Chapter 7 PBM in Nonsurgical Settings
7.1 Potential for PBM in Intensive Care Medicine
M. Hiesmayr, A. Schiferer
7.1.1 Transfusion Practice in Intensive Care Units
▶ Transfusion rates. In intensive care units (ICUs), the transfusion of red blood cells (RBCs) is virtually universal practice. Overall, one in every three patients in intensive care is transfused (Vincent et al 2002, Sakr et al 2010). Among patients whose length of stay in the ICU is longer than 1 week, approximately three in four receive a transfusion (Vincent et al 2002, Corwin et al 2004, Vincent and Piagnerelli 2006). The transfusion rate rises sharply in line with the length of stay ( Fig. 7.1 ) and is almost twofold higher for anesthesiology/surgical ICUs than for internal medicine ICUs. More than 70 % of all transfused patients receive their first transfusion during the first 2 days in the ICU. The average transfusion volume is 1 unit of RBC concentrate every 3 days.

▶ Indications for transfusion. The indication for RBC transfusion is bleeding in 53 % to 60 % of cases, followed by a low hemoglobin value in association with a reduced physiological reserve (28–29 %), impaired perfusion (17 %), and coronary heart disease (0.5–8 %) (French et al 2002, Vincent et al 2002, Corwin et al 2004, Walsh et al 2004). There appears to be widespread variability between ICUs with regard to the hemoglobin threshold for transfusion. The threshold value is typically between 7 g/dL and 9 g/dL, and it was lower in 2002 than in 1993 (Hébert et al 2005). The transfusion threshold is much higher for patients with myocardial infarction than for patients with trauma, hemorrhage, or severe sepsis. Another important change over the years has been a considerable increase in the transfusion of single units of RBCs. In addition, more restrictive transfusion practices with a lower threshold in the ICU have led to an increase in the rate of post-ICU transfusion. Thus, transfusion decision-making should be considered as a process that requires the concerted action of clinicians from all involved specialties, with the hemoglobin value serving as a basis for discussion rather than as a definitive transfusion trigger.
▶ Hemoglobin changes over time. The hemoglobin concentration changes during the course of a patient’s stay on the ICU, often depending on the baseline value. Low admission values are usually corrected rapidly by transfusion, whereas high values decline progressively over a period of days provided that there are no acute bleeding events (Fig. 7.2a, b) (Nguyen et al 2003). This decline has been attributed to a large volume of blood draws (mean 41 mL/day) (Vincent et al 2002). The daily blood sampling volume may be twice as high for patients with more complex clinical manifestations involving infection, altered coagulation, or invasive treatments such as hemofiltration and extracorporeal membrane oxygenation. Critically ill patients, whose bone marrow function is usually impaired by inflammation, are not able to compensate for blood loss of that magnitude.

Note
Clinicians can make an important contribution to quality improvement and maintenance by regularly appraising the observed average and minimal hemoglobin values, the transfusion rate, and the timing of transfusions against usual practice. Once PBM has been introduced, patients with anemia are only transfused to values slightly above the transfusion threshold, and the steady decline in hemoglobin during a patient’s stay on the ICU will be mitigated because of the systematic application of preventive strategies.
7.1.2 Outcome of Anemia
The transfusion thresholds in line with the practice of PBM are markedly lower than the WHO criteria for anemia (WHO 1968). In physiological terms, the body responds to lower hemoglobin values with the stabilization of hypoxia-inducible factor 1α (HIF1α), which is an oxygen-sensitive transcription factor that increases the concentrations of a number of adaptive molecules (Shander et al 2011b). However, even at hemoglobin values that are higher than the transfusion threshold, there are already signs of an adverse effect on certain organ systems. Accordingly, low preoperative hemoglobin values constitute a clear risk factor for cerebral and other complications in the event of a further drop in hemoglobin, e.g., during extracorporeal circulation (Karkouti et al 2008b).
▶ Tolerance to anemia. Most observations on the tolerance to anemia have been made in patients who, for logistical or religious reasons, did not receive RBC transfusions. No rise in mortality was observed for patients with a hemoglobin value of around 8 to 8.5 g/dL, whereas patients with a postoperative value of less than 8 g/dL had a higher mortality: around 33 % if the hemoglobin value dropped to 4 to 5 g/dL, and 50 % to 60 % if the value dropped to 3 g/dL (Carson et al 2002b). There was a trend toward increased mortality from cardiovascular disease. In patients with craniocerebral trauma, a worse outcome has repeatedly been observed for mean hemoglobin values below 9 g/dL on admission (Sekhon et al 2012).
