Chapter 4 Second Pillar of PBM—Minimization of Bleeding and Blood Loss
4.1 Reduction of Diagnostic and Interventional Blood Loss
4.1.1 Iatrogenic Blood Loss
Diagnostic blood tests and interventional blood loss play an important role in the development of iatrogenic anemia among hospitalized patients (Rennke and Fang 2011, Fischer et al 2014). Like all other forms of anemia, hospital-acquired anemia is a risk factor for an unfavorable disease course (Thavendiranathan et al 2005, Musallam et al 2011). It is particularly pronounced among children, the elderly (because they often have preexisting anemia), and patients with a low body weight, and it increases in line with the severity of the underlying disease (Thavendiranathan et al 2005, Madsen et al 2000, Salisbury et al 2011). Among patients undergoing cardiac surgery, 37 % had preoperative anemia, and anemia was more severe if coronary angiography was performed prior to surgery (Karski et al 1999). Furthermore, unnecessary blood draws are a major burden on a hospital’s health care budget (Vegting et al 2012). Laboratory tests should only be performed if their results will have therapeutic consequences. However, in reality, laboratory tests are often ordered on a routine basis, without any evidence to support their usefulness and without any treatment implications (Sood et al 2007).
▶ Distribution of laboratory tests. Blood draws are more frequent when the patient is first admitted to hospital, and their frequency declines progressively in line with the length of hospital stay, and especially when the patient is admitted to the intensive care unit (ICU). This decrease in frequency is noted most commonly among patients undergoing cardiac surgery, patients receiving long-term respiratory ventilation, patients with coagulation disorders, and patients undergoing multiple surgical procedures. Hospital laboratories play a part in over 60 % of all medical diagnoses. Outside ICUs, 50 % of blood draws are for blood chemistry investigations, 32 % for hematologic tests, and 18 % for coagulation tests. By contrast, blood gas analysis accounts for the majority (63 %) of all blood draws in ICUs ( Fig. 4.1 ).

▶ Study findings. A comparison of trauma patients with a historical control group showed a marked and costly rise in the number of laboratory tests, without any impact on the outcome variables studied (Branco et al 2012). A study involving a pediatric ICU revealed that the blood volume drawn was around twice that needed for the respective laboratory test; the reasons for the blood tests were not investigated (Valentine and Bateman 2012). For premature neonates, the sampled blood volume correlated with the transfused blood volume (Obladen et al 1988). By contrast, the WHO recommends a safe blood sample volume limit of up to 3 mL/kg/day for pediatric patients involved in clinical research (Howie 2011).
▶ Variability in the frequency of blood tests. As with allogeneic blood consumption, the frequency of diagnostic blood tests, and accordingly the amount of blood drawn, varies widely between different physicians, wards, and hospitals. However, it is not always possible to identify a plausible link between this frequency and therapeutic measures, let alone therapeutic consequences (Katz et al 2011). A pilot study carried out within the framework of the Second Austrian Benchmark Study revealed that there was enormous variability in the volume of diagnostic and interventional blood loss (25–75 mL per day and patient) in four comparable ICUs ( Fig. 4.2 ). This variability could not be explained on the basis of the patients’ conditions. The hemoglobin values of the patients became more similar over the course of their ICU stay. Patients whose baseline hemoglobin value was high saw their value decline because of frequent blood draws or because of their illness, whereas those with a low baseline hemoglobin were maintained at an acceptable level or their values were increased mainly through allogeneic red blood cell (RBC) transfusions (Vincent et al 2002).

Note
Frequent blood tests cause iatrogenic anemia, which can have a negative effect on the disease course.
Overall, the diagnostic blood loss volume in routine practice is probably much too high and may have negative implications for the patients concerned. In general, a balance should be struck between the blood volume drawn and the potential risk to the patient (Raheman 2009).
4.1.2 Strategies to Avoid Diagnostic Blood Loss
A number of strategies can help to reduce diagnostic blood loss. However, the use of a single method alone is unlikely to prove successful. Only by combining several methods will it be possible to reduce the blood volume drawn by 50 % or more, depending on the baseline value. In addition to reducing the rate of hospital-acquired anemia, this will lead to considerable cost savings.
