Summary
A primary survey is performed immediately on admission to evaluate and treat life-threatening injuries such as hemorrhage, airway, and brain injuries. Hemorrhage leads to 40% of trauma-related deaths, particularly in the first 24 hours. Thromboelastography (TEG), rotational thromboelastometry (ROTEM), complete blood count, fibrinogen, prothrombin time (PT), activated partial thromboplastin time (aPTT), and international normalized ratio (INR) should be obtained to evaluate coagulation targeted to obtain clot stability [1]. It is also essential to measure renal and liver function, as both affect coagulation and drug elimination [2].
Trauma Patient Evaluation
A primary survey is performed immediately on admission to evaluate and treat life-threatening injuries such as hemorrhage, airway, and brain injuries. Hemorrhage leads to 40% of trauma-related deaths, particularly in the first 24 hours. Thromboelastography (TEG), rotational thromboelastometry (ROTEM), complete blood count, fibrinogen, prothrombin time (PT), activated partial thromboplastin time (aPTT), and international normalized ratio (INR) should be obtained to evaluate coagulation targeted to obtain clot stability [Reference Foster, Sappenfield, Smith and Kiley1]. It is also essential to measure renal and liver function, as both affect coagulation and drug elimination [Reference Thomas and Makris2].
Hemostasis and the Coagulation Cascade
The vascular endothelium is designed to regulate blood flow and plays an active role in hemostasis to induce thrombosis and activate the coagulation cascade. Intact vasculature is lined by the endothelial glycocalyx (EGC), a supportive layer of glycoproteins, glycosaminoglycans, and proteoglycans. It supports vascular permeability, fluid homeostasis, immunomodulation, and hemostasis [Reference Chignalia, Yetimakman and Christiaans3, Reference Brohi, Singh, Heron and Coats4]. Hemostasis is achieved by activating the cascade of the intrinsic and extrinsic pathways, platelet activation, and disruption of the vascular endothelium.
Primary hemostasis is initiated by tissue trauma exposing the vascular endothelium and disrupting the EGC. Sheer stress on the endothelium results in increased release and binding of circulating von Willebrand factor (vWF) on its surface to glycoproteins on the exterior of platelets. After binding to the endothelium, platelets release hormones and proinflammatory substances, inducing platelet aggregation and initial clot formation.
The clotting cascade is set in motion by the extrinsic pathway, commonly known as the tissue factor (TF) pathway, and the intrinsic pathway, also known as the contact activation pathway (CAP). Trauma and the resultant exposure of TF lining the vasculature lead to binding and activation of factors V and VII, further propagating the coagulation cascade. Factor VII encounters TF, forming a complex that initiates the extrinsic pathway via conversion of factors IX and X to their activated forms.
Fibrinogen, an acute phase glycoprotein synthesized in the liver, is the precursor to fibrin, a key factor in the coagulation cascade. Activated factors IXa and Xa generate thrombin, which converts fibrinogen into fibrin, leading to further clot propagation and stabilization [Reference Yee and Kaide5, Reference Grottke, Mallaiah, Karkouti, Saner and Haas6]. Fibrin is necessary for clot strength and stability, prevention of fibrinolysis, and promotion of platelet aggregation [Reference Levy and Goodnough7].
The CAP begins with collagen exposure on the basement membrane of damaged blood vessels, forming another elaborate complex with factor XII and other inflammatory markers and progressing towards the common pathway. Minimal fibrin is formed via this route; therefore, it is less important for secondary hemostasis [Reference Yee and Kaide5]. Both coagulation routes converge into the common pathway, which is initiated by factor X.
