduced blood-gas and tissue-gas partition coefficients (λ) of the inhaled anesthetics (except sevoflurane) in infants. Therefore, induction of anesthesia is more rapid in infants and small children. The rapid increase in alveolar, blood, and tissue concentrations of inhaled anesthetics may account in part for the precipitous decreases in blood pressure that occur when greater concentrations of these anesthetics are given to infants, particularly during controlled ventilation.
Excretion of inhaled anesthetic agents, and therefore recovery, is also more rapid in infants and small children than in adults, provided that ventilation is not depressed. The alveolar concentration of N 2 O decreases to 10% within 2 minutes after discontinuation of 70% N 2 O, a level not reached until 10 minutes in adults.
The minimum alveolar concentration (MAC) of inhaled anesthetics is greater in infants than in older children and adults ( Table 3-1 ); the reasons for this are unknown. MAC values have been determined for a number of anesthesia interventions including tracheal intubation and extubation, and for LMA insertion and removal ( Table 3-2 ).
Age | Halothane | Isoflurane | Sevoflurane | Desflurane |
---|---|---|---|---|
Preterm neonate | 0.55 | 1.3–1.4 | Not available | Not available |
Full-term neonate | 0.87 | 1.6 | 3.3 | 9.1 |
Infant | 1.2 | 1.8 | 3.2 | 9.4 |
Child | 0.95 | 1.6 | 2.5 | 8.5 |
MAC (%) | |
---|---|
Tracheal intubation |
|
Tracheal extubation |
|
LMA insertion |
|
LMA extubation | Sevoflurane: 1.84 |
Tracheal intubation/ skin incision ratio * | Halothane, enflurane, sevoflurane: 1.33 |
Awake | Sevoflurane: 0.3 |
All inhaled anesthetics depress ventilation to a similar degree as evidenced by CO 2 response curves. A dose related decrease in V t and minute ventilation is accompanied by an increase in Pa CO 2 . Intercostal muscle activity is inhibited, particularly during halothane anesthesia. This contributes to the depressed ventilation, and may lead to paradoxical chest wall movement, especially if any degree of airway obstruction occurs.
Inhaled anesthetics are myocardial depressants due to their calcium channel blocking activity; this is most marked with halothane, less with isoflurane, and least with sevoflurane or desflurane. Halothane frequently, and sevoflurane rarely, causes bradycardia, which may be corrected by atropine administration. Inhaled anesthetics may prolong the Q-Tc interval (halothane > sevoflurane > isoflurane) in some children, especially young infants. Although this may be insignificant, in many children it may predispose those with long Q-T syndrome to serious arrhythmias. Recent evidence points to the dispersion of repolarization (defined as the difference between the maximum and minimum Q-T interval) as predisposing to ventricular arrhythmias.
Inhaled anesthetics inhibit hypoxic pulmonary vasoconstriction and thereby disrupt the mechanism that normally redistributes perfusion away from underventilated alveoli. This increased shunt may cause a clinically significant decrease in arterial oxygen saturation, especially in infants with lung disease (e.g., bronchopulmonary dysplasia).
All of the inhaled anesthetics reduce the requirements for nondepolarizing neuromuscular blocking drugs (NMBDs) to produce a standard degree of block. This relaxant enhancing effect may be useful in reducing the total dose of NMBDs administered and facilitating antagonism of the neuromuscular blockade.
All inhaled anesthetics are capable of triggering MH and should be avoided in a child with an MH history. Controversy exists regarding the risk of rhabdomyolysis in children anesthetized with inhalational anesthetics.