Evaluation of Blood Draw Volume and Test Frequency
The volume of blood drawn as per current practice must first of all be identified to be able to optimize blood sampling (minimizing the blood draw volume without putting the patient at risk) (Mukhopadhyay et al 2010, Valentine and Bateman 2012, Page et al 2013). Simply being aware of this issue can already bring about a considerable reduction in the blood draw volume and the transfusion requirement (Fowler and Berenson 2003). Likewise, the purpose of laboratory tests should be considered. In ICUs, physician-requested, symptom-based laboratory testing is generally superior to routine testing, since routine tests are often continued for longer than needed. The blood volume drawn is also strongly correlated with the number and functional design of indwelling arterial and venous lines. The more lines there are and the easier it is to draw blood (e.g., if the lines are large), the more blood will be withdrawn.
Other interventions include the restriction of laboratory tests to those medically indicated (avoidance of requests that cannot be medically justified), identification and discussion of inappropriate blood tests and their implications for the patient, and feedback on the impact of newly introduced measures (audits, amended request forms, etc.) (Calderon-Margalit et al 2005, May et al 2006). It has been possible to reduce the number of ordered laboratory tests by half through the implementation of lectures and supervision (Attali et al 2006). Likewise, generous type-and-screen testing and blood product ordering practices should be scrutinized. After all, around two-thirds of all RBC concentrates issued for elective surgery are not transfused (Attali et al 2006, Gombotz et al 2007).
Reduction of the Blood Draw Volume
The blood draw volume can be reduced by half or more simply by switching from large-volume to pediatric blood sample tubes (Gleason et al 1992, Jung and Ahn 2013). Adult tubes can hold up to 7.5 mL of blood, but this amount is not always needed for a blood test. It is also unclear how much blood has to be discarded, for example from a heparinized line, to obtain reliable laboratory results. The recommendations differ, ranging from the system’s dead-space volume plus 2 mL to 2.5 times the dead space. This means that around 2.5 mL have to be discarded for arterial lines. The use of smaller syringes (1 mL) also precludes the sampling of larger blood volumes. It may also be necessary to manually fill sampling tubes with a smaller volume. A further subject of debate is whether the blood drawn before sampling should be retransfused rather than discarded. Counter-arguments include the risks of infection, activation of blood coagulation, and hemolysis.
Evaluation of the Need for a Laboratory Test
This is an important point since unnecessary laboratory tests are often continued for days simply as a matter of routine. Based on a conservative estimate, up to 50 % of all laboratory results are not taken into account in routine clinical practice. A regular review does not only help to reduce diagnostic blood loss, but it might also result in considerable savings. The argument that several tests can be performed from a single blood sample and that the cost for additional tests is then negligible only holds true if the request does not include expensive, usually modern, parameters (e.g., procalcitonin, troponin).
Point-of-care Testing
Point-of-care methods such as thromboelastography generally call for specific experience and knowledge, but confer a number of advantages over routine laboratory tests (Weber and Zacharowski 2012). They are timely, carried out on site, and paid more attention thanks to their immediacy; they permit quick decision-making but are generally associated with considerably higher reagent and material costs. For methodological reasons, their analytical sensitivity and specificity are also lower. Besides, a greater blood volume may be needed than in the case of routine laboratory tests.
Switch to Noninvasive Methods
A large number of noninvasive, mainly continuous, methods have become available for the measurement of vital parameters. After initial alignment with their standard laboratory counterparts, these methods can be used for continuous routine monitoring. They include the assessment of blood sugar, hemoglobin, oxygen, and carbon dioxide. The benefit of continuous monitoring is that it provides for an earlier detection of pathologic fluctuations, and accordingly for earlier intervention. However, only a guess can be ventured at present as to what extent such noninvasive methods are able to reduce, or even replace, diagnostic blood tests.
Note
A marked reduction in the volume of diagnostic blood loss can only be achieved by combining several methods. These include reducing the blood draw volume and the frequency of blood tests, point-of-care testing, and using modern noninvasive diagnostic procedures instead of blood tests.
4.1.3 Strategies to Avoid Interventional Blood Loss
Blood loss during interventional investigations plays an additional role in hospital-acquired anemia. It is one of the reasons why preoperative anemia is more common among patients who have recently undergone coronary angiography than among those who have undergone orthopaedic surgery (Gombotz et al 2007). The volume of interventional blood loss is also strongly correlated with the duration of the investigation.
