Abstract
The liver is responsible for a wide range of strategic biochemical functions, synthesising and eliminating a huge number of molecules for a variety of purposes. A healthy liver has some important features.
The liver is responsible for a wide range of strategic biochemical functions, synthesising and eliminating a huge number of molecules for a variety of purposes. A healthy liver has some important features:
A huge physiological reserve. Even if 80% of liver is removed, it can continue to carry out all of its physiological functions.
Regeneration. In contrast to the other organs, following resection of up to three-quarters of the liver, active mitosis can regenerate a normal liver mass. This amazing ability of the liver has been used in transplantation medicine: living donor transplantation involves transplanting four segments of the right lobe (50–60% of the liver mass) from a live donor to a recipient. In the donor, the remaining liver regenerates to full size within 6–8 weeks; in the recipient, regeneration takes a little longer. Eventually, both donor and recipient have fully functioning, normal-sized livers.
Classify the functions of the liver
The functions of the liver are varied, and can be broadly classified as:
Metabolic;
Exocrine;
Endocrine;
Immunological;
Synthetic;
Hepatic clearance of drugs;
A number of additional miscellaneous functions.
What are the metabolic functions of the liver?
The liver has a vast array of metabolic functions (see Chapter 77), the most important of which are:
Carbohydrate metabolism. Many metabolic processes occur in the liver in order to maintain a normal plasma glucose concentration:
– Glycolysis. Like all other cells, the liver produces energy by transforming glucose into pyruvate.
– Glycogenesis. Following a meal, plasma glucose concentration rises, which causes insulin to be released from the pancreas. In the liver, insulin stimulates the polymerisation of excess glucose into its storage form, glycogen. Up to 100 g of glucose can be stored in this way.
– Glycogenolysis. When plasma glucose concentration falls between meals, insulin secretion is reduced and glucagon is released from the pancreas. In response to glucagon secretion, the liver releases glucose by breaking down its glycogen store.
– Gluconeogenesis. When plasma glucose concentration is low, glucagon also simulates the liver to synthesise glucose from non-carbohydrate precursors (e.g. amino acids, lactate and glycerol).
Fat metabolism. Lipid is the body’s most efficient method of energy storage. The liver is involved in lipid metabolism in a number of ways:
– Lipid breakdown. Energy is extracted from free fatty acids as they are metabolised in a process called ‘β-oxidation’ within the hepatocyte mitochondria.
– Lipid synthesis. The liver synthesises triglyceride from excess glucose. Cholesterol is also synthesised in the liver. Cholesterol is used as a structural component of cell membranes and as a precursor for steroid hormone and bile salt synthesis.
– Lipid processing. Apolipoproteins are synthesised in the liver. These are involved in the packaging of cholesterol and triglyceride as low-density lipoprotein, very-low-density lipoprotein and high-density lipoprotein.
Protein metabolism:
– Deamination. Individual amino acids have their amino groups removed by the liver. The resulting carbon skeleton (a keto acid) can be used for energy in the citric acid cycle (see Chapter 77), transformed into another amino acid or used as a substrate for gluconeogenesis.
– Urea formation. The other product of deamination is ammonia (NH3). This is detoxified in the liver through conversion to urea or glutamine.
– Amino acid synthesis. Keto acids can be transformed into non-essential amino acids by transamination, taking an amino group from one amino acid and transferring it to a keto acid, forming a new amino acid. Essential amino acids cannot be synthesised within the body and are only found in the diet.
– Protein synthesis. The liver synthesises most plasma proteins, with the exception of immunoglobulins and some hormones (see below).
What are the exocrine functions of the liver?
The liver is an important exocrine organ as it secretes bile:
Around 1000 mL of bile is produced by the hepatocytes per day. This is then concentrated to a fifth of its volume within the gallbladder.
The main constituents of bile are water, electrolytes, bile salts, bile pigment, cholesterol and phospholipids.
Bile acids are produced through the oxidation of cholesterol. Bile salts – the Na+ and K+ salts of bile acids – have an essential role in the emulsification of dietary lipid:
– Bile salts are amphipathic: they are hydrophobic at one end and hydrophilic at the other.
– Bile salts surround the dietary lipid, breaking up large fat droplets into a suspension of small fat droplets.
– Formation of these micelles is essential; without them, pancreatic lipase is unable to act on the dietary lipid in their core.