Coagulopathy in Trauma
Coagulopathy in trauma patients is multifactorial. Extrinsic factors are activated in traumatic brain injury, acidosis, inflammation, hypothermia, and consumptive coagulopathies. Traumatic injury, hypoperfusion, and tissue damage result in trauma-induced coagulopathy (TIC). This condition affects approximately 25–30% of trauma patients and is directly related to the Injury Severity Score (ISS) [Reference Brohi8]. Risk factors for the development of TIC include a base deficit <−6 mEq L−1, temperature <35°C, prehospital crystalloid administration, and head injuries (Glasgow Coma Scale score <6) [Reference Brohi, Singh, Heron and Coats4, Reference Simmons, Pittet and Pierce9, Reference Davenport and Brohi10]. TIC occurs at the onset of traumatic injuries, as patients exhibit prolonged PT and partial thromboplastin times immediately following the event prior to the start of resuscitation [Reference Brohi8]. These patients have higher transfusion requirements, longer hospital lengths of stay, higher incidences of organ dysfunction, and up to four times higher overall mortality risks [Reference Brohi, Singh, Heron and Coats4]. The mechanisms behind TIC are not fully understood but likely stem from a combination of tissue injury and the resulting endothelial and EGC dysfunction causing an impaired coagulation response, combined with a hyperfibrinolytic state.
Tissue injury, sympathetic upregulation, inflammation, and hypoperfusion result in disruption and breakdown of the vascular endothelium and EGC [Reference Brohi8, Reference Moore, Moore, Chapman, Gonzalez and Sauaia11–Reference Astapenko, Benes, Pouska, Lehmann, Islam and Cerny13]. Consumptive coagulopathy is directly proportional to the amount of tissue injured, and can worsen factor depletion and exaggerate hypofibrinogenemic states [Reference Jensen, Stensballe and Afshari14]. EGC breakdown leads to an increase in cellular expression of glycoproteins, thrombomodulin (TM), and endothelial protein C receptors. With less extensive injury, there is local release of thrombin, which binds to TM. The thrombin–TM complex then binds to, and activates, protein C. In severe trauma, there is a large systemic, rather than local, release of thrombin. This creates more thrombin–TM complexes to accelerate activated protein C (APC) activity, which has multiple pathways to function as an anticoagulant. The rapid rise in APC leads to coagulopathies by inactivating factors Va and VIIa, which aid in clot formation [Reference Chignalia, Yetimakman and Christiaans3, Reference Brohi, Cohen, Ganter, Matthay, Mackersie and Pittet15, Reference Davenport, Guerreiro and Frith16].
Severe trauma leads to a hyperfibrinolytic state due to initial upregulation of plasmin through thrombin release, as well as tissue ischemia. This state results in an increased release of tissue plasminogen activator. APC additionally inactivates plasminogen activator inhibitor-1, leading to an overall increase in plasmin and subsequent fibrinolysis [Reference Chignalia, Yetimakman and Christiaans3, Reference Davenport, Guerreiro and Frith16]. Trauma patients with elevated APC levels have been found to have higher fibrinolytic activity, lower fibrinogen levels, higher transfusion rates, longer hospitalizations, and higher mortality [Reference Davenport and Brohi10, Reference Davenport, Guerreiro and Frith16]. An early treatment of hyperfibrinolysis is use of tranexamic acid (TXA), a lysine analog that functions as an antifibrinolytic in patients who are bleeding, preventing clot breakdown by inhibiting plasmin. The CRASH-2 trial found no evidence of increased thromboembolic events with the use of antifibrinolytics in trauma and a decrease in blood loss with a reduced need for blood product transfusions [Reference Roberts, Shakur and Coats17]. The recommended dosing for TXA in the trauma patient varies from a fixed dose of 1 g every 8 hours. Other guidelines promote weight-based doses ranging from 2.5 to 100 mg kg−1, with varying administration periods ranging from 1 to 12 hours [Reference Thomas and Makris2, Reference Roberts, Shakur and Coats17]. Two other drugs that function in this class of antifibrinolytics are aminocaproic acid and aprotinin [Reference Roberts, Shakur and Coats17].