7.1.3 Treatment of Anemia (First Pillar of PBM)
In the intensive care setting, two pharmacological strategies for the conservative treatment of anemia are under discussion.
▶ Erythropoietin. Corwin et al (2007) conducted a large study on the use of erythropoietin for the stimulation of de novo erythropoiesis. In a mixed cohort of intensive care patients (surgical, medical, trauma), evidence of a statistically nonsignificant trend toward reduced mortality was found for patients treated with erythropoietin, but it was not possible to reduce the need for transfusion by administering erythropoietin. A major side effect was a higher rate of thrombosis among patients who had not received heparin-based antithrombotic treatment or prophylaxis. Apart from its impact on hematopoiesis, erythropoietin may also have a cytoprotective effect at a much higher dose than that customarily used (Hayden et al 2012).
▶ Iron supplementation. The second approach to the treatment of anemia is iron supplementation. Whereas treatment strategies based on iron supplementation exist for various patient groups, such as patients with cardiac failure (Anker et al 2009) and those undergoing dialysis, there continues to be a paucity of data on intensive care patients (Corwin et al 2007, Pieracci et al 2009). The conventional method of diagnosing iron deficiency states on the basis of ferritin and transferrin saturation is not suitable in intensive care because the blood iron parameters, which are unrelated to the body’s iron stores, fluctuate as part of the acute-phase response. Iron deficiency diagnostic tests tailored to intensive care patients should be able to identify whether individual patients could benefit from iron supplementation. Therefore, diagnostic testing should be investigated in the context of effectiveness studies to allow the definition of meaningful cutoff values and to identify potential treatment side effects, such as infections (Litton et al 2013).
Note
In the intensive care setting, erythropoietin administration and iron supplementation are strategies under discussion for the treatment of anemia. However, it is not yet clear which patients might benefit from these treatment approaches.
7.1.4 Outcome of Transfusion
▶ Observational studies. In 42 of 45 observational studies, transfusions in intensive care patients were found to be associated with a 1.5- to 2-fold higher risk of death or infection (Marik and Corwin 2008); the effects of transfusion were neutral in two studies, and they were favorable in one study in patients with acute myocardial infarction and a hematocrit below 30 % (Wu et al 2001). However, there was no benefit of transfusion if the hematocrit at admission was higher than 30 %. Similar findings were observed in patients with acute coronary syndrome, where a nadir hematocrit of around 25 % was associated with the lowest mortality (Rao et al 2004). A randomized pilot study of patients with myocardial infarction revealed that liberal transfusion practices with a target hematocrit of 30 % to 33 %, compared with 27 % to 29 %, were associated with a markedly higher rate of complications, e.g., signs of cardiac insufficiency (Cooper et al 2011). Transfusion was associated not only with transfusion-related acute lung injury (TRALI) (Gajic et al 2007), but also with higher rates of positive blood cultures (Shorr et al 2005) and nosocomial pneumonia (Shorr et al 2004).
A number of studies aimed to identify whether transfusion was an independent risk factor for a poor outcome or just a marker for the severity of disease. Based on propensity score matching, transfusions tended to have a neutral or favorable effect. However, it remains unclear why the mortality during the first 5 days was markedly higher in the nontransfused control group than in the transfused group, and why this trend then reversed up to day 30 (Park et al 2012).
▶ Liberal versus restrictive transfusion strategy. Several randomized trials compared liberal transfusion practices with hemoglobin target values above 10 g/dL with more restrictive practices based on target values of 7–9 g/dL. In a mixed group of intensive care patients, lower target values did not have a negative effect and reduced the transfusion rate by around one-half (Hébert et al 1999a). The restrictive strategy was beneficial for younger patients and those who were less seriously ill, whereas the implications for patients with myocardial ischemia were unclear. In the second randomized study, it was not possible to identify any benefit for a liberal transfusion strategy in cardiac surgery patients, but the liberal strategy was associated with a markedly higher transfusion rate of 78 % versus 47 %. In both groups, each single transfusion was associated with a 1.2-fold rise in complications or death after 30 days (Shehata et al 2012). Among patients with septic shock, the mortality at 90 days and the rates of ischemic events and use of life support were similar among those assigned to blood transfusion at a higher hemoglobin threshold and those assigned to blood transfusion at a lower threshold (Holst et al 2014). In another prospective randomized study, a restrictive transfusion threshold after cardiac surgery was not superior to a liberal threshold with respect to morbidity or health care costs (Murphy et al 2015b).
