Fig. 8.1
The synaptic vesicle exocytosis–endocytosis cycle. After an action potential and Ca 2+ influx, phosphorylation of synapsin is activated by calcium-calmodulin activated protein kinases I and II. This results in the mobilization of synaptic vesicles (SVs) from the cytomatrix toward the plasma membrane. The formation of the SNARE complex is an essential step for the docking process. After fusion of SVs with the presynaptic plasma membrane, acetylcholine (ACh) is released into the synaptic cleft. Some of the released acetylcholine molecules bind to the nicotinic acetylcholine receptors (nAChRs) on the postsynaptic membrane, while the rest is rapidly hydrolyzed by the acetylcholinesterase (AChE) present in the synaptic cleft to choline and acetate. Choline is recycled into the terminal by a high-affinity uptake system, making it available for the resynthesis of acetylcholine. Exocytosis is followed by endocytosis in a process dependent on the formation of a clathrin coat and of action of dynamin. After recovering of SV membrane, the coated vesicle uncoats and another cycle starts again. See text for details. Acetyl CoA acetylcoenzyme A, CAT choline acetyltransferase, PK protein kinase (Reproduced with permission from Naguib et al. [2])
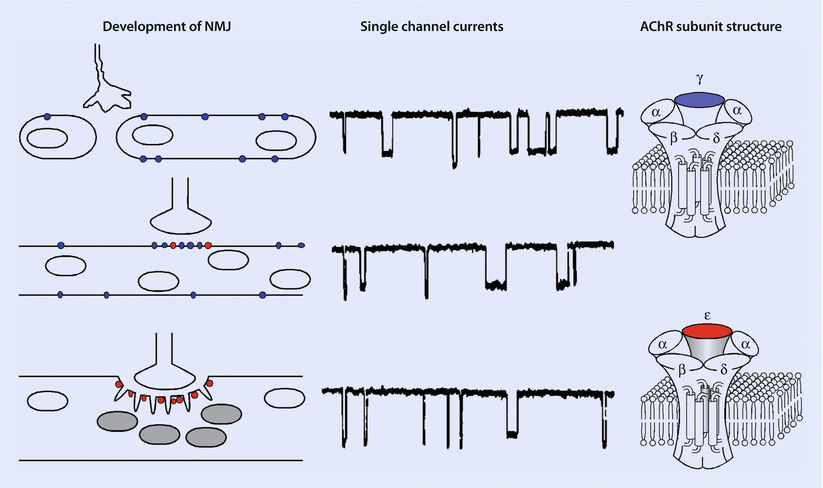
Fig. 8.2
Development of the neuromuscular junction. (Left) Motor neuron growth cones contact myotubes as they fuse from myoblasts and express mostly fetal nicotinic acetylcholine receptors (nAChRs; marked in blue) in their surface membranes. In adult muscle, adult nAChRs (marked in red) predominate and are largely concentrated at the neuromuscular junction. (Center) Records of AChR channel openings from muscle membranes at different stages of neuromuscular development. Fetal (top) and adult nAChRs (bottom) are activated by acetylcholine to form ion channels of different conductance and gating properties. (Right) Subunit composition of fetal and adult AChR subtypes. Fetal and adult AChR subtypes are characterized by the presence of a γ(gamma) and ε(epsilon) subunit, respectively (Reproduced with permission from Naguib et al. [2])
Once both binding sites on the α(alpha) subunits are bound by 2 ACh molecules or other agonist, a conformational change occurs that opens the ion channel, allowing for the entrance of sodium and calcium and the exit of potassium. The subsequent end-plate potential, when combined with enough other ACh-receptor interactions, depolarizes post-junctional membrane. This depolarization activates voltage-gated sodium channels, which mediate the initiation and propagation of action potentials across the surface of the muscle membrane and into the transverse tubules (T tubules) resulting in the upstroke of the action potential. There are 2 types of calcium channels: the dihydropyridine receptor (DHPR) in the transverse (T) tubules and the ryanodine receptor (RyR1) in the sarcoplasmic reticulum. DHPR-RyR1 interaction releases large amounts of calcium from the sarcoplasmic reticulum, causing muscle contraction. This process is known as excitation-contraction coupling. Repolarization of the muscle membrane is initiated by the closing of the sodium channels and by the opening of potassium ion channel that conducts an outward potassium current. Binding of ACh to only 1 α(alpha) subunit will not result in ion channel opening, current flow through these channels, or membrane depolarization. This scenario may occur in the presence of a nondepolarizing NMBD. Acetylcholine, succinylcholine, and nondepolarizing NMBDs have affinity for the binding sites on the nAChRs.