Another source of coagulopathy is “auto-heparinization” that occurs in severe traumatic injuries. Damaged vasculature leads to shedding and release of endogenous heparin sulfates that make up the EGC [Reference Moore, Moore, Chapman, Gonzalez and Sauaia11–Reference Astapenko, Benes, Pouska, Lehmann, Islam and Cerny13]. “Auto-heparinization” has been documented in TEG and ROTEM studies seen with prolonged reaction time (R-time) or clotting time (CT).
The combination of hemodilution, hypothermia, and acidosis are referred to as the trauma triad. Acidosis, defined as a pH of <7.2, hypothermia with temperatures lower than 36°C, and hemodilution from prehospital crystalloid administration frequently occur together and contribute to this coagulopathic phenomenon. Mild hypothermia of 34–36°C typically does not result in clinically significant coagulation derangements. However, when a patient’s temperature is lower than 32–34°C, thrombin generation, fibrinogen production, and platelet function are reduced and bleeding risk becomes clinically significant [Reference Simmons, Pittet and Pierce9, Reference Jensen, Stensballe and Afshari14, Reference Watts, Trask, Soeken, Perdue, Dols and Kaufmann18]. Effects of hypothermia may not be evident on viscoelastic testing, as samples are warmed to 37°C. To combat hypothermia, trauma rooms should be warmed, with readily available fluid warmers and forced air warming devices. Correction of these metabolic or respiratory derangements is necessary for coagulation enzymes to function properly, using ventilator adjustments on intubated patients, as well as administration of medications containing bicarbonate to raise the pH. Crystalloid resuscitation-induced coagulopathy occurs due to further injury and breakdown of the EGC, existing clots, hemodilution, and immunomodulation [Reference Simmons, Pittet and Pierce9]. Dilutional coagulopathy is inversely proportional to clot strength, factor availability, and time to initiation of clot formation [Reference Jensen, Stensballe and Afshari14]. Balanced crystalloid solutions are recommended because large volumes of normal saline create hyperchloremic metabolic acidosis [Reference Simmons, Pittet and Pierce9].
Hypercoagulability of Trauma
After an initial TIC, patients are at risk of becoming hypercoagulable and are at higher risk of thromboembolic events. Up to 80% of trauma patients may develop deep vein thrombosis (DVT), and up to 20% may form pulmonary embolism. Some may be hypercoagulable on admission, which can be detected with a short TEG R-time, elevated TEG maximum amplitude (MA), and alpha angle. Contributing factors include endothelial injury, upregulation of inflammatory factors, increased platelet and factor activation, venous stasis, and a shift to a hypofibrinolytic state after the initial trauma [Reference Gonzalez, Moore and Moore19]. Risk increases as the ISS increases, as well as with head injuries, pelvic and lower extremity fractures, age, longer surgical procedures, and prolonged immobilization [Reference Toker, Hak and Morgan20, Reference Ruskin21]. Patients are at an increased risk of thromboembolic events as long as 3 months after the initial traumatic injury [Reference Ruskin21].
Pharmacologic prophylaxis is the most effective preventative measure against thromboembolic events in trauma patients. Unless there is a contraindication, low-molecular weight heparin is currently recommended by the Eastern Trauma Association for DVT prophylaxis because it is associated with the lowest mortality from thromboembolic events [Reference Ruskin21]. Unfractionated heparin and fondaparinux, a factor Xa inhibitor, have also been proved for use in DVT prophylaxis in trauma patients. Mechanical prophylaxis with graduated compression stockings, pneumatic compression stockings, and vena cava filters can be used in conjunction with pharmacologic prophylaxis or as a substitute in cases where patients cannot receive pharmacologic prophylaxis [Reference Ruskin21].
Anticoagulation
Anticoagulants are prescribed for patients with thrombotic diseases, mechanical heart valves, and stent placement, and for treatment of acute or chronic venous thrombosis and stroke prevention in patients with atrial fibrillation. If the patient also suffers traumatic injuries, these medications lead to increased blood loss. In addition to evaluating injuries, it is essential to obtain the patient’s medical history, identify the disease process necessitating anticoagulation, and determine the timing of their last medication dose to appropriately weigh the risks and benefits of anticoagulation reversal [Reference Thomas and Makris2].