▶ Cardiac surgery patients. For the large group of cardiac surgery patients, who account for around 20 % of blood consumption, it was demonstrated that transfusion of RBC concentrates did not constitute an independent risk factor if the analysis was adjusted for the amount of blood collected from the chest drains (Dixon et al 2013). The mortality continued to rise in line with the amount of blood in the drains, regardless of whether the patient was transfused or not. The authors inferred that patients with increased bleeding developed subclinical forms of pericardial tamponade, since increased bleeding was accompanied by reduced cardiac output, a greater need for catecholamines, and elevated pulmonary artery pressure, ventilator pressure, and left ventricular filling pressure. The authors concluded that this led to delayed weaning from mechanical ventilation, delayed mobilization, and prolonged ICU stay.
Note
A Cochrane analysis (Carless et al 2010a) recommends a restrictive strategy for all patients without cardiac disease, because this can help to reduce the transfusion rate by around 33 % with no increase in the complication rate.
▶ Recommendations. The most recent clinical practice guideline (Carson et al 2012b) contains four recommendations:
Use of a restrictive transfusion strategy (hemoglobin 7–8 g/dL) for stable hospitalized patients.
Use of a restrictive transfusion strategy (hemoglobin 8 g/dL) for patients with preexisting cardiovascular disease based on clinical symptoms.
No recommendation with regard to a restrictive or liberal transfusion threshold for patients with acute coronary syndrome.
Decisions should be influenced by clinical symptoms and hemoglobin values.
7.1.5 Integrative Strategy
Each ICU must devise a strategy that helps to keep the transfusion rate to a minimum. Such a strategy reduces the risk of blood-related side effects and has financial benefits.
The cornerstone of this strategy is an essentially restrictive transfusion policy, which, however, must not lead to a delay in the stabilization of bleeding or trauma patients. Protracted shock phases must definitely be avoided, or at least kept as short as possible.
The first component of such an approach must entail the preoperative optimization of all patients prior to large elective surgical procedures. The main focus here is on the appropriate treatment of anemia based on iron supplementation (Litton et al 2013) and, possibly, the administration of erythropoietin (Karkouti et al 2005b). There is evidence to suggest that the transfusion of anemic patients one to two days before surgery could be more beneficial than intraoperative transfusion (Karkouti et al 2012).
The second component must involve the timely control of bleeding and meticulous intraoperative hemostasis. The perioperative administration of anticoagulants and platelet inhibitors should only occur on the basis of strict indication criteria and interdisciplinary agreement. Accumulation of low-molecular heparins must be avoided under all circumstances, even in patients with slightly impaired renal function.
The third component entails reducing to an absolute minimum the frequency and volume of blood draws for diagnostic and interventional purposes, since even healthy individuals can only produce 20 to 30 mL of new RBCs per day (around 1 % of the total RBC volume). The use of smaller sampling tubes and a conservative blood testing strategy helped to reduce diagnostic blood loss by up to 80 % (Harber et al 2006, Sanchez-Giron and Alvarez-Mora 2008).
A potential fourth component is the promotion of de novo erythropoiesis in the ICU. Although studies to date have not shown any clearly identifiable positive effect of systematic erythropoietin therapy on the transfusion rate, a target group approach could be taken (Hayden et al 2012). Patients exhibiting few signs of active inflammation might show a better response to erythropoietin. The same holds true for the use of iron in the ICU. There is widespread concern that iron might increase the infection rate since iron promotes the growth of several bacterial species (Pieracci and Barie 2005, Weinberg 2009, Auerbach 2014, Auerbach and Macdougall 2014). However, to date there is no evidence that this is an issue in humans, and following cardiac surgery procedures iron reduced the transfusion rate by around one-quarter (Pieracci et al 2009). However, this effect could only be identified in patients who presented with impaired erythropoiesis related to iron deficiency.
Conclusion
The cornerstone of an integrative strategy designed to reduce the transfusion rate in intensive care medicine consists of the use of a restrictive transfusion threshold of 7 to 8 g/dL. However, this must be adapted for patients who have virtually no mechanisms to compensate for ischemia.