Fetal nicotinic ACh receptors have a similar pentameric complex (◘ Fig. 8.2), although it contains a δ(delta) rather than an ε(epsilon) subunit, which accounts for its decreased cation conductance and subsequent longer opening time. Clinically, this translates to ACh causing brief activation of these receptors and a reduction in the probability of the channel opening and causing a muscle contraction. This trait makes such fetal receptors resistant to nondepolarizing NMBD and more sensitive to succinylcholine. Furthermore, these receptors increase in denervated states via upregulation, when they extend beyond the NMJ (extrajunctional receptors) throughout the muscle membrane. Such upregulation leaves patients vulnerable to exaggerated potassium plasma increases (from intracellular stores) when succinylcholine is administered.
8.3 Depolarizing Neuromuscular Blocking Agents
8.3.1 Succinylcholine
Succinylcholine is the only depolarizing NMBD in clinical use. This compound, also called suxamethonium, is composed of 2 ACh molecules adjoined through an acetate methyl group. This structure accounts for this medication’s clinical effects. Succinylcholine produces the fastest, shortest, and most reliable neuromuscular blockade. This is accomplished by activating the postsynaptic ACh nicotinic receptor. Once bound to the both binding sites on the α(alpha) subunits of the nicotinic ACh receptors, hyperpolarization occurs and flaccid paralysis then ensues as the membrane desensitizes. The clinical presentation of fasciculations noted after succinylcholine administration is variable (in location and severity) among patients. It appears that antidromic conduction of action potentials can lead to the activation of unparalyzed parts of the motor unit, resulting in fasciculations.
After an intravenous (IV) bolus of 1–1.5 mg/kg (3–5 × ED95; ED95 is the dose that results in 95% depression of twitch height), peripheral muscles become flaccid in 1–2 min, and remain so for 10–12 min. Such a large dose is required as nearly 90% of this compound is hydrolyzed by butyrylcholinesterase (also known as plasma cholinesterase or pseudocholinesterase) in the plasma prior to reaching the NMJ. Despite peripheral muscle paralysis, patients may be able to resume spontaneous breathing as soon as 5 min after administration, as central muscle groups (such as the diaphragm) recover before peripheral groups. As very low concentrations of butyrylcholinesterase exist at the synaptic cleft, the termination of the effect of succinylcholine is determined primarily by its ability to diffuse into plasma (based on its concentration gradient) and the activity of butyrylcholinesterase for its hydrolysis (◘ Table 8.1).
Table 8.1
Pharmacokinetic and pharmacodynamic properties of the depolarizing neuromuscular blocking agent succinylcholine
Succinylcholine | |
---|---|
Class | Depolarizing |
Duration | Ultra-short acting |
Potency – ED95 (mg/kg) | 0.25–0.30 |
Intubating dose (mg/kg) | 1.0–1.5 |
Onset time (min) | 1.0–1.5 |
Clinical duration (min) | 7–12 |
Recovery index (RI25–75) (min) | 2–4 |
Volume of distribution (L/kg) | 0.04 |
Clearance (mL/kg/min) | 35 |
Elimination half-life (min) Normal organ function Renal impairment Hepatic impairment | <1 <1 <1 |
Maintenance dose (mg/kg) | N/A |
Infusion dose (mcg/kg/min) | Titrate to ST muscle response |
Elimination route/ metabolism | Butyrylcholinesterase |
Active metabolites | No active metabolites |
Side effects | Myalgia; bradycardia/ asystole in children or with repeated dosing; dual (phase II) block; anaphylaxis |
Contraindications (other than specific allergy) | High K+; MH; muscular dystrophy; children; receptor up-regulation (e.g., patients with neurologic deficiencies, prolonged immobility; etc.); butyrylcholinesterase deficiency (see text for more details) |
Comments | Fastest onset, most reliable NMBD for rapid tracheal intubation |
While succinylcholine has many characteristics that make it ideal for facilitating endotracheal intubation, clinicians must be familiar with its ample side effect profile. Most patients experience muscle fasciculation after administration, a clinical sign that foreshadows the ensuing flaccid paralysis, which occurs approximately 1 min after offset of fasciculations. Myalgias are fairly common after succinylcholine exposure, with about half of patients reporting this side effect. While an intuitive causal relationship between uncomfortable-appearing fasciculations and myalgia seems plausible, large-scale reviews have never established such a link. Nonetheless, small “defasciculating” doses of non-depolarizing NMBDs or “self-taming” doses of succinylcholine have been used in an effort to decrease the fasciculations and postoperative myalgia. Such techniques may attenuate myalgia, but have not been found to reliably prevent these side effects; alternatively, such pretreatment may expose patients to risks associated with partial paralysis, such as pulmonary aspiration. Non-steroidal anti-inflammatory drugs (NSAIDs) remain the only evidence-based treatment for succinylcholine-induced postoperative myalgias.