Nitrous Oxide (N 2 O)
N 2 O is commonly used in pediatric anesthesia to speed and facilitate induction and to provide analgesia/amnesia during maintenance. It may also be administered to sedate and to provide analgesia before intravenous induction of anesthesia. N 2 O is odorless and insoluble (blood/gas partition coefficient (λ b/g ) is 0.47) with a MAC of 104% in adults. In large concentrations, it enhances the rate of uptake of the inhaled anesthetics into the alveoli, accelerating induction of anesthesia (second-gas effect). The analgesic effects of N 2 O may complement the anesthetic regimen during maintenance. The effects of N 2 O on ventilation appear to equal those of equipotent concentrations of halothane. N 2 O mildly depresses cardiac output and systemic blood pressure in infants, but it has little effect on pulmonary artery pressure or pulmonary vascular resistance, even in those with pulmonary vascular disease. In infants and small children, the cardiovascular effects of N 2 O combined with either halothane or isoflurane to 1.5 MAC are similar to those of equipotent (1.5 MAC) concentrations of either halothane or isoflurane in oxygen. N 2 O rapidly diffuses into any gas-containing space within the body; this contraindicates its use in those with lung cysts, pneumothorax, lobar emphysema, necrotizing enterocolitis, bowel obstruction, and any other gas filled cavity. N 2 O (70%) doubles the size of a pneumothorax in 12 minutes and that of gas containing bowel in 120 minutes. The tenfold difference in doubling time is the result of the shrinking blood supply to the bowel, as the lumen of the bowel expands, and unchanged blood flow to the chest wall/pleura with expansion of the pneumothorax. N 2 O also diffuses into the middle ear and may displace the graft during tympanoplasty. In some children with a normal ear and an intact eardrum, postoperative absorption of N 2 O from the middle ear results in atelectasis of the drum and a later complaint of earache. N 2 O does not appear to increase postoperative vomiting in children. However, when treating children for procedures that are associated with a high incidence of PONV, it may be prudent to avoid nitrous oxide.
Sevoflurane
In the past decade, sevoflurane has become the agent of choice for inhalational induction in children. A fluorinated methyl isopropyl ether, it has a low solubility in blood (λ b/g is 0.68), a not-unpleasant odor, and is the least irritating to the airway of the inhaled anesthetics. Thus it is the ideal agent for inhalational induction. Induction of anesthesia can most rapidly be achieved by administering the maximum deliverable initial concentration (8%); it is not necessary to introduce sevoflurane in slow stepwise increases in inspired concentration except as a means of reducing its pungency for the child. Slowly increasing the inspired concentration of sevoflurane only prolongs the excitement period. To minimize the response to 8% sevoflurane during induction, the mask should be flavored and 70% N 2 O in oxygen administered first until the child stops responding. For older, cooperative children, the so called “single breath induction” (i.e., a vital capacity breath) using an anesthesia circuit primed with 8% sevoflurane in 66% nitrous oxide and fitted with a large reservoir bag will induce anesthesia rapidly and smoothly. The effect of age on the MAC of sevoflurane differs from that of the other anesthetics: it is 2.4% in children 6 months to 10 years and 3.2% in neonates up to 6 months of age. The large MAC limits the magnitude of the overpressure technique that is possible with an 8% vaporizer. The addition of 60% N 2 O to sevoflurane in children only decreases the MAC by 23%. Attempts at intravenous cannulation should not be made until the child is adequately anesthetized and unresponsive. Satisfactory conditions for endotracheal intubation without neuromuscular blocking agents can be achieved quite rapidly as anesthesia is deepened especially if a single dose of propofol is administered before intubation or ventilation is controlled for a brief period.
During sevoflurane anesthesia there is a dose related decrease in tidal volume, respiratory frequency, and minute ventilation, which is reversed by surgical stimulation. In practice, the addition of nitrous oxide to sevoflurane decreases the MAC of the latter and thus minimizes respiratory depression in the spontaneously ventilating child.
Sevoflurane causes less myocardial depression than halothane, isoflurane, or desflurane and arrhythmias are uncommon, even when epinephrine-containing solutions are injected. An increase in heart rate and a slight decrease in blood pressure usually occur after induction of anesthesia. Occasionally bradycardia occurs during induction of anesthesia in infants and particularly in children with trisomy 21, but this may be prevented by pretreatment with an anticholinergic. Compared with halothane, sevoflurane causes less hypotension in children with congenital heart disease and less desaturation in those with cyanotic CHD.
Epileptiform EEG activity and myoclonic jerking movements have been reported exceedingly rarely during sevoflurane anesthesia in children. The combination of high concentrations of sevoflurane and hyperventilation appear to increase the epileptiform activity, although frank seizures are exceedingly rare. Epileptiform EEG activity during sevoflurane may cause paradoxical readings on “depth of anesthesia” monitors (e.g., the BIS monitor). Caution should be exercised when interpreting BIS values during sevoflurane anesthesia at higher concentrations.