Since interventional blood loss can occur in many medical disciplines, multidisciplinary cooperation is often needed to reduce such blood loss. This is particularly the case when frequent and different interventions are prescribed by different medical specialists. Even if the blood loss associated with an individual intervention appears to be negligible, the total blood loss from several interventions can be of clinical importance. Therefore, a single multidisciplinary approach to PBM must be formulated for all specialisms involved. This includes—whenever possible—the timely discontinuation of anticoagulants and platelet aggregation inhibitors. However, the frequent practice of routinely increasing the anticoagulation parameters in ICU patients is pointless, at least in the case of nonbleeding patients, and only results in increased costs (Gajic et al 2006). In such patients, any invasive investigations should be carried out by experienced physicians.
Note
Interventional blood loss can only be reduced using a multidisciplinary approach.
Conclusion
Diagnostic and interventional blood loss can be reduced using a multidisciplinary combination of different approaches. These include evaluation of the need for individual laboratory tests, restriction of laboratory diagnostic tests to those medically indicated, reduction of the blood draw volume, point-of-care testing, and use of modern noninvasive diagnostic procedures instead of blood tests.
4.2 Coagulation Management
4.2.1 Introduction
Interdisciplinary cooperation is needed in the event of perioperative bleeding complications to limit blood loss, reduce the transfusion rate of allogeneic blood products through effective coagulation management, reduce the incidence of thromboembolic complications, and—equally important—curtail the primary and secondary costs of hemotherapy.
By taking a standardized coagulation history, it will be possible to identify existing preoperative coagulation disorders and to draw up an individual bleeding risk profile tailored to the planned surgical procedure. Coagulation management should be based on hemotherapy algorithms that take account of both the individual bleeding risks (e.g., medication containing acetylsalicylic acid or antiplatelet agents) and the causes of bleeding specific to a given patient group (e.g., anticoagulation for procedures involving extracorporeal circulation).
The key elements of modern coagulation management are the timely and comprehensive analysis of any underlying coagulation disorder, followed by its targeted treatment. Compared with conventional laboratory tests, point-of-care coagulation testing enables a more rapid and comprehensive analysis of the causes of an existing coagulopathy. The incorporation of point-of-care coagulation testing into hemotherapy algorithms can help to reduce perioperative blood loss and transfusion of allogeneic blood products.
4.2.2 Successive Escalation of Treatment for Coagulation Disorders
Surgical Hemostasis/Permissive Hypotension
Any initial surgery-induced bleeding can lead to coagulopathic bleeding in the ensuing course. If there is loss of volume, the infusion of crystalloids and/or colloids needed for the maintenance of normovolemia can induce dilutional coagulopathy. Therefore, surgical/interventional hemostasis is a fundamental prerequisite and an important key element for the prophylaxis and effective treatment of perioperative blood coagulation disorders. Provided that there are no contraindications, restrictive volume therapy and permissive hypotension (mean arterial blood pressure 60–65 mmHg) are temporary treatment options to reduce blood loss until surgical hemostasis is achieved.
Note
Effective hemotherapy is impossible without surgical/interventional hemostasis.
Maintenance of an Optimal Physiological Environment for Hemostasis
pH Value
Acidosis affects primary hemostasis and can reduce the adhesion and aggregation of platelets. It also restricts plasma coagulation, thus reducing the speed at which thrombin—a key enzyme in the coagulation cascade that is essential for the formation of blood clots—is formed. As buffering can only partially reverse an acidosis-induced coagulation disorder, the prevention of acidosis should have top priority in everyday clinical practice.
Temperature
Hypothermia (body temperature < 35 °C) reduces the hemostatic potential of primary hemostasis and the plasma coagulation system. Coagulation disorders resulting from hypothermia-induced thrombocytopathy can be aggravated by platelet sequestration in the spleen and liver.