Again, owing to its similar structure to ACh, succinylcholine can produce bradycardia and even asystole, particularly in children or after repeated doses. In pediatrics, co-administration with atropine is a common practice to maintain the heart rate and subsequently, cardiac output, in the pediatric patients with fixed stroke volumes.
Succinylcholine causes a reliable increase in the plasma potassium level (0.5 mEq/L) in otherwise healthy patients. This side effect has little clinical importance unless patients have pre-existing hyperkalemia. Renal failure patients are no more susceptible to an exaggerated response to succinylcholine than are those with normal renal function. Alternatively, an exaggerated potassium increase that may lead to cardiac arrhythmia and even arrest can occur in patients with conditions associated with upregulation of extra junctional nicotinic nAChRs. As previously mentioned, such upregulation occurs in chronic denervated states, seen in patients with neuromuscular disease, massive trauma, sepsis, prolonged immobility or burns.
Succinylcholine is also associated with increases in intragastric pressure; however, proportional increases in the lower esophageal sphincter tone negate the potential risk of regurgitation. Transient increases in intraocular pressure also occur (up to 15 mm Hg) by unclear mechanisms. While this small increase in pressure must be considered and avoided in patients with preexisting high intraocular pressure, inadequate anesthesia, coughing, and ventilator dyssynchrony produce much greater increases in intraocular pressure. Co-administration with lidocaine or sufentanil can be used to attenuate succinylcholine-induced increases in intracranial pressure. Small increases in intracranial pressure also occur after succinylcholine administration; however, inadequate anesthesia during laryngoscopy with a subsequent hypertensive response is much more likely to elevate intracranial pressure.
The United States Food and Drug Administration (FDA) has issued a “black box” warning for succinylcholine in pediatrics, as these patients may have an undiagnosed myotonia or muscle dystrophy. Succinylcholine may trigger rhabdomyolysis and a fatal hyperkalemic state in these patients. Furthermore, succinylcholine is contraindicated in patients with malignant hyperthermia, a risk that is significantly increased when volatile anesthetics are used. Masseter muscle spasm is another complication that is specific to succinylcholine administration and may be associated with malignant hyperthermia.
The use of succinylcholine is also contraindicated in patients with butyrylcholinesterase deficiency (◘ Table 8.2). The heterozygous deficient state is present in approximately 1 in 480 patients, while the homozygous version may be present in 1 in 3,200 individuals. These patients require a much longer time to recover from succinylcholine administration and may require unanticipated postoperative mechanical ventilation and sedation/amnesia as they recover.