Emergence from sevoflurane anesthesia is smooth and rapid, although emergence agitation may occur in some children, especially preschool-age children (see page 216 ). This transient phenomenon is characterized by restless and inconsolable behavior, inability to establish eye contact, lack of purposeful movement, and lack of interaction with their surroundings. To reduce the incidence of agitation, care must be taken to ensure adequate analgesia during emergence by means of a regional block or systemic analgesics. Antinociceptive strategies combined with midazolam premedication, propofol, or an alpha 2 agonist (e.g., dexmedetomidine) all reduce the risk of emergence agitation. There is some evidence that recovery of fine coordination over the first few hours after sevoflurane is more complete than it is after isoflurane or halothane.
Sevoflurane is metabolized (5%) in vivo by CYP450 2E1 releasing inorganic fluoride; maximum plasma concentrations occur within 2 hours of terminating the anesthetic. Although large plasma inorganic fluoride concentrations after methoxyflurane were associated with nephrotoxicity, this does not occur after sevoflurane. Nephrotoxicity depends on the affinity of renal CYP450 2E1 for the ether anesthetic, which locally releases inorganic fluoride that may impair renal tubular function. In the case of CYP450 2E1, the affinity for sevoflurane is one fifth that of methoxyflurane, hence nephrotoxicity does not occur with the former despite similar plasma concentrations of inorganic fluoride to those after methoxyflurane. Sevoflurane is also degraded in vitro in the presence of some carbon dioxide absorbents (baralyme > soda lime>>Amsorb Plus). The extent of degradation in vitro increases at low fresh gas flows and in desiccated absorbent. Degradation of sevoflurane in vitro yields five compounds, the most common being Compound A, a potentially nephrotoxic vinyl compound. Compound A production varies directly with the weight of the child. Absorbents that are free of KOH and NaOH such as Amsorb Plus do not degrade sevoflurane. In some countries, a minimum fresh gas flow and concentration-time exposure have been recommended for sevoflurane although there is limited evidence that very low fresh gas flows present any risk to humans. The combination of desiccated baralyme and sevoflurane resulted in an absorber fire (the result of hydrogen production from degradation of sevoflurane in the presence of desiccated baralyme at temperatures >200 °C) that led to the withdrawal of baralyme from the market. To date, neither sevoflurane nor its degradation products have been shown to be toxic in humans.
Isoflurane
Isoflurane is a polyhalogenated methyl ethyl ether. It is a stable compound that is metabolized less than 0.2% in vivo, although it is degraded to carbon monoxide in the presence of some desiccated CO 2 absorbents. It is eliminated almost completely unchanged via the lungs; therefore recovery should be very complete.
The λ b/g of 1.43 dictates that wash-in to the alveoli is slower than that of sevoflurane. In addition, isoflurane is not suited as an induction agent because its pungent odor irritates airway reflexes (coughing, laryngospasm, and breath holding). Nonetheless, isoflurane can be successfully introduced after an intravenous induction, provided the concentration is increased slowly. Alternatively, for more prolonged surgery, anesthesia may be maintained with isoflurane after induction has been successfully completed with sevoflurane. Recovery after isoflurane anesthesia is slower than after sevoflurane although emergence agitation appears to be less of a problem. The incidence of laryngospasm during extubation and emergence is similar to that with halothane (see later discussion). Isoflurane depresses the respiratory system to a similar extent as sevoflurane.
During isoflurane anesthesia, blood pressure decreases although heart rate does not change substantively. The decrease in blood pressure is due in part to myocardial depression, but also to peripheral vasodilation. Infusion of intravenous fluids tends to restore the blood pressure. The vasodilating effect of isoflurane may be useful to control blood pressure, such as during induced hypotension. Isoflurane depresses the baroreflex in the neonate. This impairs the ability to compensate for changes in arterial blood pressure and for hypovolemia. Isoflurane does not sensitize the myocardium to the effects of catecholamines or theophylline.
Isoflurane potentiates nondepolarizing neuromuscular blocking drugs to a greater extent than sevoflurane or halothane, thus allowing reduced doses of relaxant drugs to be used. The neuromuscular effects of isoflurane are reversible when isoflurane is withdrawn, thereby facilitating reversal of neuromuscular blockade.