The varying pharmacokinetics of each anticoagulant, in combination with the patient’s bleeding risk and medical comorbidities, determine whether a reversal agent or a specific blood product is required. For example, in a patient with a recent cardiac stent taking dual antiplatelet medications, platelet administration may increase the risk of stent thrombosis. If their bleeding risk from trauma is low, reversal is likely not indicated; however, it may be lifesaving if they suffer significant traumatic injuries or head injuries. In addition to antiplatelet medications blocking cyclooxygenase 1 and 2 and preventing platelet aggregation, factor inhibition can range from vitamin K antagonists (VKAs) to direct oral anticoagulants (DOACs). These medications impede specific components of the coagulation cascade, such as direct thrombin or factor Xa inhibitors. Warfarin is a commonly used VKA that was prescribed for prophylaxis against stroke in 2010 to over 30 million patients with atrial fibrillation [Reference Yee and Kaide5]. DOACs are now more commonly used than VKAs due to reduced risk of life-threatening bleeds and ease of perioperative management [Reference Cuker, Burnett and Triller22].
Patients taking these medications will have abnormal diagnostic labs. A prolonged aPTT is indicative of drugs or other coagulopathies affecting factors of the intrinsic pathway. Factor II inhibitors, such as dabigatran, can also prolong aPTT times [Reference Yee and Kaide5]. The extrinsic pathway, measured with PT and INR, is affected by warfarin and factor Xa inhibitors, such as rivaroxaban. Drug-specific assays, such as antifactor Xa, cannot be obtained within the time needed to treat a trauma patient during primary resuscitation [Reference Yee and Kaide5, Reference Cuker, Burnett and Triller22].
TEG and ROTEM
TEG (TEG® analyzer 5000 and TEG® 6s, Haemonetics Corporation, Boston, MA) and ROTEM (ROTEM® analyzer systems, Instrumentation Laboratory, Bedford, MA) are point-of-care whole blood functional assay tests of clot formation that guide goal-directed blood product and factor replacement. TEG and ROTEM provide rapid results and have been proven effective at identifying trauma-related coagulopathies [Reference Baksaas-Aasen, Van Dieren and Balvers23]. Both tests measure phases of clot formation and degradation, producing electric signals that are transformed into the graph shown in Figure 31.1.

Figure 31.1 Schematic thromboelastography (TEG, upper part)/rotational thromboelastometry (ROTEM, lower part) trace, indicating commonly reported variables: reaction time (R)/clotting time (CT), clot formation time (K, CFT), alpha angle (α), maximum amplitude (MA)/maximum clot firmness (MCF), and lysis (Ly)/clot lysis (CL). Source: Reprinted under CC BY 2.0 (https://creativecommons.org/licenses/by/2.0/) from Johansson PI, Stissing T, Bochsen L, et al. Thrombelastography and tromboelastometry in assessing coagulopathy in trauma. Scand J Trauma Resusc Emerg Med. 2009;17:45.