Table 8.2
Relationship between dibucaine number and duration of succinylcholine or mivacurium neuromuscular block
Type of butyrylcholinesterase | Genotype | Incidence | Dibucaine numbera | Response to succinylcholine or mivacurium |
---|---|---|---|---|
Homozygous typical | E1 uE1 u | Normal | 70–80 | Normal |
Heterozygous atypical | E1 uE1 a | 1/480 | 50–60 | Lengthened by 50–100% |
Homozygous atypical | E1 aE1 a | 1/3200 | 20–30 | Prolonged to 4–8 h |
8.4 Nondepolarizing Neuromuscular Blocking Agents
Nondepolarizing NMBDs act as competitive antagonists by binding to the α(alpha) subunits of the nicotinic acetylcholine receptor. NMBDs have been classically classified either based on their duration of action (long-, intermediate, and short-acting agents) or based on their structure (steroids or benzylisoquinolinium) (◘ Table 8.3 and ◘ Table 8.4). Nondepolarizing NMBDs are positively charged, relatively large molecules. In general, a dose of 2–3 × ED95 is used to facilitate tracheal intubation while a dose of 10% of the ED95 is used to maintain neuromuscular blockade (◘ Fig. 8.3).
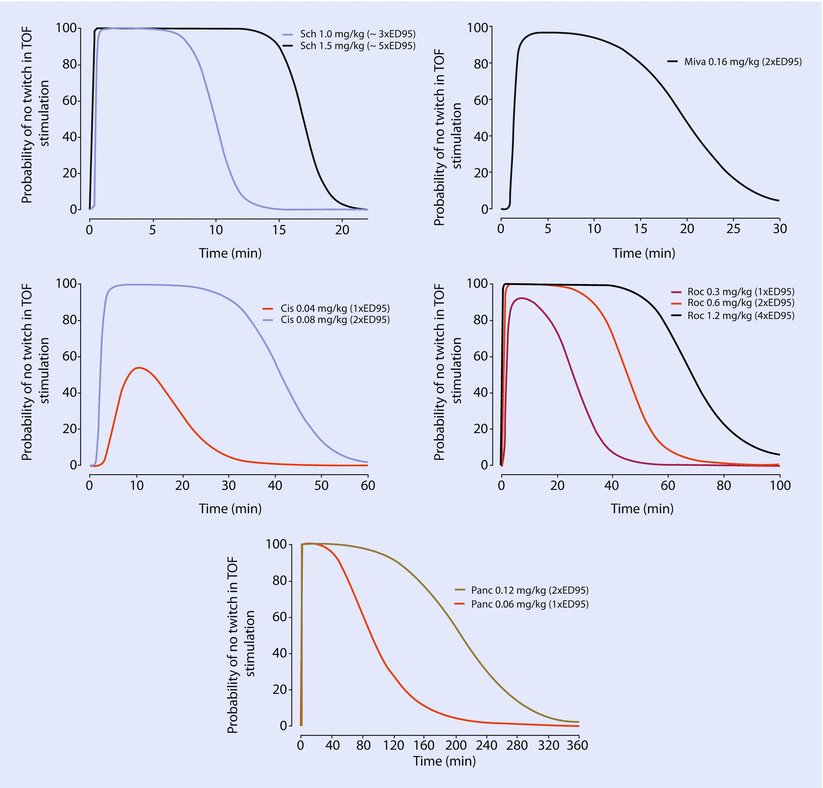
Table 8.3
Pharmacokinetic and pharmacodynamic properties of benzylisoquinolinium nondepolarizing neuromuscular blocking agents
Mivacurium | Atracurium | Cisatracurium | |
---|---|---|---|
Class | Non-depolarizing | Non-depolarizing | Non-depolarizing |
Duration | Short | Intermediate | Intermediate |
Potency – ED95 (mg/kg) | 0.08 | 0.25 | 0.05 |
Intubation dose (mg/kg) | 0.2 | 0.5 | 0.15–0.20 |
Onset time (min) | 3–4 | 3–5 | 4–7 |
Clinical duration (min) | 15–20 | 30–45 | 35–50 |
*Recovery index (RI25–75) (min) | 7–9 | 10–15 | 12–15 |
Volume of distribution (L/kg) | ~0.2 for the 3 isomers | ~0.15 | ~0.16 |
Clearance (mL/kg/min) | 30–45 | 5.3–6.6 | 5.7 |
Elimination half-life (min) Normal organ function Renal impairment Hepatic impairment | 2–2.5 3–4 3–6 | 21 21 21 | 23–30 Mild increase 23–30 |
Maintenance dose (mg/kg) | 0.1 | 0.1 | 0.01 |
Infusion dose (mcg/kg/min) | 5–8 | 10–20 | 1–3 |
Elimination route | Plasma cholinesterase (70% of succinylcholine rate) | Renal 10%; Hofman elimination 30%; ester hydrolysis 60% | Hofman elimination. No ester hydrolysis of the parent molecule |
Active metabolites | No active metabolites | No active metabolites. Laudanosine and acrylates metabolite | No active metabolites. Laudanosine and monoquaternary acrylate metabolite |
Side effects | Histamine release | Histamine release | |