Calcium
Calcium is an essential cofactor in all phases of blood coagulation. Among other things, it mediates complex formation between the negatively charged phospholipids on the surface of wounds and the likewise negatively charged coagulation factors II, VII, IX, and X, thus promoting the accumulation and activation of coagulation factors at the site of vascular injury. As there is an inverse relationship between lactate and calcium, metabolic acidosis can give rise to clinically relevant hypocalcemia. Besides, the free calcium concentration can be reduced through chelate formation with the citrate that is contained in allogeneic blood products for anticoagulation purposes. Hypocalcemia secondary to major blood loss and massive transfusion must be treated in a timely fashion. The plasma concentration of ionized calcium should be > 1 mmol/L.
Hematocrit
RBCs have multiple effects on blood coagulation. They circulate in the middle of blood vessels, pushing the smaller platelets toward the vessel wall and thus toward the site of injury. Besides, RBCs have a role in providing phospholipids, ADP, and thromboxane A2, and they are catalysts in the process of blood clotting. As oxygen carriers, RBCs help to maintain tissue oxygenation and prevent acidosis. It is recommended that the hematocrit should not drop below around 25 % if there is acute bleeding.
Note
Continuous correction of the basic physiological parameters required for hemostasis is the first treatment step.
Treatment Step: Antifibrinolytic Therapy
▶ Hyperfibrinolysis. The systemic activation of fibrinolysis (hyperfibrinolysis) can lead to life-threatening bleeding through the dissolution of blood clots and the consumption of circulating fibrinogen. Polytrauma patients, patients undergoing extracorporeal circulation, and those undergoing surgery of organs that are rich in tissue plasminogen activator, such as the lungs, adrenal glands, prostate gland, or uterus, are at higher risk for the development of hyperfibrinolysis. Hyperfibrinolysis can also be associated with a tumor, caused by hyperthermia or hypothermia, or induced by medication. Classic laboratory parameters, such as D-dimer, are not suitable for the diagnosis of hyperfibrinolysis or the monitoring of its treatment; rather, viscoelastic methods such as thromboelastography or thromboelastometry represent the gold standard for the diagnosis of hyperfibrinolysis (Luddington 2005).
▶ Tranexamic acid. Since the restriction of aprotinin use in 2008, the lysine analogue tranexamic acid has become the most commonly used antifibrinolytic drug. Its early administration in trauma patients reduced the mortality without increasing the risk of undesirable side effects such as thrombosis (Shakur et al 2010). Any hyperfibrinolysis must be treated before the transfusion of fresh frozen plasma (FFP) or the administration of another form of hemotherapy; otherwise the administered factors will immediately be consumed. A clinical suspicion of hyperfibrinolysis—even without viscoelastic confirmation—is sufficient to justify early treatment with tranexamic acid, especially in trauma patients. The dosing recommendations vary, ranging from a single bolus dose to continuous administration.
Note
If there is diffuse bleeding, the early administration of tranexamic acid is recommended even without thromboelastographic confirmation of hyperfibrinolysis.
Treatment Step: Replacement of Deficient Coagulation Factors
The infusion of crystalloids or colloids for the maintenance of normovolemia can induce dilutional coagulopathy. Besides, coagulation factors are activated and consumed in a bleeding situation. Factor concentrates and FFP are available to treat coagulation factor deficiencies.
Fibrinogen Concentrates
Fibrinogen—a ligand of the platelet glycoprotein IIb/IIIa receptor—promotes blood clotting and determines the mechanical stability of the clot (Levy et al 2012). The average plasma concentration of the acute-phase protein fibrinogen is around 150 to 300 mg/dL; it can rise to 400–600 mg/dL toward the end of pregnancy. Although fibrinogen accounts for 85 % to 90 % of the total mass of plasma coagulation factors, it reaches its critical concentration sooner than most other coagulation factors in a bleeding or dilutional situation (Hiippala et al 1995). The target value (measured for example with the Clauss method or with a viscoelastic method, e.g., the FIBTEM test) in bleeding situations has not been clearly defined. Studies have demonstrated that the propensity for bleeding is greater at a plasma concentration of < 150–200 mg/dL or with maximum clot firmness of < 8 mm according to the FIBTEM test.
German and European guidelines recommend that the plasma fibrinogen concentration should not drop below 150–200 mg/dL in massive bleeding situations (Rossaint et al 2010). The fibrinogen concentration can generally be increased through the transfusion of FFP or the administration of fibrinogen concentrates. The administration of fibrinogen concentrates to increase the fibrinogen concentration has proved to be a rapid, safe, and effective measure. This is particularly true for the treatment of bleeding at the end of pregnancy, because the required fibrinogen concentration cannot be achieved with FFP.