Such an integrative strategy should comprise three components: appropriate treatment of anemia based on iron supplementation and, possibly, the administration of iron and/or erythropoietin before major elective surgical procedures; minimization of intra- and perioperative blood loss; and reduction of the frequency and volume of blood drawn for diagnostic purposes to an absolute minimum. A further component may be the promotion of de novo erythropoiesis based on erythropoietin administration or iron supplementation in the ICU.
An integrative strategy can prove successful only if its implementation is regularly assessed by practitioners appraising their own results.
7.2 Potential for PBM in Oncology and Hematology
7.2.1 Significance of the Three Pillars
The three pillars of PBM also apply to anemia in the nonsurgical setting, albeit to a varying extent. The cornerstone of the first pillar, which is also the most important pillar in the nonsurgical area, is diagnosis. In patients with iron deficiency anemia, the cause of iron loss (mainly blood loss) must be identified and eliminated. The third pillar plays only a minor role in the nonsurgical setting and is limited to optimization of the individual patient’s tolerance to anemia.
7.2.2 Diagnosis of Anemia
Unlike in the surgical setting, where anemia is primarily attributable to perioperative blood loss, anemia in oncology and hematology has a broad variety of causes. Therefore, differential diagnosis of the clinical picture is a prerequisite for effective treatment. A complete blood count, including the differential blood count and the reticulocyte count, gives a general overview. If the differential blood count shows lymphoma cells or blast cells, or if anemia is associated with leukopenia and/or thrombopenia, bone marrow cytology and histology tests should be performed.
Hyperproliferative Anemia
A high reticulocyte count is indicative of active hematopoiesis. This increased activity may be attributable to a regeneration process following blood loss or after supplementation therapy with erythropoietin, iron, vitamin B12, or folic acid. However, if the hematocrit does not rise despite a high reticulocyte count, hemolytic anemia is likely to be implicated. The next diagnostic steps include optical examination of a blood smear, Coombs test, test for paroxysmal nocturnal hemoglobinuria, and hemoglobin electrophoresis.
Note
Hemolytic anemia is associated with a high reticulocyte count.
Hypoproliferative Anemia
All other forms of anemia with a low or normal reticulocyte count are classified as hypoproliferative anemia. The mean corpuscular volume (MCV) is another parameter that points to the genesis of anemia. A low MCV is indicative of impaired hemoglobin synthesis, whereas an elevated MCV is suggestive of a nuclear maturation defect ( Fig. 7.3 ).

▶ Low mean corpuscular volume. Iron deficiency anemia in menstruating women is a classic example of hypoproliferative anemia. At an advanced stage, it is associated with a low MCV, and is diagnosed on the basis of low ferritin. However, a normal ferritin level does not rule out iron deficiency anemia. Also, a low iron level alone is not sufficient for the diagnosis of iron deficiency anemia. A definitive diagnosis is based on Berlin blue staining of the bone marrow, which is able to identify iron activity in the bone marrow. If one wants to avoid a bone marrow puncture, a rise in the hemoglobin value after a trial of iron therapy for a few weeks also serves to confirm iron deficiency. If iron deficiency anemia is ruled out in the presence of a low MCV and a high reticulocyte count, the only issue to be clarified is thalassemia. Investigation of any form of iron deficiency anemia must include gastrointestinal tests, except in the case of young women.
▶ High mean corpuscular volume. AhighMCV without reticulocytosis is found most commonly where there is damage to the liver parenchyma, and in myelodysplastic syndromes. The latter are generally associated additionally with leuko- and/or thrombopenia, whereas vitamin B12 or folic acid deficiency are rarely implicated as having a causal role. Myelodysplastic syndrome is diagnosed through bone marrow cytology testing.
Note
Bone marrow tests are needed to diagnose macrocytic anemia with low reticulocyte counts and low leukocyte and/or platelet counts.
▶ Normal mean corpuscular volume. The diagnosis is more complex if the MCV is normal with a low or normal reticulocyte count. This suggests secondary anemia or anemia of chronic disease, and calls for investigation of the thyroid and renal function, screening for chronic infection, and screening for tumors. The ferritin level is generally high and the bone-marrow iron stores are full, but iron is not sufficiently available for erythropoiesis.