Contraindications (other than specific allergy) | Butyrylcholinesterase deficiency | Hemodynamically unstable patients due to histamine release | None |
Comments | Composed of 3 isomers (cis-trans, trans-trans and cis-cis). Reversal by cholinesterase inhibitors; edrophonium for antagonism more effective during deep block | It is composed of 10 isomers. Organ-independent elimination | Cisatracurium is the cis–cis isomer of atracurium, accounting for 50% in terms of neuromuscular blocking activity of atracurium. It is approximately 4 times as potent as atracurium but does not cause histamine release. Minimal plasma laudanosine and acrylate levels |
Table 8.4
Pharmacokinetic and pharmacodynamic properties of aminosteroid nondepolarizing neuromuscular blocking agents
Vecuronium | Rocuronium | Pancuronium | |
---|---|---|---|
Class | Non-depolarizing | Non-depolarizing | Non-depolarizing |
Duration | Intermediate | Intermediate | Long |
Potency – ED95 (mg/kg) | 0.05 | 0.3 | 0.07 |
Intubating dose (mg/kg) | 0.1 | 0.6 | 0.1 |
Onset time (min) | 3–4 | 1.5–3 | 3–5 |
Clinical duration (min) | 25–50 | 30–70 | 60–120 |
Recovery index (RI25–75) (min) | 10–25 | 8–13 | 30–45 |
Volume of distribution (L/kg) | 0.4 | 0.3–0.7 | 0.2–0.3 |
Clearance (mL/kg/min) | 5 | 10 | 1.8 |
Elimination half-life (min) Normal organ function Renal impairment Hepatic impairment | 65–75 Mild increase Significant increase | 100–250 100–300 120–400 | 90–160 Increased x2 Increased x2 |
Maintenance dose (mg/kg) | 0.01 | 0.1 | 0.02 |
Infusion dose (mcg/kg/min) | 1–2 | 5–12 | 20–40 (not recommended) |
Elimination route | Renal 10–50%; Hepatic 30–50% | Hepatic 90%; Renal 10% | Renal 40–70%; Hepatic 20% |
Active metabolites | 3-desacetyl-vecuronium | 17-desacetyl-rocuronium (minimal) | 3-OH-pancuronium; 17-OH-pancuronium |
Side effects | Vagal blockade with large doses | Minimal | Vagal block (tachycardia), catecholamine release |
Contraindications (other than specific allergy) | None | None | Short surgical procedures (< 60 min); not recommended for continuous infusion |
Comments | In patients in the ICU who have renal failure, 3-desacetylvecuronium can accumulate and produce prolonged neuromuscular blockade; elimination half-life halved in late pregnancy; 3-desacetyl metabolite has 60% of the parent compound potency | Rocuronium is approximately 6–10 times less potent than pancuronium and vecuronium, respectively. Elimination half-life prolonged in ICU patient; 17-desacetyl metabolite has 20% activity | Significant accumulation, prone to residual block (3-OH metabolite has 50% activity of pancuronium); total clearance is delayed, and the duration of action is significantly lengthened by severe disorders of renal or hepatic function |