– The micelles then make contact with enterocytes, which absorb the lipid contents. The fat-soluble vitamins (vitamins A, D, E and K) are also absorbed by this micelle-mediated mechanism.
Bile is the main route of excretion of bilirubin metabolites, also known as bile pigment. The metabolism of bilirubin is complex (Figure 66.1):
– Red blood cells (RBCs) are taken up by the spleen when they get old or damaged.
– In the spleen, haemoglobin (Hb) is broken down (1) and its useful components recycled: the globin chain is broken down into its constituent amino acids and the Fe2+ ion is bound to transferrin and transported to the bone marrow for use in erythropoiesis (see Chapter 74). However, the body cannot make further use of the porphyrin ring.
– Within the macrophages of the spleen, the porphyrin ring is oxidised to biliverdin and then reduced to bilirubin (2).
– This unconjugated bilirubin is not water soluble, so is bound to albumin and transported to the liver for further processing (3).
– In the liver, the hepatocytes take up bilirubin and conjugate it to glucuronic acid, making it water soluble (4). The enzyme responsible for this process is glucuronosyltransferase.
– This conjugated bilirubin is excreted with the bile into the small intestine (5). Almost all of the conjugated bilirubin in the small intestine ends up being reabsorbed (6), transported to the liver in the portal vein and re-secreted into the small intestine; this is known as ‘enterohepatic circulation’. However, some conjugated bilirubin inevitably passes into the large intestine.
– In the large intestine, conjugated bilirubin is converted into urobilinogen by colonic bacteria (7). Urobilinogen has two fates: it may be oxidised further to urobilin and stercobilin (8) and excreted with faeces (stercobilin gives faeces its brown colour). Alternatively, urobilinogen may be reabsorbed by the gut (9) and transported via the portal vein to the liver (i.e. enterohepatic circulation). Hepatic uptake of urobilinogen is incomplete, so some reaches the systemic circulation (10) and is excreted by the kidney (11), giving urine its yellow colour.
Jaundice (plasma bilirubin concentration of >30 pmol/L) may be caused by excessive RBC breakdown or a failure of any of the excretory mechanisms described above:
Pre-hepatic jaundice – increased bilirubin as a result of increased RBC breakdown; for example, haemolytic anaemia due to blood transfusion, sickle cell crisis or malaria. The large amount of bilirubin produced far exceeds the liver’s capacity to conjugate – jaundice is therefore the result of a high plasma concentration of unconjugated bilirubin. Because it is water insoluble, no unconjugated bilirubin is filtered by the glomerulus, so urinary dipstick is negative for bilirubin.
Although anatomically within the liver, conditions involving the failure of bilirubin conjugation are often included with the ‘pre-hepatic’ causes of jaundice, such as neonatal jaundice (as a result of immature glucuronosyltransferase enzyme) and Gilbert’s syndrome (a common genetic disorder where there is reduced activity of the glucuronosyltransferase enzyme). As the problem is conjugation of bilirubin, the plasma concentration of unconjugated bilirubin is high.
Post-hepatic jaundice – failure of excretion of conjugated bilirubin in the bile; for example, as a result of a gallstone obstructing the common bile duct. When the normal route of bilirubin excretion is blocked, conjugated bilirubin instead enters the systemic circulation, resulting in a high plasma concentration of conjugated bilirubin. As conjugated bilirubin is water soluble, it is freely filtered at the glomerulus and excreted in the urine; dipstick is therefore positive for bilirubin. As conjugated bilirubin does not enter the small intestine, urobilinogen and stercobilin are never formed; faeces is therefore pale in colour.
Hepatocellular jaundice – hepatocyte necrosis and disruption of the biliary tree lead to a reduced ability to conjugate and excrete bilirubin. Causes include cirrhosis, acute viral hepatitis and paracetamol toxicity. Whether conjugated or unconjugated bilirubin predominates depends on the relative degrees of hepatocyte dysfunction compared with biliary duct disruption.