Halothane
Halothane was considered to be an ideal anesthetic for children, although its popularity in pediatric anesthesia has waned since the introduction of sevoflurane into clinical practice. Indeed, many recent North American trainees have never used halothane in children, unless they have administered anesthesia overseas. Nonetheless, halothane is inexpensive and is still used in some parts of the world where more expensive anesthetics are less available. Pediatric anesthesiologists familiar with halothane consider that it may have some advantages over sevoflurane when a more prolonged emergence is needed to facilitate endoscopy with spontaneous ventilation (e.g., for laryngoscopy, bronchoscopy), or when managing a difficult airway. Its use will therefore be discussed.
With a λ b/g of 2.3 the wash-in of halothane is slow compared with sevoflurane. However, with a 5% maximum inspired concentration and a MAC in children of approximately 1%, greater MAC-multiples of halothane can be delivered than of sevoflurane. The MAC in children who are cognitively challenged may be 25% less than in children who are not challenged. Halothane provides a smooth inhalation induction with minimal irritation of the airways.
During halothane anesthesia, a dose-dependent depression of spontaneous ventilation occurs; there is usually an increase in respiratory rate, but tidal volume and minute ventilation decrease considerably. This increases the end-tidal carbon dioxide concentration but prevents anesthetic overdose as long as respirations are not assisted or controlled (see later discussion). Alveolar ventilation returns toward normal during surgical stimulation but is variable throughout anesthesia. Halothane inhibits intercostal muscle activity. Diaphragmatic ventilation predominates, and paradoxical movement of the chest wall may occur. Even very low blood levels of halothane severely depress the ventilatory response to hypoxia in young adult volunteers. It is likely that this effect also occurs in children of all ages. Laryngospasm may occur during light planes of halothane anesthesia, especially during extubation of the trachea. This effect can be avoided by extubating the trachea while the child is either still deeply anesthetized or completely awake. Lidocaine (1 to 2 mg/kg IV) given slowly before extubation may reduce coughing but will not prevent laryngospasm. Halothane is a potent bronchodilator and is very useful in children with asthma.
Halothane depresses myocardial contractility, especially in small infants. It also produces bradycardia and thus causes a decrease in cardiac output. Atropine prevents the bradycardia and tends to maintain cardiac output, but does not reverse the reduced myocardial contractility. Neonates are especially sensitive to the myocardial depressant effects of halothane. Severe hypotension may ensue if large concentrations of halothane are administered to infants and children, particularly when ventilation is controlled. This is a result of myocardial depression and, with the subsequent decreased cardiac output, the concentration of halothane rapidly increases in the child’s myocardium, leading to electromechanical dissociation/cardiac arrest.
The infant’s blood pressure is very sensitive to changes in cardiac output. Vasoconstriction is less effective than in the adult. Halothane also depresses reflex baroresponses. Inspired halothane concentrations are usually limited to 0.5% to 1% during controlled ventilation, and the blood pressure should be carefully monitored. In children with cardiac failure, the myocardial depressant effects of halothane are prominent, and severe hypotension may occur.
Arrhythmias also occur during halothane anesthesia. Ventricular premature beats are common, especially during spontaneous ventilation in the presence of hypercarbia. If they occur, ventilation should be assisted or controlled; if they persist, another inhalational anesthetic (e.g., isoflurane) should be substituted for halothane. Junctional rhythm and wandering pacemaker also occur during halothane anesthesia. This is usually of little consequence but might compromise the child with congenital heart disease. Halothane sensitizes the myocardium to exogenous catecholamines, and arrhythmias may occur when these compounds are injected (e.g., to infiltrate the skin). Studies indicate that children tolerate greater doses of injected epinephrine than adults. Epinephrine in doses up to a maximum of 10 μg/kg mixed with local anesthetic or saline and injected into the tissues of healthy children appears to be safe. Serious arrhythmias may occur if halothane is administered to children who have been receiving theophylline medication chronically; other agents should be used.
Halothane, like most other inhalational anesthetics, increases cerebral blood flow and therefore may increase ICP. At small concentrations, this effect is minimal, and if hyperventilation is employed, the increase is insignificant even in those with intracranial space-occupying lesions.