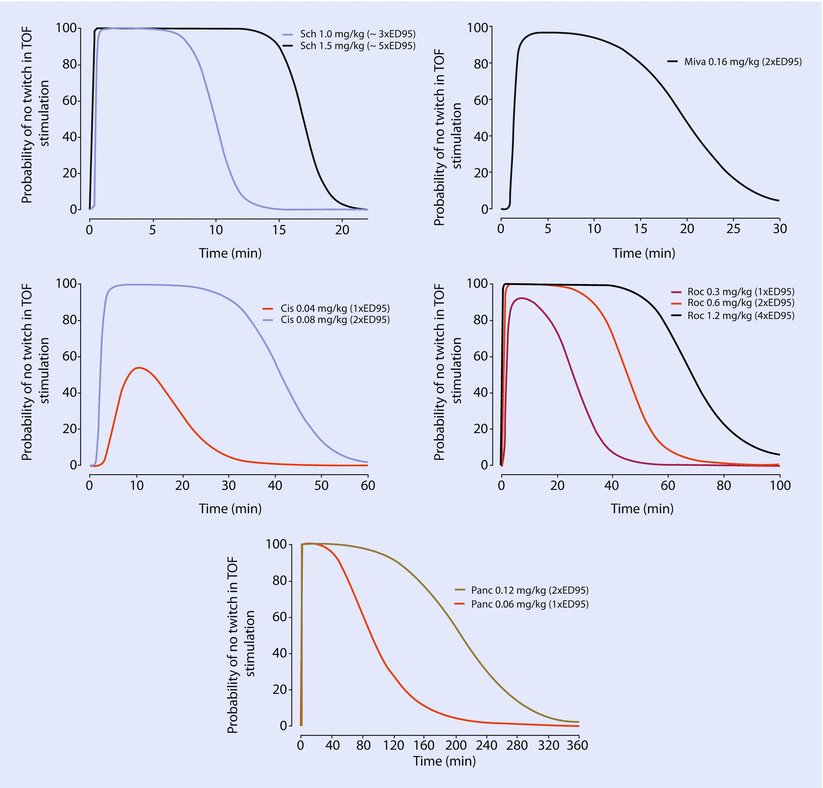
Fig. 8.3
Probability of no-twitch response to train-of-four (TOF) stimulation over time resulting from administration of different ED95 (the dose that results in 95% depression of twitch height) doses of different neuromuscular blocking drugs. Sch succinylcholine, Miva mivacurium, Cis cisatracurium, Roc rocuronium, Pancpancuronium
8.4.1 Benzylisoquinolinium Compounds
Atracurium is a bis-benzylisoquinolinium compound that is mixture of 10 isomers. The ED95 is 0.2 mg/kg, with an intubating dose 0.5 mg/kg that yields suitable laryngoscopy conditions after 2.5–4.0 min. This intermediate-duration nondepolarizing NMBA lasts 30–45 min after administration of an intubating dose. Atracurium is metabolized through 2 distinct pathways that are almost completely independent of renal and hepatic function: a nonenzymatic degradation (called Hofmann elimination) and hydrolysis by nonspecific plasma esterases. Hofmann elimination is a pH- and temperature-dependent reaction in which higher pH and temperature favor elimination. Atracurium is relatively stable at pH 3.0 and 4 °C and becomes unstable when injected into the bloodstream. Doses exceeding 0.5 mg/kg are associated with histamine release that can result in flushing, tachycardia, and hypotension. One breakdown product from Hofmann elimination, laudanosine, has been implicated in a theoretical risk of increased central nervous system excitability; however, at clinically relevant doses such complications have not been reported.
Cisatracurium is the cis-isomer of atracurium and represents about 15% of the marketed atracurium mixture by weight, but accounts for more than 50% in terms of potency or neuromuscular blocking activity. It is approximately 4 times more potent than atracurium with an ED95 of 0.05 mg/kg and, unlike atracurium, it does not cause histamine release in the clinical dose range. The administration of 2 × ED95 of cisatracurium provides intubating conditions in about 3–5 min. Like atracurium, it is broken down by Hofmann elimination, but there is no ester hydrolysis of the parent molecule. Its organ-independent elimination pathway, combined with its hemodynamic stability and lack of associated histamine release make this NMBD ideal for use in the intensive care unit (ICU). The incidence of anaphylaxis from both cisatracurium and atracurium is lower than that of rocuronium and succinylcholine.