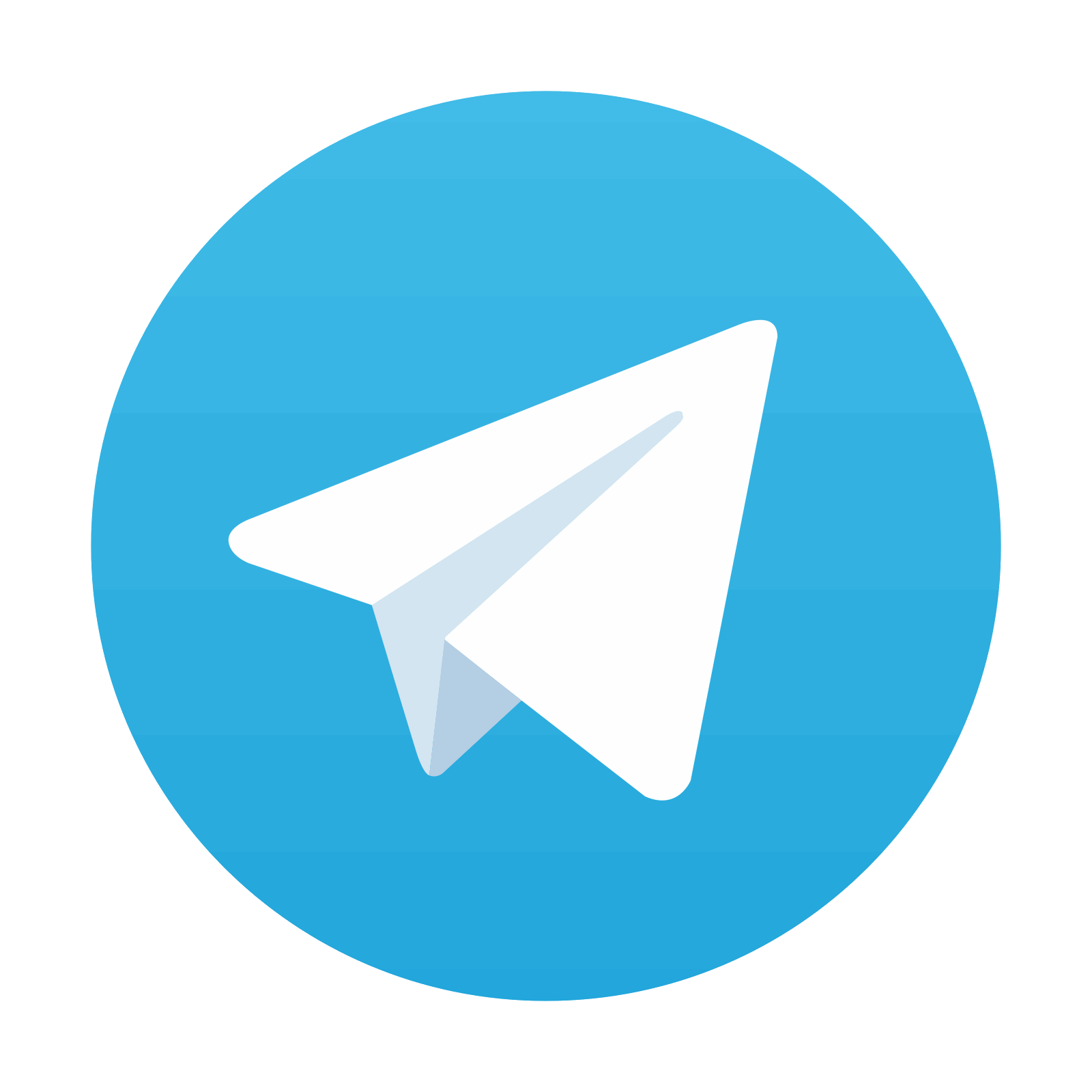
Stay updated, free articles. Join our Telegram channel

Full access? Get Clinical Tree
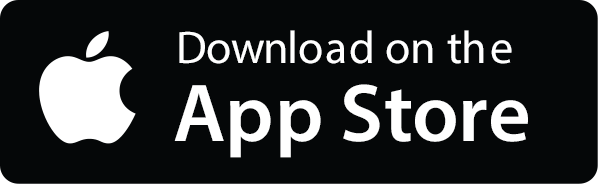
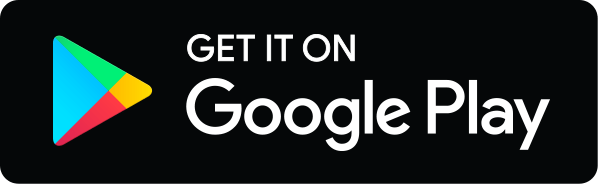