7.2.3 Management of Iron Deficiency Anemia
In the Western hemisphere, iron deficiency anemia is almost always caused by acute or chronic blood loss—only rarely is it attributable to malabsorption or an extreme diet. The standard treatment comprises daily oral iron supplementation. The treatment duration is based on the severity of anemia, but treatment must be continued after a normal blood count has been achieved to quickly fill the iron stores. Treatment lasting less than 6 months rarely suffices. To permit their absorption, iron supplements must be taken in a fasting state 1 hour before a meal. Antacids, H2 blockers, and protein pump inhibitors prevent the absorption. After 3 to 4 weeks of treatment, the blood count should be verified. If the hemoglobin value has not risen, the product is not being absorbed, has not been taken, or the diagnosis was incorrect. The gastrointestinal tolerability of all iron supplements is poor. In such a case, or if the product is not being absorbed, intravenous iron supplementation is justified. A single infusion of ferric carboxymaltose can eliminate anemia and fill the iron stores; the recommended dose with hemoglobin concentrations below 10 g/dL is 1,500 mg (or 1,000 mg for more moderate forms of anemia).
Iron deficiency anemia develops slowly. That is why patients tolerate even a very low hemoglobin value surprisingly well. RBC transfusion is needed to treat iron deficiency anemia only in the event of syncopes. Iron therapy is contraindicated in the presence of iron overload. Iron supplements should also not be administered to treat inflammatory or tumor-associated anemia because they could aggravate the immune status, unless functional iron deficiency is also present. However, the latter is poorly defined (see Chapter 7.2.8).
Note
Iron deficiency anemia is the most common form of anemia in menstruating women, and should be treated with an oral iron supplement for several months.
7.2.4 Management of Vitamin-B12 and Folic-acid Deficiency Anemia
An autoimmune process directed against the parietal cells of the stomach causes pernicious anemia. In this situation, parenteral supplementation with vitamin B12 is needed. An intramuscular injection of 1 mg of vitamin B12 is administered three times weekly until the hematocrit has normalized. Afterward (and also after gastrectomy) 1 mg of vitamin B12 is administered every 6 to 8 weeks. Patients with pernicious anemia can tolerate very low hematocrit values and RBC transfusion is not indicated.
Folic-acid deficiency anemia occurs very rarely in Europe and is seen mainly in persons with severe alcohol-related disease. Oral supplementation with 5 mg daily suffices.
7.2.5 Management of Autoimmune Hemolytic Anemia
▶ Warm-reactive antibodies. Autoimmune hemolytic anemia caused by warm-reactive autoantibodies produces a positive indirect Coombs test for IgG antibodies. Hemolysis can be generally controlled with prednisolone at 1 mg/kg daily. Alternatively, high-dose dexamethasone can be administered at 40 mg daily for 4 days. If no improvement in hemolysis is seen on prednisolone, or if hemolysis can only be controlled with maintenance treatment of more than 5 mg prednisolone daily, splenectomy should be performed. Alternatively, treatment with rituximab and a glucocorticoid is possible. The required dose is unclear. Instead of the standard intravenous dose of 4 × 375 mg/m2 at weekly intervals, doses of 4 × 100 mg/m2 are also effective (Lechner and Jäger 2010). RBC transfusion should be avoided in principle since it further aggravates the immune mechanism.
▶ Cold-reactive antibodies. Autoimmune hemolytic anemia caused by cold-reactive antibodies is less common. In the Coombs test these antibodies generally only result in complement activation. They are often associated with rheumatologic disease or lymphoma. Apart from treatment of the underlying disease and protection against the cold, treatment is the same as for warm-reactive autoantibodies, but is not as effective. Infusions should be administered at body temperature.
7.2.6 Management of Renal Anemia
There is a loose relationship between the severity of renal anemia and the extent of impaired renal function. Renal anemia is caused by a decline in erythropoietin production. However, iron or vitamin B12 deficiency can also be implicated, as can impaired iron utilization due to chronic inflammation or tumor disease. Treatment is based on erythropoietin supplementation therapy, with initial subcutaneous or intravenous injection of 400 IU erythropoietin three times weekly. Then the dose and interval are chosen such that the hemoglobin concentration is between 10 g/dL and 12 g/dL (Palmer et al 2014). Persistent hemoglobin values above 12 g/dL should be avoided. Additional iron supplementation can help to improve anemia and cut back on the need for erythropoietin.