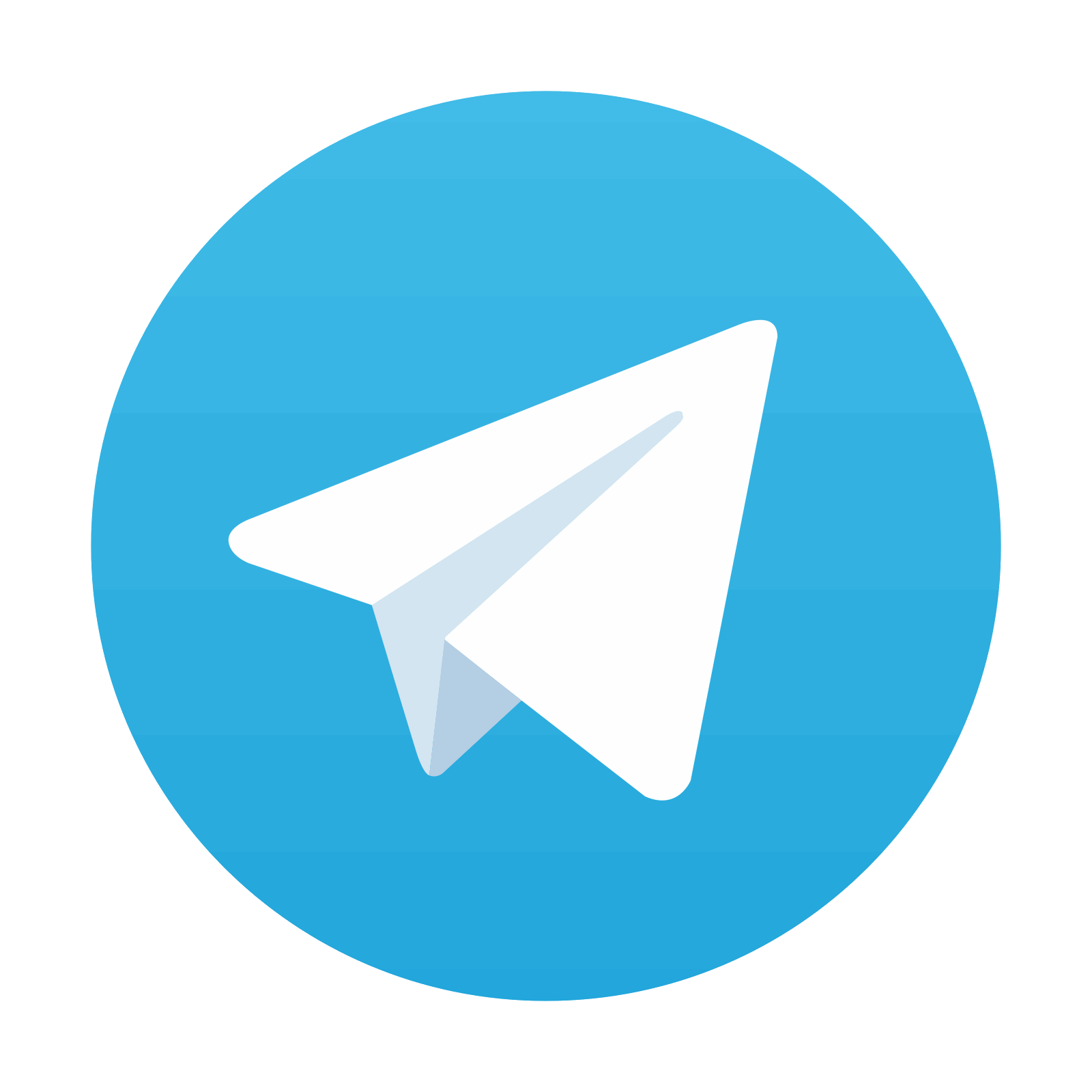
Stay updated, free articles. Join our Telegram channel

Full access? Get Clinical Tree
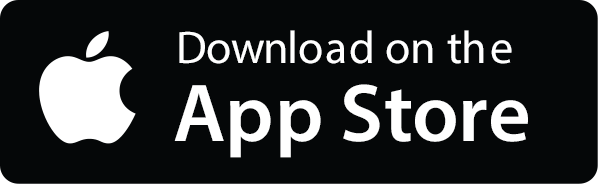
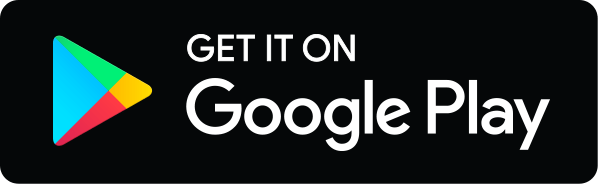