Prothrombin Complex Concentrates
Prothrombin complex concentrate (PCC) is a factor concentrate containing the coagulation factors II, VII, IX, and X (the prothrombin complex); depending on the manufacturer, PCC also contains varying concentrations of proteins C and S as well as small amounts of antithrombin and heparin. PCC is licensed for the treatment and prophylaxis of bleeding caused by hereditary or acquired factor deficiency. This applies for instance to patients with a low prothrombin time, for example because of cirrhosis of the liver, treatment with coumarin derivatives, or acute blood loss. Administration of 1 IU/kg PCC raises the coagulation factor concentration by around 1 %. For diffuse bleeding, PCC should be given at a dose of at least 25 IU/kg. The combination of PCC with antithrombin III, which was standard practice a few years ago, is no longer recommended.
Factor XIII
Factor XIII stabilizes blood clots through the cross-linking of fibrin and the integration of proteins with antifibrinolytic activity (e.g., α2-antiplasmin) into the clot. There is no clearly defined lower reference range. However, it is recommended that the factor XIII concentration should not drop below 60–70 % in bleeding situations (Gerlach et al 2002). The factor XIII concentration can be increased with FFP or factor concentrate. Since neither conventional laboratory analysis nor thromboelastometry are suitable for the diagnostic evaluation of a potential factor XIII deficiency, specific factor analysis should be carried out in cases of doubt.
Fresh Frozen Plasma
In the majority of cases, the administration of fibrinogen concentrate and PCC is sufficient to assure adequate clot formation in patients with coagulopathy. However, if there is major blood loss and a massive transfusion is required, a deficiency of factors V, VIII, and XI can occur, which cannot be treated with concentrates. In such cases, FFP transfusion appears to be indicated. FFP should be transfused only at a dose with hemostatic efficacy (at least > 15 mL/kg) (BAEK 2011a). The indication for FFP transfusion should be based on strict criteria, since FFP transfusion is associated with a significant rise in the incidence of acute respiratory failure, nosocomial infections, sepsis, and multiple organ failure (Watson et al 2009).
Coagulation Therapy Based on Factor Concentrates versus Fresh Frozen Plasma
A number of retro- and prospective studies, predominantly among patients undergoing cardiac surgery or general surgery, have shown that selective coagulation therapy with coagulation factor concentrates is superior to the nonspecific treatment with FFP, with the exception of situations involving massive transfusion.
Note
Coagulation factor deficiencies can be treated with factor concentrates or FFP. With the exception of situations involving massive transfusion, selective coagulation therapy with coagulation factor concentrates appears to be superior to nonspecific therapy with FFP.
Treatment Step: Optimization of Primary Hemostasis
In bleeding situations, platelets tend to reach their critical limit of 50,000–100,000/µL only at a late stage (Hiippala et al 1995). However, the hemostatic potential of primary hemostasis can be reduced even before the onset of thrombocytopenia. The presence of impaired platelet function and a patient’s individual bleeding dynamics can necessitate the optimization of primary hemostasis, regardless of the current platelet count.
Desmopressin
Administration of the vasopressin analogue 1-deamino-8-D-arginine vasopressin (desmopressin; dose: 0.3 µg/kg) leads to a threefold increase in the concentrations of von Willebrand factor and factor VIII (Cattaneo 2002), and can improve platelet adhesion and aggregation. As such, desmopressin represents a drug-based treatment option for the optimization of primary hemostasis. It has relatively few side effects and is more cost-effective than the transfusion of platelet concentrates. Since desmopressin can induce fibrinolysis, it is often used in combination with tranexamic acid.
Transfusion of Platelet Concentrates
The transfusion of platelet concentrates is often inevitable if there is major blood loss and thrombocytopenia. A rise in the platelet count by 20,000–30,000/µL can be expected following the transfusion of platelet concentrate. When transfusing platelet concentrates, particular attention should be paid to the legal restrictions on the shelf life, since the infection risk rises proportionally to the shelf life and the hemostatic potential of platelets declines from the time of harvesting (Cauwenberghs et al 2007).
Note
In addition to the transfusion of platelet concentrates, a drug-based treatment option (administration of desmopressin) is available for the optimization of primary hemostasis.