Shivering and muscle rigidity are common during emergence from halothane anesthesia. These effects may be of concern after orthopedic surgery and in children in whom the additional oxygen demands of shivering might be detrimental. In such cases, an alternative anesthetic technique may be more appropriate.
Halothane is metabolized 15% to 20% in adults in vivo, but the extent of metabolism is less in infants and small children. Occasional cases of hepatic failure have been reported in adults, but many fewer have been reported in children, despite its wide and often repeated use in the latter age group. These rare cases of halothane hepatitis in children have been confirmed by the presence of halothane-related antibodies. Most episodes of halothane hepatitis in children run a less fulminant course than in adults. The reason for the reduced susceptibility of prepubertal children to halothane hepatitis is not known. Contraindications to halothane include a history of unexplained postoperative jaundice.
Desflurane
Desflurane is a fluorinated ether with a boiling point of 23 °C and the smallest λ b/g , 0.42. In addition, it is the least soluble anesthetic in all tissues. It is a very stable compound, with less than 0.02% metabolized in vivo, although it is degraded to carbon monoxide in the presence of some desiccated absorbents. Desflurane is a less potent anesthetic agent with a MAC of 7% to 9.5% in children. MAC increases with decreasing age, peaking in infants 6 to 12 months of age. The effects of desflurane on the cardiovascular system at 1 MAC anesthesia are similar to those of other ether inhalational anesthetics, although bradycardia is rare. In adults, when desflurane is the sole anesthetic agent, sudden increases in the inspired concentration can lead to profound central sympathetic discharge, resulting in sudden increases in blood pressure and heart rate.
Desflurane is very pungent and causes airway irritation; breath holding and laryngospasm are very common and for these reasons desflurane is not recommended for inhalational inductions in children. It can, however, be safely used for maintenance after induction with other agents in children whose airways are intubated. There are limited data on airway responses to desflurane in children managed with either a mask or an LMA. Emergence is very rapid as a result of its limited solubility. To prevent the sudden onset of acute pain, analgesics should be administered before emergence. Emergence agitation has also been reported after desflurane, particularly if pain is present. The very rapid recovery may be useful in some children (e.g., small infants at risk for postoperative apnea, although this does not eliminate this risk).
Desflurane increases intracranial pressure more than isoflurane and sevoflurane, although this effect may be attenuated by hyperventilation before introducing the desflurane.
Desflurane is less convenient to use than other agents because its low boiling point demands a specially designed, electrically heated vaporizer. A greater concentration is required to maintain anesthesia (because of its greater MAC), necessitating small fresh gas flows to limit the cost.
Enflurane
This inhaled agent has several disadvantages compared with other agents and, as a result, is not commonly administered to children.
Summary
- 1.
The MAC values of inhalational anesthetics increase with decreasing age from adults to infancy at which age these peak and decrease as age further decreases in neonates and preterm infants.
- 2.
The smaller the child, the more rapid the uptake of inhalational anesthetics.
- 3.
Large concentrations of inhalational anesthetics can cause serious hypotension in infants and young children, particularly when ventilation is controlled. Beware: overdose of inhaled agents is a leading cause of serious complications.
- 4.
Sevoflurane is currently the most commonly used induction agent in infants and children.
- 5.
All inhalational anesthetics may be used for maintenance, although recovery is more rapid with those of reduced solubility: desflurane < isoflurane ∼ sevoflurane < halothane.
- 6.
Halothane remains a useful alternative to sevoflurane and is less expensive. It may be useful during endoscopy and in the management of the difficult airway.
- 7.
Halothane-induced hepatic failure is rare in children younger than 14 years of age.