7.2.7 Management of Myelodysplastic Syndromes
Myelodysplastic syndromes are heterogeneous diseases of varying prognosis. The Revised International Prognostic Scoring System classifies the disease on the basis of cytogenetic features, blast percentage, hemoglobin value, platelet count, and neutrophil count into five risk categories (very low, low, intermediate, high, and very high) (Greenberg et al 2012). Although erythropoietin is not licensed in Europe for myelodysplastic syndromes, one-third of the patients with very low and/or low risk respond to high-dose erythropoietin. The initial dose is 3 × 10,000 IU/week given subcutaneously. If an adequate (≥ 1 g/dL) rise in hemoglobin is not observed after 6 weeks, the dose can be increased to 3 × 20,000 IU. Combination with granulocyte colony-stimulating factor (G-CSF) can help to increase the response rate further. The highest response rates are seen for endogenous erythropoietin levels of less than 100 IU/L. Levels of ≥ 400 IU/L indicate that a response to erythropoietin is very unlikely (Ludwig and Fritz 1998). If an adequate hemoglobin increase cannot be achieved either with high-dose erythropoietin alone or in combination with G-CSF, erythropoietin must be discontinued. Patients with intermediate or high risk can be treated with 5-azacytidine, leukemia induction therapy, and/or allogeneic stem cell transplantation (Alessandrino et al 2002). Patients who are not suitable candidates for this treatment, or patients who did not respond to treatment, are enrolled in a chronic transfusion program (see Chapter 7.2.10).
7.2.8 Management of Anemia of Chronic Disease
Anemia of chronic disease is characterized by impaired iron utilization. Nonetheless, diagnostic measures should always include tests for iron deficiency, vitamin B12 deficiency, and occult blood loss. Typically, serum iron levels are low and ferritin levels are high. This constellation should under no circumstances result in the misguided administration of iron supplements. Apart from the already existing iron overload of the reticuloendothelial system, there is evidence that this would weaken the body’s defense against infection. Functional iron deficiency is rare in anemia of chronic disease and difficult to diagnose. A German working group defines functional iron deficiency on the basis of the ferritin index (soluble transferrin receptor divided by the logarithm of ferritin; see Chapter 3.1.2) (Steinmetz et al 2010). The National Comprehensive Cancer Network guidelines use the term functional iron deficiency to denote a state where the serum ferritin value is lower than 800 ng/mL and the transferrin saturation is lower than 20 % (NCCN 2015). Erythropoietin can improve anemia, but there is evidence that it shortens life expectancy. Erythropoietin is therefore not recommended for the treatment of anemia of chronic disease. Causal therapy must be based on treatment of the underlying disease. If this is not possible, a chronic transfusion program should be initiated (see Chapter 7.2.10).
7.2.9 Management of Anemia during Chemotherapy
Certain cytostatic substances, in particular the platinum derivatives, give rise to anemia through bone marrow suppression and inhibition of erythropoietin production. An Austrian survey carried out in hematology and medical oncology departments identified anemia in 36 % of patients (Ludwig et al 2004). It has been unequivocally demonstrated that erythropoietin therapy for tumor patients can reduce the transfusion incidence, improve anemia, and enhance the quality of life. However, a meta-analysis of randomized trials identified a rise in thromboembolic events and a shorter life expectancy among patients with cancer who were treated with erythropoietin (Bohlius et al 2009b). The reason for this finding is not clear. Potential explanations include the association between hemoglobin values above 12 g/dL and thromboembolic events and tumor growth stimulation via erythropoietin receptors on malignant cells. There is no clear proof for either of these two theories. Erythropoietins are therefore only recommended in chemotherapy-induced anemia if treatment does not have a curative intent. Treatment is indicated at a hemoglobin concentration below 10 g/dL and the target range should be 10 to 12 g/dL. This should be achieved with the lowest possible erythropoietin dose. The additional use of iron supplementation is controversial (Rizzo et al 2008). Patients who do not respond to erythropoietin and those who are not suitable candidates for erythropoietin therapy are transfused (see Chapter 7.2.10).
Note
In nephrogenic anemia, myelodysplastic syndromes, and chemotherapy-induced anemia, erythropoietin therapy is indicated for palliative purposes only.