Treatment Step: Ultima Ratio
If life-threatening coagulopathy persists after the implementation of surgical or interventional hemostatic measures, the optimization of primary hemostasis, and adequate substrate replacement (fibrinogen > 200 mg/dL, platelet count > 100,000/µL), the administration of recombinant activated factor VII (rVIIa; NovoSeven, Novo Nordisk; dose: 90 µg/kg) constitutes the treatment option of last resort (Vincent et al 2006). However, meta-analyses of prospective randomized studies did not find any evidence for a positive effect on the rate of allogeneic blood transfusion or the perioperative mortality. They did, however, identify a higher incidence of severe thromboembolic events following rVIIa administration (Lin et al 2011). For this reason, rVIIa should only be administered in life-threatening situations.
Note
The administration of rVIIa represents the treatment option of last resort and should be governed by particularly stringent criteria.
4.2.3 Diagnosis of Coagulopathy: Conventional Laboratory Analysis versus Point-of-care Methods
The standard laboratory tests used for the diagnosis of coagulation disorders (activated partial thromboplastin time, international normalized ratio, platelet count, fibrinogen concentration) have several diagnostic shortcomings. Cell–cell interactions, the effects of hypothermia and acidosis, and fibrin polymerization disorders are not sufficiently captured in vitro. It is also impossible to gain insights into the clot stability. The positive predictive value and the specificity are low with regard to the diagnosis of perioperative bleeding. The time delay between a blood draw and the availability of the results can mean that inadequate hemostatic treatment is initiated (Toulon et al 2009).
National and international guidelines therefore state that conventional laboratory analysis is not adequate for the diagnosis of acute bleeding or the monitoring of its treatment (Lier et al 2011), and they point out that the treatment algorithms for blood coagulation disorders should include point-of-care coagulation testing. Several prospective randomized studies concluded that the incorporation of point-of-care methods in hemotherapy algorithms led to a reduction in the rate of allogeneic blood transfusion (in particular the transfusion of FFP and RBC concentrates). A prospective randomized trial of cardiac surgery patients with a coagulation disorder demonstrated that hemotherapy based on point-of-care testing compared with hemotherapy based on conventional coagulation laboratory analysis led to a reduction in the RBC transfusion rate (Weber et al 2012). Analysis of the secondary outcome parameters revealed that the transfusion rate for FFP and platelet concentrates was significantly lower in the point-of-care group. Furthermore, the duration of postoperative ventilation and the duration of intensive care treatment were significantly shorter in the point-of-care group. The postoperative blood loss, incidence of postoperative kidney failure, 6-month mortality, and costs incurred for hemotherapy were lower in the point-of-care group (Weber et al 2012).
Point-of-care Methods
A broad spectrum of different methods are available for point-of-care coagulation testing. These range from simple strip tests to procedures of such complexity that their performance, and the interpretation of the results, should be entrusted only to experienced and trained personnel. These methods can be implemented in patient care at the pre-, intra-, or postoperative stage ( Fig. 4.3 ). In addition, the complex process of blood coagulation cannot be reproduced by a single point-of-care device. A combination of several methods is often needed for a comprehensive diagnosis of complex coagulation disorders.

Thromboelastometry and viscoelastic methods are based on the technique of thromboelastography presented by Hartert (1951). Following activation of the coagulation cascade in vitro, parameters such as the time to onset of clot formation, the clotting speed, and the clot firmness and stability in relation to time are analyzed ( Fig. 4.4 ). Aggregometry methods are used for the point-of-care analysis of primary hemostasis, especially for the evaluation of platelet function. By combining aggregometry and viscoelastic methods, it is possible to obtain a diagnostic spectrum that greatly surpasses that of conventional laboratory coagulation tests.