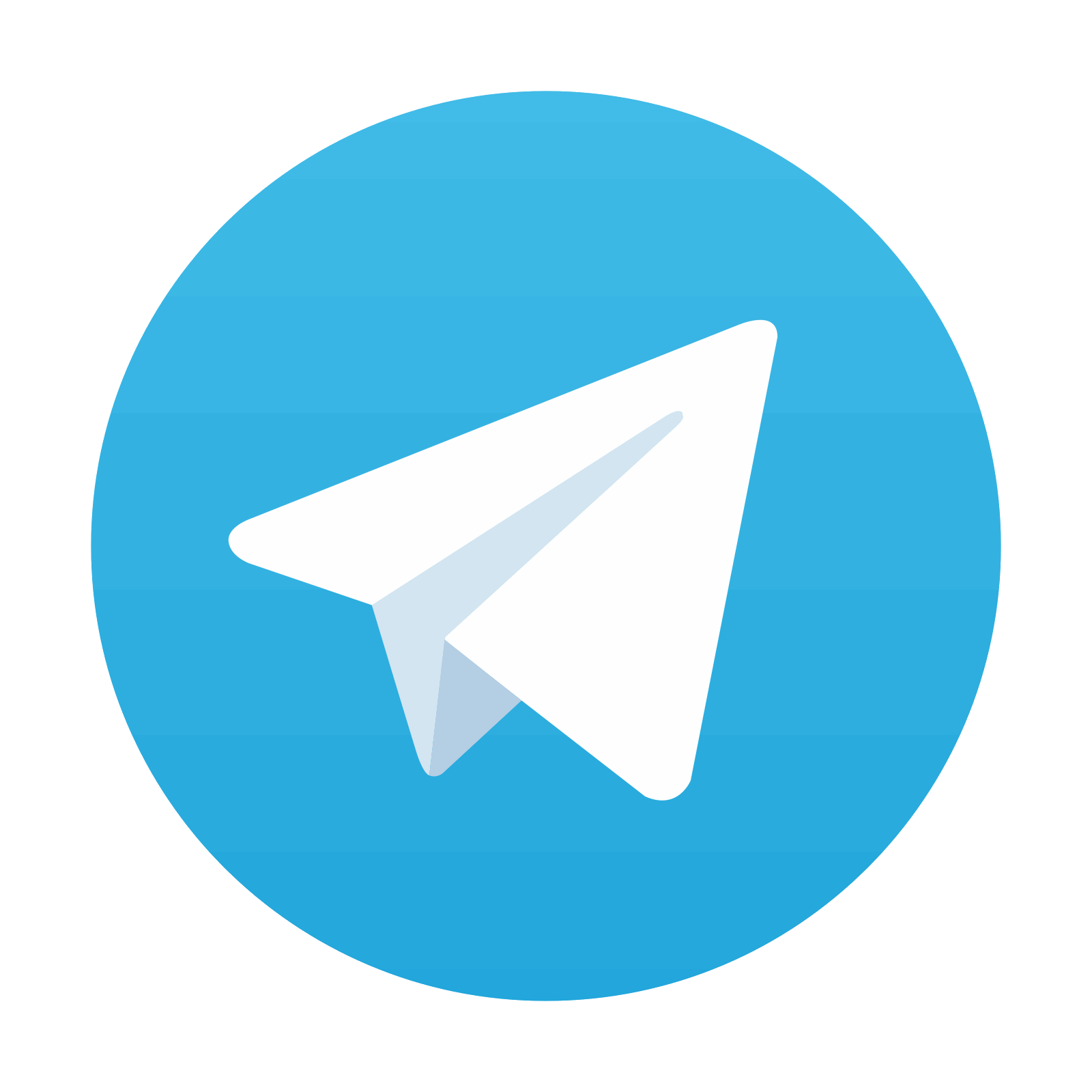
Stay updated, free articles. Join our Telegram channel

Full access? Get Clinical Tree
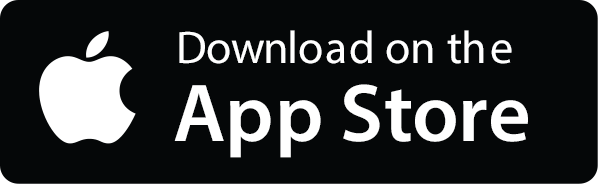
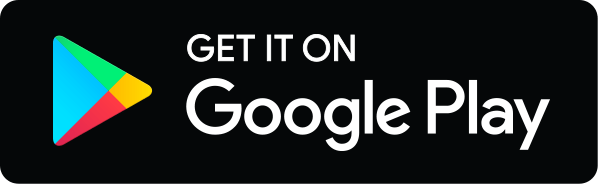