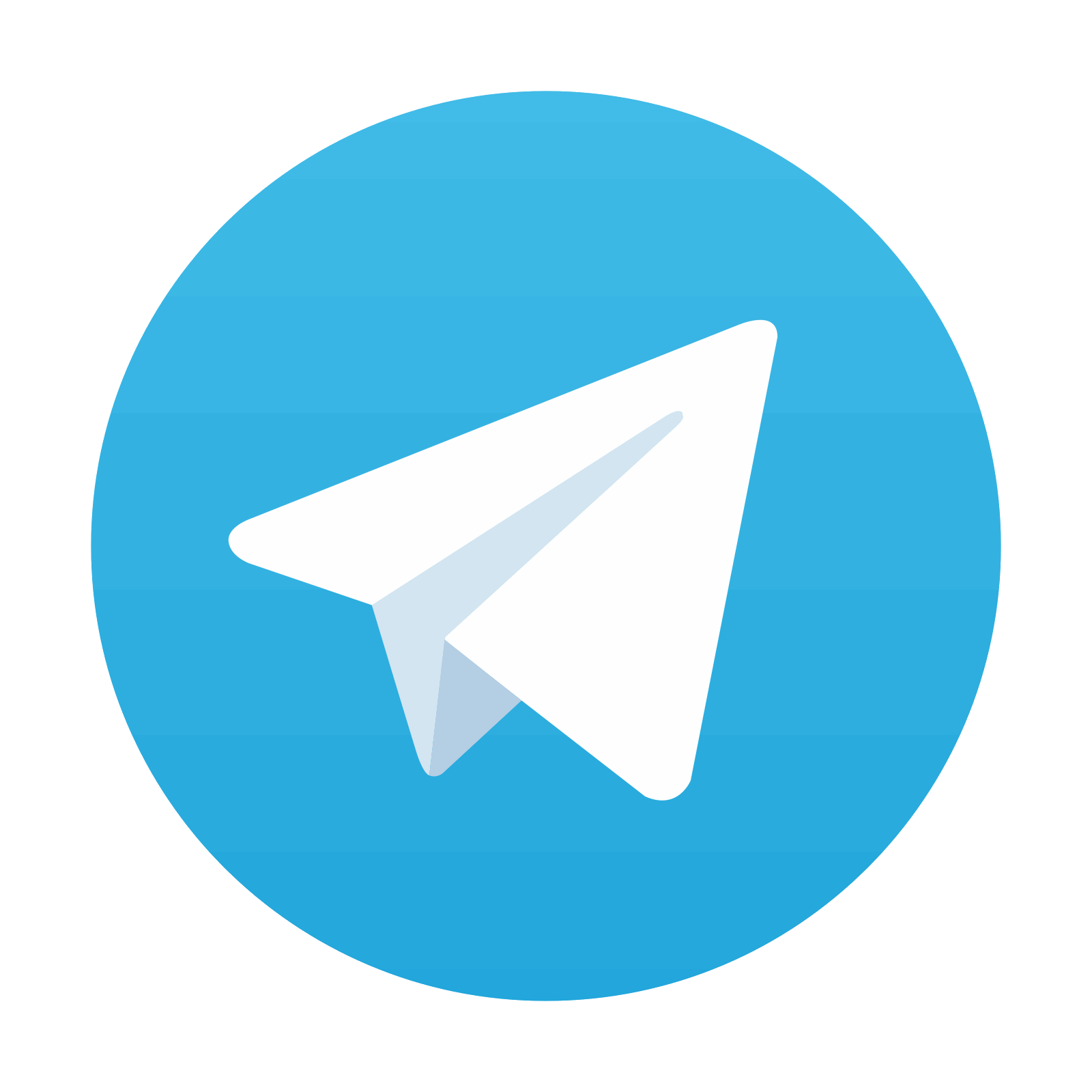
Stay updated, free articles. Join our Telegram channel

Full access? Get Clinical Tree
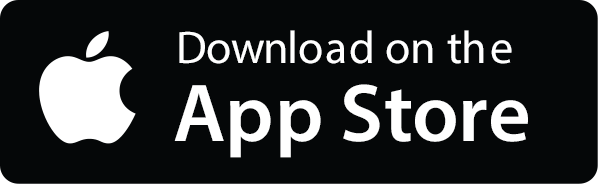
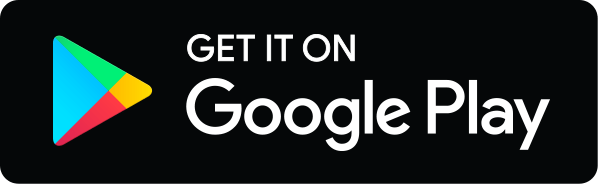