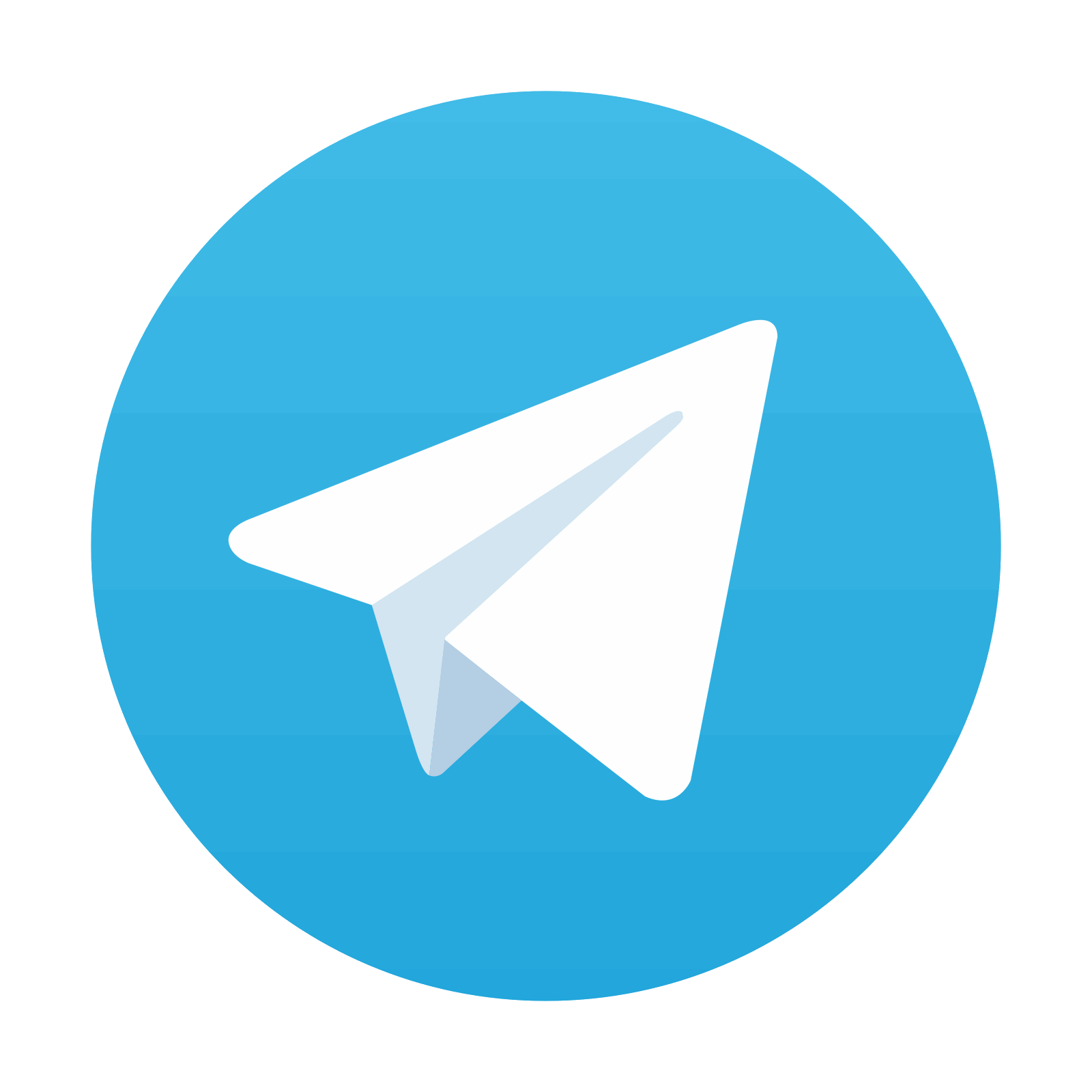
Stay updated, free articles. Join our Telegram channel

Full access? Get Clinical Tree
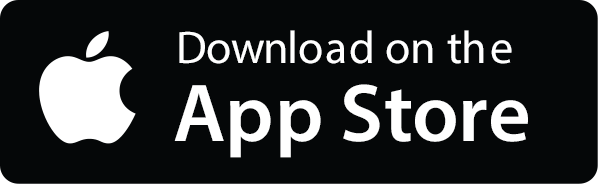
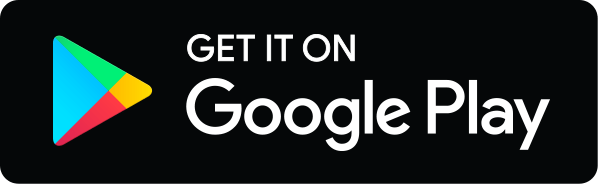