Suggested Reading
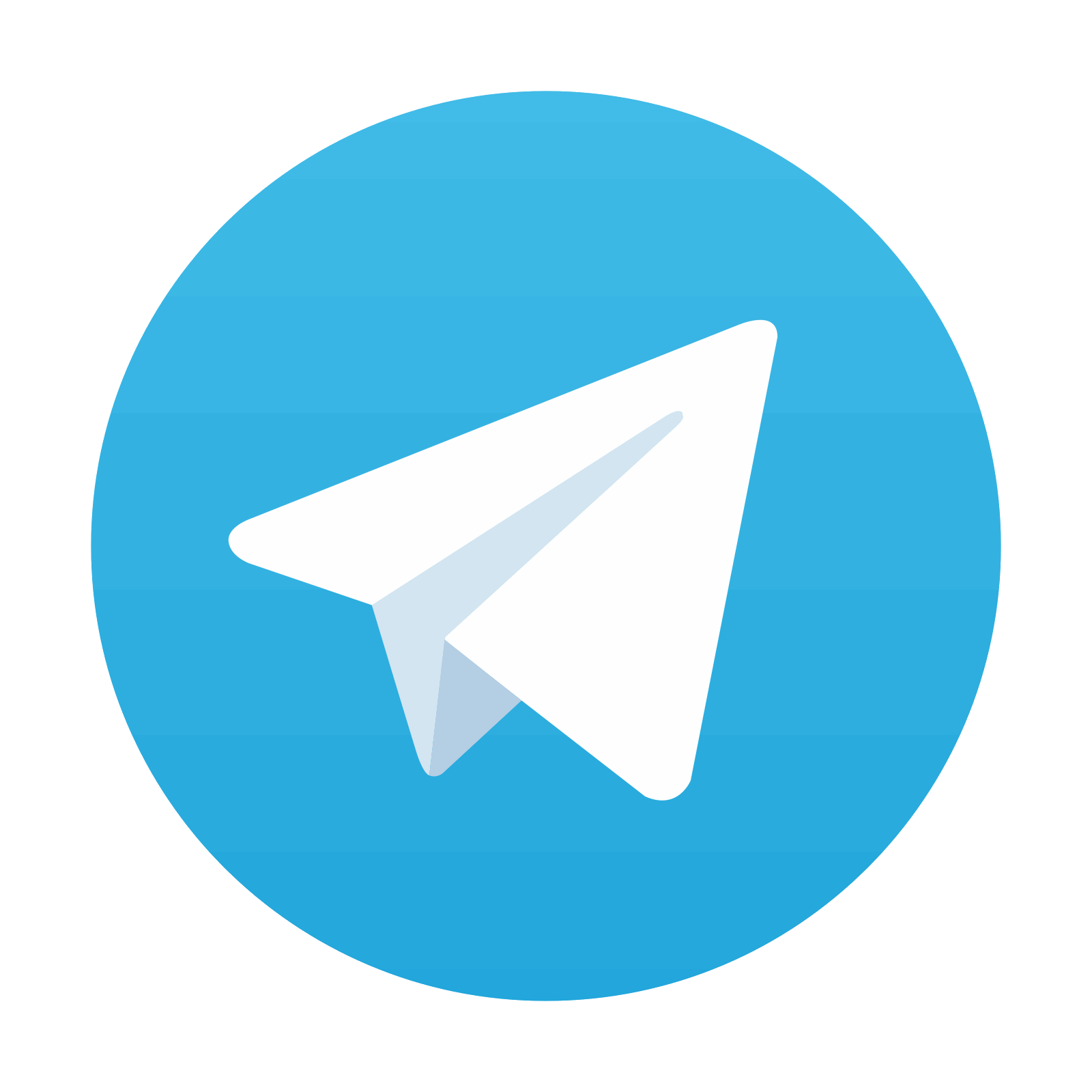
Stay updated, free articles. Join our Telegram channel

Full access? Get Clinical Tree
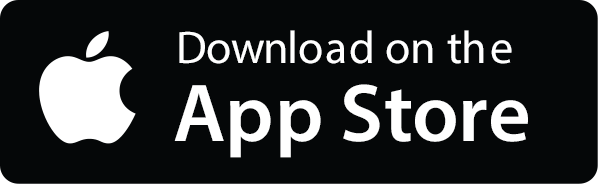
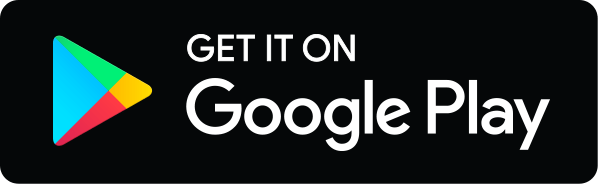